Bioenergetics II 2025 Student PDF
Document Details
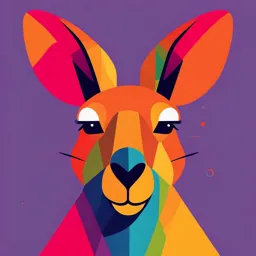
Uploaded by ProfoundFuchsia6830
George Washington University
2025
Donald Murray
Tags
Summary
These notes cover bioenergetics, focusing on metabolic pathways like substrate and oxidative phosphorylation, slow glycolysis, the Krebs cycle, and the electron transport chain. The document also details the utilization of lipids during exercise, and the energy yield calculations involved in these processes.
Full Transcript
Bioenergetics II D O N A L M U R RAY , P H D , C S C S PT 8202 Metabolic pathways occur at two levels Substrate level Phosphorylation anaerobic metabolism immediate energy systems glycolytic system (anaerobic or fast glycolysis) Oxidative level Phosphorylation aerobic metabolism oxida...
Bioenergetics II D O N A L M U R RAY , P H D , C S C S PT 8202 Metabolic pathways occur at two levels Substrate level Phosphorylation anaerobic metabolism immediate energy systems glycolytic system (anaerobic or fast glycolysis) Oxidative level Phosphorylation aerobic metabolism oxidation of glucose beta oxidation protein catabolism and gluconeogenesis The goal of all of these energy systems is to replenish ATP to provide energy for cellular processes Slow Glycolysis Aerobic (oxidative) Glycolysis Utilization of oxygen to produce ATP Occurs in mitochondria of cell Kreb’s Cycle, tricarboxylic (TCA), citric acid cycle Electron Transport Chain Utilized in activities of greater than 2 min in duration Aerobic ATP Production Krebs cycle (citric acid cycle, TCA) Completes the oxidation of substrates and produces NADH and FADH to enter the electron transport chain Electron transport chain Oxidative phosphorylation Electrons oxidized from NADH and FADH are passed along a series of carriers to produce ATP H+ from NADH and FADH are accepted by O2 to form water Mitochondria respiration During rest and steady state activity, most of the pyruvate is not converted to lactate but is instead taken up by the mitochondria further catabolized to produce ATP and CO2 Krebs cycle (also referred to as TCA or CAC) is carried out in the matrix of the mitochondria Respiratory Chain/Electron Transport Chain (ETC) is located on the mitochondrial inner membrane Mitochondrial functions Outer membrane – barrier to maintain important internal constituents (i.e. NADH) & to exclude exterior factors Contains specific transport mechanisms to regulate influx and efflux of various materials Intermembrane space – contains enzymes for exchange and transport Inner membrane a) Functions w/ outer and inner membrane in transport capacity b) Referred to as cristae membrane b/c of its many folds, main site for oxidative phosphorylation Molecular (F) complexes – actual site of phosphorylation Matrix – contains nearly half of mitochondrial proteins Where LDH & Krebs cycle enzymes are located Oxidation Biologic burning of macronutrients provides the energy required for phosphorylation Occurs on inner membrane of mitochondria Involves transferring electrons from NADH and FADH2 to molecular O2, which then releases and transfers chemical energy to combine ATP from ADP plus a phosphate ion During aerobic ATP resynthesis, O2 combines with hydrogen to form H2O 90% of ATP synthesis takes place in the respiratory chain by oxidative reactions coupled with phosphorylation Prerequisites of OXPHOS Continual ATP resynthesis during coupled oxidative phosphorylation of macronutrients has three prerequisites: 1. Availability of reducing agents NADH or FADH2 2. Presence of a terminal oxidizing agent as oxygen 3. Sufficient quantity of enzymes and metabolic machinery in tissues to make energy transfer reactions “go” at appropriate sequence and rate Pyruvate entry into mitochondria Both lactate and pyruvate are a) released into the blood and b) gain entry into the mitochondrion by Monocarboxylate Transport Proteins (MCT) Pyruvate Dehydrogenase catalyzes RX Pyruvate Acetyl-COA Committed Step Additional products CO2 PDH NADH Pathway for pyruvate entry into TCA Plays a major role in determining the rate of lactate formation and substrate availability for mitochondrial oxidation PDH reaction Converting of pyruvate to Acetyl-CoA Pyruvate loses a carbon – blown off as CO2 Total yield from one glucose Two pyruvates Two Acetyl-CoA – Enters the Krebs or TCA Two CO2 – blown off through the respiratory system Two NADH – enters the electron transport chain Acetyl-CoA is very important as it is entry point of all the major nutrients into the TCA – carbs, fats and protein) Controlled by Phosphorylation State – Inhibited by phosphorylation – Activated by dephosphorlyation Activation Inhibition Low ATP/ADP High ATP/ADP Low Acet-CoA High Acet-CoA Low NADH/NAD High NADH TCA Cycle 9 reactions that results in Decarboxylation of acetyl-CoA Production of NADH & FADH Molecules can enter and leave the cycle at any stage Carbon added then taken away Oxaloacetate only has 4 carbons which are added to 2 carbon Acetyl-CoA to start process again Summary of TCA regulators PDH regulated by ATP/ADP, Acet-CoA/CoA, NADH/NAD Citrate synthase regulated by ATP/ADP conditions of adequate ATP such as rest decrease conversion of acetyl-CoA to citrate Dehydrogenase enzymes Regulated by ATP/ADP and NADH/NAD Under enzyme control Under adequate ATP and NADH or near resting conditions control enzymes are inhibited and decrease TCA function When ATP and NADH are decreased, enzymes are stimulated to increase function of the TCA TCA Products ATP x 1 NADH x 4 3 from TCA 1 from Acet-CoA FADH x 1 CO2 x 2 1 from Acet-CoA 1 step-4 Net Energy Transfer from Glucose Catabolism Electron Transport Chain ETC Consists of 4 complexes located on the mitochondria inner membrane Series of reactions that oxidize NADH and FADH to NAD & FAD End product is water Main purpose is to phosphorylate ADP to ATP So basically uses electrons and protons from FADH and NADH to generate ATP + water This process is called oxidative phosphorylation Reduction potential Measure of affinity for electrons by the electron transport chain Electrons move along the chain because the reduction potential progressively increases along the chain Each successive complex has an increasing affinity for electrons Oxygen in particular has a very high affinity for electrons The presence of oxygen inside the mitochondria drives all of the mitochondria reactions NADH donates electrons and protons at the beginning of the chain FADH donates electrons and protons further along the chain ETC https://www.youtube.com/watch?v=xbJ0nbzt5Kw ETC control ATP Synthase Protein complex found on inner membrane Involved in phosphorylation of ADP to ATP The synthesis of one ATP molecule is thought to require use of four protons in the ATP synthase protein The synthesis of ATP is brought about by the rotary motion of the complex's on this enzyme: when a large electrochemical potential (proton gradient) flows through the enzyme subunit, this causes rotation of the all subunits leading to ATP synthesis. ETC control No one single substrate identified as the main controller ATP production rate, pyruvate, ADP, Pi, NADH, FADH, and oxygen have been identified as controllers Although even a small drop in ADP levels though mean pathway is activated The concentration of cytochrome-C oxidase and oxygen availability are two of the main regulators during exercise Energy yield per molecule of glucose Each glucose unit = 2 ATRP and 2 NADH in cytoplasm Thus glycolysis yields 6 ATP in mitochondria in addition to 2 cytoplasmic ATP Pyruvate-to-Acetyl-CoA = 6 ATP (from the NADH produced) TCA = 24 ATP Total energy yield per molecule of glucose = 38 ATP What is the energy yield of glycogen? 3 ATP in cytoplasm (39 ATP) Oxidation of Carbohydrate Stage 1: Glycolysis Stage 2: Krebs cycle Stage 3: Electron transport chain Oxidation of Carbohydrate Beta-oxidation Energy Release from Lipids Stored fat: most plentiful source of potential energy Three specific energy sources for fat catabolism: 1.Triacylglycerols stored directly within a muscle fiber in close proximity to mitochondria 2.Circulating triacylglycerols in lipoprotein complexes become hydrolyzed on surface of a tissue’s capillary endothelium 3.Adipose tissue provides circulating free fatty acids (FFA’s) mobilized from triacylglycerols in adipose tissue FFA transferred across mitochondrial membrane by carnitine shuttle this carrier may be the regulator Breakdown of triacylglycerol molecule yields about 460 ATP molecules Lipid metabolism Lipolysis is the breakdown of a triglyceride to three fatty acids and glycerol Lipase is the main enzyme involved Lipase is hormone sensitive Activated by epinephrine, glucagon & growth hormones Inhibited by an increase in insulin levels These products can then enter metabolism Despite large fuel stores of lipid, metabolism is very slow Much slower than carbs Yet a small increase in the utilization of lipids/fats as fuel could help negate muscle glycogen and blood glucose loss Fat metabolism decreases with dependence on glucose metabolism such as in exercise Lipid metabolism Lipolysis – breakdown of fats to create FFA Happens in adipose storage sites throughout the body Skeletal muscle contains lipid droplets called intramuscular triglycerides (IMTG) Lower intensity exercise adipose tissue release is major site of lipolysis Higher intensity between 65% -80% VO2 max IMTG (Intramuscular Triglycerides major site of lipolysis Lipid metabolism Fatty acids are insoluble meaning they need a carrier protein in the blood – albumin Uptake into the muscle dependent on two transport proteins Fatty acid translocase (FAT/CD36) Fatty acid binding protein(FABP) Increase in both of these associated with weight loss Once in cell cytosol coenzyme A is added to form a fatty acyl-CoA Occurs in outer membrane of mitochondria Enzyme = acyl-CoA synthase Requires energy from two ATP The fatty acyl-CoA is now ready for beta oxidation Beta oxidation Breakdown of the fatty acyl-CoA to actyl-CoA, NADH & FADH Consists of 4 reactions that cleave 2 carbons from the fatty acyl-CoA with each pass through, generating 1 acetyl-CoA 1 NADH 1FADH Fatty acids are very large with as much as 16 carbons, meaning a large haul Utilization of lipids during exercise 1. Mobilization – breakdown of adipose & intramuscular triglyceride 2. Circulation – transport of FFA from adipose to muscle 3. Uptake – entry of FFA into muscle from blood 4. Activation – raising energy level of FFA 5. Translocation – entry of activated FFA into mito 6. Beta-oxidation – production of acetyl- CoA, NADH, and FADH 7. Mitochondrial oxidation – TCA and ETC activity Total Energy Transfer from Fat Catabolism Each triacylglycerol molecule contains 3 fatty acid molecules to form 441 ATP molecules from fatty acid components (3 x 147 ATP) 19 ATP molecules form during glycerol breakdown to generate 460 ATP molecules for each triacylglycerol molecule catabolized Considerable energy yield compared to net ATP formed from glucose Efficiency of energy conservation for fatty acid oxidation amounts to 40%, a value slightly higher than glucose oxidation Summary of Substrate Metabolism Characteristics of the Energy Systems Energy Oxygen? Chemical Relative ATP per Capacity System Reaction rate of molecule ATP/sec ATP-PCr No PCr to Cr 10 1 100 Days (fat) CO2 and H 2O Protein metabolism Provides minimal energy during exercise under normal conditions of proper nutrient storage Inadequate caloric intake or prolonged exercise protein can be broken down to glucose and used to generate ATP Gluconeogenesis – done in liver Amino acid breakdown from stored pools of amino acid contributes to the synthesis of TCA cycle intermediates Ketoglutaric acid is part of the TCA Protein catabolism Amino acids cleaved from the protein strand Amino groups split off - called deamination Carbon skeleton incorporated into catabolic pathways for glucose and lipid Protein catabolism is generally undesirable Applications & Considerations of Bioenergetics Intro Previous classes we talked about resting situations Now we will discuss how metabolism shifts depending on the situation the ‘system’ is in Short bouts of intense exercise Steady state exercise Maximal exercise intensities When we begin exercise many factors influence metabolism Fiber type Type of training Intensity or duration of activity Remember goal of exercise metabolism is to meet ATP demand! Skeletal muscle carbohydrate metabolism Exercise can increase whole body energy metabolism by as much as 20 times above basal levels Depending on the length of the exercise endogenous CHO may be depleted Fatigue or decrement in performance greatly linked to glycogen stores and/or hypoglycemia Hyper – high levels Hypo – low levels Rest Both fat and carbs used as fuel source Heavily influence by what you eat – eat more carbs that’s what is favored Acetyl-CoA is the key regulator – entry point of fatty acid & glucose Generally fatty acid oxidation will be the key fuel source Acetyl-CoA from fatty acid inhibits the PDH reaction Increase in citrate (from TCA) inhibits PFK Once exercise starts this is changed Exercise intensity Both absolute and relative intensity of exercise plays an important role in fuel metabolism Absolute work rate – total quantity of fuel required by working muscles Relative– dictates the proportion of CHO and FAT used Substrate Metabolism During Exercise Short bouts of intense exercise Immediate source is supplied through ATP-PCr system Activated due to increase in ADP – ATP hydrolysis Capacity is small maybe 5-15 seconds An increase in AMP and ADP upregulates PFK and PK In about 6s of max intensity exercise = 15mmol of ATP/s With ~50% supplied by PCr Rest nonaerobic glycolytic sources Short bouts of intense exercise Nonaerobic contribution to energy production is roughly 90% in events lasting less than 20s ~60-70% of energy supplied by nonaerobic metabolism during 30s of all out cycling As duration increases contribution declines Anything over 1 minute majority of energy supplied through aerobic means Submaximal Exercise intensity Goal during submaximal is to reach steady state ATP supply being met through aerobic means Usually takes a period of time for the system to ‘catch up’ TCA enzyme activity accelerates as there is a slight NADH/NAD and ATP/ADP ratios Epinephrine as sympathetic systems ramps up Activates lipase and lipolysis Submaximal exercise intensity At about 50-80% of VO2 max oxidative phosphorylation is the major source of ATP supply Fatty acid oxidations accounts for about 50% of this energy production below ~60% VO2 As intensity increases muscle glycogen becomes major contributor Submaximal exercise intensity During steady state glucose/glycogen supplying ATP aerobically – slow glycolysis If contracting muscle has enough ATP from FFA, Acetly-CoA and NADH levels are maintained then PDH will not be highly active However if a slight decline in acetyl-CoA, ATP/ADP and NADH/NAD occurs then PDH activity will be amped up Enzymes of metabolism are constantly active just depends on how intense the muscle activity is Submaximal Exercise intensity Oxygen uptake increases rapidly Reaches steady state within 1-4 minutes Under constant work demands Oxygen deficit Lag in oxygen uptake at the beginning of exercise Suggests anaerobic pathways contribute to total ATP production After steady state is reached, ATP requirement is met through aerobic ATP production VO2 on-kinetics in SCI INCOMPLETE SCI CONTROL GOLLIE ET AL 2017 Maximal intensity Exercise Low-intensity exercise (70% VO2max) CHO are primary fuel “Crossover” concept Describes the shift from fat to CHO metabolism as exercise intensity increases Due to: Recruitment of fast muscle fibers Increasing blood levels of epinephrine Illustration of the “Crossover” Concept Maximal exercise intensity ATP turnover has greatly increased NADH/NAD and ATP/ADP ratios increase AMP increases Which activates the PFK and PK reactions More glycolysis More ADP and NAD along with increasing levels of pyruvate cause PDH reaction to be ramped up Increase in plasma epinephrine releases stored muscle glycogen glycogenolysis Lipolysis during Max exercise Fatty acid oxidation rates decline at 85% of VO2 max Between 65-85% rate o lipolysis does not change too much But blood flow to/through adipose tissue maybe reduced Meaning less fatty acid movement into bloodstream to be used for metabolism Lipolysis and use of fatty acid remains very high but delivery to the contracting muscle might be reduced Priorities! Estimation of Fuel Utilization During Exercise Respiratory exchange ratio (RER or R) VCO2 / VO2 Fat (palmitic acid) = C16H32O2 C16H32O2 + 23O2 16CO2 + 16H2O + ATP R = VCO2/VO2 = 16 CO2 / 23O2 = 0.70 Glucose = C6H12O6 C6H12O6 + 6O2 6CO2 + 6H2O + ATP R = VCO2/VO2 = 6 CO2 / 6O2 = 1.00 Estimation of Fuel Utilization During Exercise Indicates fuel utilization RER % Fat %CHO 0.70 = 100% fat 0.70 100 0 0.85 = 50% fat, 50% 0.75 83 17 CHO 1.00 = 100% CHO 0.80 67 33 0.85 50 50 During steady-state 0.90 33 67 exercise VCO2 and VO2 reflective 0.95 17 83 of O2 consumption and 1.00 0 100 CO2 production at the cellular level Shift From CHO to Fat Metabolism During Prolonged Exercise Sources of Fuel During Exercise Carbohydrate Blood glucose Muscle glycogen Fat Plasma FFA (from adipose tissue lipolysis) Intramuscular triglycerides Protein Only a small contribution to total energy production (only ~2%) May increase to 5-15% late in prolonged exercise Blood lactate Gluconeogenesis via the Cori cycle Effect of Exercise Duration on Muscle Fuel Source Glycogen depletion Availability of CHO as substrate for ATP production is finite After roughly 90-120 minutes depending on the intensity of the activity Leads to lack of pyruvate production, and in general ATP production Reduces performance levels Ingestion of CHO prior to event may help with this More on this to come…… Quiz opens Tuesday at noon and closes Sunday (2/9) at 11:59pm Friday – Nutrition overview Next week….supplementary systems