Biochemistry and the Organization of Cells PDF
Document Details
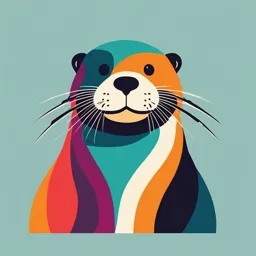
Uploaded by OrderlySchrodinger
Eastern Samar State University
Tags
Summary
This document discusses the organization of cells, and the fundamental processes of biochemistry in detail. It lays the foundation for further study in chemistry and biology.
Full Transcript
1...
1 ©Victor Habbick Visions/Corbis Biochemistry and the Organization of Cells © Biochemistry unlocks the mysteries of the human body. 1.1 Basic Themes Chapter Outline How does biochemistry describe life processes? 1.1 Basic Themes How does biochemistry describe life processes? Living organisms, such as humans, and even the individual cells of which they How did living things originate? are composed, are enormously complex and diverse. Nevertheless, certain uni- 1.2 Chemical Foundations of Biochemistry fying features are common to all living things from the simplest bacterium to Can a chemist make the molecules of life in a the human being. They all use the same types of biomolecules, and they all use laboratory? energy. As a result, organisms can be studied via the methods of chemistry and What makes biomolecules special? physics. The belief in “vital forces” (forces thought to exist only in living organ- 1.3 The Beginnings of Biology: Origin of Life isms) held by 19th-century biologists has long since given way to awareness of How and when did the Earth come to be? an underlying unity throughout the natural world. How were biomolecules likely to have formed on the early Earth? Disciplines that appear to be unrelated to biochemistry can provide answers Which came first—the catalysts or the to important biochemical questions. For example, the MRI (magnetic reso- hereditary molecules? nance imaging) tests that play an important role in the health sciences orig- 1.4 The Biggest Biological Distinction— inated with physicists, became a vital tool for chemists, and currently play a Prokaryotes and Eukaryotes large role in biochemical research. The field of biochemistry draws on many What is the difference between a prokaryote disciplines, and its multidisciplinary nature allows it to use results from many and a eukaryote? sciences to answer questions about the molecular nature of life processes. Important 1.5 Prokaryotic Cells applications of this kind of knowledge are made in medically related fields; an How is prokaryotic DNA organized without a understanding of health and disease at the molecular level leads to more effec- nucleus? tive treatment of illnesses of many kinds. 1.6 Eukaryotic Cells The activities within a cell are similar to the transportation system of a city. What are the most important organelles? What are some other important components of The cars, buses, and taxis correspond to the molecules involved in reactions (or cells? series of reactions) within a cell. The routes traveled by vehicles likewise can be compared to the reactions that occur in the life of the cell. Note particularly 1.7 Five Kingdoms, Three Domains How do scientists classify living organisms today? that many vehicles travel more than one route—for instance, cars and taxis Is there a simpler basis for classifying can go almost anywhere—whereas other, more specialized modes of transpor- organisms? tation, such as subways and streetcars, are confined to single paths. Similarly, 1.8 Common Ground for All Cells some molecules play multiple roles, whereas others take part only in specific Did eukaryotes develop from prokaryotes? series of reactions. Also, the routes operate simultaneously, and we shall see that Did symbiosis play a role in the development of this is true of the many reactions within a cell. eukaryotes? To continue the comparison, the transportation system of a large city has 1.9 Biochemical Energetics more kinds of transportation than does a smaller one. Whereas a small city What is the source of energy in life processes? How do we measure energy changes in may have only cars, buses, and taxis, a large city may have all of these plus oth- biochemistry? ers, such as streetcars or subways. Analogously, some reactions are found in all cells, and others are found only in specific kinds of cells. Also, more structural 1.10 Energy and Change What kinds of energy changes take place in features are found in the larger, more complex cells of larger organisms than living cells? in the simpler cells of organisms such as bacteria. 1.11 Spontaneity in Biochemical Reactions An inevitable consequence of this complexity is the large quantity of ter- How can we predict what reactions will happen minology that is needed to describe it; learning considerable new vocabulary in living cells? is an essential part of the study of biochemistry. You will also see many cross- 1.12 Life and Thermodynamics references in this book, which reflect the many connections among the pro- Is life thermodynamically possible? cesses that take place in the cell. Online homework for this chapter may be assigned in OWL. Copyright 2010 Cengage Learning. All Rights Reserved. May not be copied, scanned, or duplicated, in whole or in part. Due to electronic rights, some third party content may be suppressed from the eBook and/or eChapter(s). Editorial review has deemed that any suppressed content does not materially affect the overall learning experience. Cengage Learning reserves the right to remove additional content at any time if subsequent rights restrictions require it. 10 CHAPTER 1 Biochemistry and the Organization of Cells Amino acid Amino acid Polypeptide H R1 H R2 H R1 H C + C C N COO– H+3N COO – H+3N COO – H+3N C C...... H2O O H R2 N C Sense A Amino acids build proteins by connecting the carboxyl group of one amino acid with the amino group of the next amino acid. Sugar Sugar Polysaccharide HO 4 6 HO 4 6 5 CH2OH 5 CH2OH HO................ CH2OH O + O O HO HO 3 3 HO HO 2 HO 2 OH OH HO 1 1 H 2O O 4..... 1 CH2OH O HO 4 1 OH HO Sense HO OH B Polysaccharides are built by linking the first carbon of one sugar with the fourth carbon of the next sugar. Nucleotide Nucleotide Nucleic acid NH2 NH2 NH2 N N N N O O O 5' N O 5' N N 5' N O HO P OCH2 + HO P OCH2 HO P OCH2 O O O O– O– O– NH2........... 4' 1' 4' 1' H2O 3' 2' 3' 2' 3' 2' N N OH OH OH OH O OH.... N N 5' PO4 3' OH O P OCH2 Sense O O– C In nucleic acids the 3'-OH of the ribose ring of one nucleotide 3' forms a bond to the 5'-OH of the ribose ring of a neighboring OH OH nucleotide. All these polymerization reactions are accompanied by the elimination of water. FIGURE 1.6 Directionality in macromolecules. Biological macromolecules and their building blocks have a “sense” or directionality. Molecules to Cells Which came first—the catalysts or the hereditary molecules? A discovery with profound implications for discussions of the origin of life is that RNA (ribonucleic acid), another nucleic acid, is capable of catalyzing its own processing. Until this discovery, catalytic activity was associated exclusively with proteins. RNA, rather than DNA, is now considered by many scientists to have been the original coding material, and it still serves this function in some viruses. The idea that catalysis and coding both occur in one molecule has pro- vided a point of departure for more research on the origins of life. The “RNA Copyright 2010 Cengage Learning. All Rights Reserved. May not be copied, scanned, or duplicated, in whole or in part. Due to electronic rights, some third party content may be suppressed from the eBook and/or eChapter(s). Editorial review has deemed that any suppressed content does not materially affect the overall learning experience. Cengage Learning reserves the right to remove additional content at any time if subsequent rights restrictions require it. 1.3 The Beginnings of Biology: Origin of Life 11 world” is the current conventional wisdom, but many unanswered questions Polynucleotide template exist regarding this point of view. G G A C U C According to the RNA-world theory, the appearance of a form of RNA capable of coding for its own replication was the pivotal point in the origin A G of life. Polynucleotides can direct the formation of molecules whose sequence U G C C is an exact copy of the original. This process depends on a template mecha- Complementary polynucleotides nism (Figure 1.7), which is highly effective in producing exact copies but is a relatively slow process. A catalyst is required, which can be a polynucleotide, Synthesis of a even the original molecule itself. Polypeptides, however, are more efficient cat- complementary H2O polynucleotide alysts than polynucleotides, but there is still the question of whether they can direct the formation of exact copies of themselves. Recall that in present-day cells, the genetic code is based on nucleic acids, and catalysis relies primarily G G A C U C on proteins. How did nucleic acid synthesis (which requires many protein enzymes) and protein synthesis (which requires the genetic code to specify the C C U G A G order of amino acids) come to be? According to this hypothesis, RNA (or a system of related kinds of RNA) originally played both roles, catalyzing and Strands separate encoding its own replication. Eventually, the system evolved to the point of being able to encode the synthesis of more effective catalysts, namely proteins The complementary strand acts as a new (Figure 1.8). Even later, DNA took over as the primary genetic material, relegat- template strand ing the more versatile RNA to an intermediary role in directing the synthesis of proteins under the direction of the genetic code residing in DNA. A certain amount of controversy surrounds this theory, but it has attracted considerable C C U G A G attention recently. Many unanswered questions remain about the role of RNA in the origin of life, but clearly that role must be important. C C U Another key point in the development of living cells is the formation of G A G membranes that separate cells from their environment. The clustering of cod- ing and catalytic molecules in a separate compartment brings molecules into Synthesis of new copies of the closer contact with each other and excludes extraneous material. For reasons original strand H2O we shall explore in detail in Chapters 2 and 8, lipids are perfectly suited to form cell membranes (Figure 1.9). Recently, attempts have been made to combine several lines of reason- C C U G A G ing about the origin of life into a double-origin theory. According to this line of thought, the development of catalysis and the development of a coding system G G A C U C came about separately, and the combination of the two produced life as we know it. The rise of aggregates of molecules capable of catalyzing reactions FIGURE 1.7 The role of templates in synthesis was one origin of life, and the rise of a nucleic acid-based coding system was of polynucleotides. Polynucleotides use a another origin. template mechanism to produce exact copies of themselves: G pairs with C, and A pairs with A theory that life began on clay particles is a form of the double-origin theory. U by a relatively weak interaction. The original According to this point of view, coding arose first, but the coding material strand acts as a template to direct the synthesis was the surface of naturally occurring clay. The pattern of ions on the clay sur- of a complementary strand. The complementary strand then acts as a template for the production face is thought to have served as the code, and the process of crystal growth of copies of the original strand. Note that the is thought to have been responsible for replication. Nucleotides, then RNA original strand can be a template for a number molecules, formed on the clay surface. The RNA molecules thus formed were of complementary strands, each of which in turn can produce a number of copies of the original released from the clay surface and enclosed in lipid sacs, forming protocells. strand. This process gives rise to a many-fold In this scenario, protocells exist in a pond with a warm side and a cold side. amplification of the original sequence. (Copyright Double-stranded polynucleotides are formed on the cold side of the pond on a © 1994 from The Molecular Biology of the Cell, 3rd Edition by A. Alberts, D. Bray, J. Lewis, M. Raff, single-stranded template (Figure 1.10). The protocell moves to the warm side K. Roberts, and J. D. Watson. Reproduced by permission of the pond, where the strands separate. The membrane incorporates more of Garland Science/Taylor & Francis Books, Inc.) lipid molecules. The protocell divides, with a single-stranded RNA in each daughter cell, and the cycle repeats. In the development from protocells to single cells similar to modern bacteria, proteins and then DNA enter the picture. In this scenario, ribozymes (catalytic RNA molecules) develop and direct the duplication of RNA. Other ribozymes catalyze metabolic reactions, eventually giving rise to proteins (Figure 1.11). Eventually, proteins rather than ribozymes catalyze most of the reactions in the cell. Still later, other enzymes catalyze the production of DNA, which takes over the primary role in coding. RNA now serves as an intermediary between DNA Copyright 2010 Cengage Learning. All Rights Reserved. May not be copied, scanned, or duplicated, in whole or in part. Due to electronic rights, some third party content may be suppressed from the eBook and/or eChapter(s). Editorial review has deemed that any suppressed content does not materially affect the overall learning experience. Cengage Learning reserves the right to remove additional content at any time if subsequent rights restrictions require it. 12 CHAPTER 1 Biochemistry and the Organization of Cells 1 3 Catalyst Replication The RNA sequence becomes a template for the sequence of amino acids in the protein by using A catalytic RNA directs its own the adaptor mechanism. replication with the original nucleotide sequence and shape. 2 Coding RNA Adaptor RNA Growing protein More catalytic RNAs evolve. Some One RNA molecule in a group (adaptor RNAs) bind to amino acids. catalyzes the synthesis of all The adaptor RNAs also engage in RNAs in the group. complementary pairing with coding RNA. FIGURE 1.8 Stages in the evolution of a system of self-replicating RNA molecules. At each stage, more complexity appears in the group of RNAs, leading eventually to the synthesis of proteins as more effective catalysts. (Copyright © 1994 from The Molecular Biology of the Cell, 3rd Edition by A. Alberts, D. Bray, J. Lewis, M. Raff, K. Roberts, and J. D. Watson. Reproduced by permission of Garland Science/Taylor & Francis Books, Inc.) Without compartments With compartmentalization by cell membrane en co de s Protein catalyzes reactions for all RNA The protein made by the cell’s RNA is retained for use in the Self-replicating RNA cell. The RNA can be selected molecules, one of which on the basis of its use of a more can direct protein synthesis effective catalyst. FIGURE 1.9 The vital importance of a cell membrane in the origin of life. Without compartments, groups of RNA molecules must compete with others in their environment for the proteins they synthesize. With compartments, the RNAs have exclusive access to the more effective catalysts and are closer to each other, making it easier for reactions to take place. (Copyright © 1994 from The Molecular Biology of the Cell, 3rd Edition by A. Alberts, D. Bray, J. Lewis, M. Raff, K. Roberts, and J. D. Watson. Reproduced by permission of Garland Science/Taylor & Francis Books, Inc.) Copyright 2010 Cengage Learning. All Rights Reserved. May not be copied, scanned, or duplicated, in whole or in part. Due to electronic rights, some third party content may be suppressed from the eBook and/or eChapter(s). Editorial review has deemed that any suppressed content does not materially affect the overall learning experience. Cengage Learning reserves the right to remove additional content at any time if subsequent rights restrictions require it. 1.4 The Biggest Biological Distinction—Prokaryotes and Eukaryotes 13 Cold side of pond Warm side of pond ASSISTED REPRODUCTION 4 Membrane Once released from clay, the newly incorporates new 5 Protocell fatty molecules formed polymers might become divides, and and grows engulfed in water-filled sacs as fatty daughter cells acids spontaneously arranged D themselves into membranes. These repeat the cycle co irec nv ti protocells probably required some ec ontion external prodding to begin duplicating of their genetic material and thus reproducing. In one possible scenario (right), the protocells circulated between Daughter the cold and warm sides of a pond, which cells may have been partially frozen on one side (the early earth was mostly cold) and thawed Fatty on the other side by the heat of a volcano. molecules On the cold side, single RNA strands 1 acted as templates on which new 1 Nucleotides Nucleotides nucleotides formed base pairs (with As enter and pairing with Us and Cs with Gs), resulting form comple- in double strands 2. On the hot side, mentary 3 Heat separates heat would break the double strands strand the strands apart 3. Membranes could also slowly grow 4 until the protocells divided into “daughter” protocells 5 , RNA which could then start the cycle again. double Once reproduction cycles got going, strand 2 Protocell evolution kicked in—driven by random mutations—and at some point the reaches protocells gained the ability to “maturity” reproduce on their own. Life was born. FIGURE 1.10 Replication and reproduction. When RNA polymers first formed, they could replicate on the cold side of a pond, producing double-stranded RNA. The strands separate on the warm side and divide into new protocells. (Reprinted with permission. Copyright © 2009 Scientific American, a division of Nature America, Inc. All rights reserved.) and proteins. This scenario assumes that time is not a limiting factor in the pro- cess. In an attempt to study the origins of life, scientists have also attempted to combine the best properties of proteins and nucleic acids and have created Peptide Nucleic Acids, PNA. Evidence shows that the building blocks of these hybrids could also have formed in the primordial world, and some theorize that PNA may have been the original molecule that allowed life to form. Currently scientists are attempting to create artificial living cells based on PNA. The goal is to demonstrate that under the conditions of the “primordial soup” simple mole- cules could form complex molecules possessing the critical functions of catalysis and replication, and that these could then form cells capable of dividing. At this writing, none of the theories of the origin of life is definitely estab- lished, and none is definitely disproved. The topic is still under active investi- gation. It seems highly unlikely that we will ever know with certainty how life originated on this planet, but these conjectures allow us to ask some of the im- portant questions, such as those about catalysis and coding, that we are going to see many times in this text. 1.4 The Biggest Biological Distinction— Prokaryotes and Eukaryotes All cells contain DNA. The total DNA of a cell is called the genome. Individual units of heredity, controlling individual traits by coding for a functional pro- tein or RNA, are genes. Copyright 2010 Cengage Learning. All Rights Reserved. May not be copied, scanned, or duplicated, in whole or in part. Due to electronic rights, some third party content may be suppressed from the eBook and/or eChapter(s). Editorial review has deemed that any suppressed content does not materially affect the overall learning experience. Cengage Learning reserves the right to remove additional content at any time if subsequent rights restrictions require it. 14 CHAPTER 1 Biochemistry and the Organization of Cells 2 RNA CATALYSTS Ribozymes—folded RNA mole- Journey to the cules analogous to protein-based Modern Cell enzymes—arise and take on such Waste jobs as speeding up reproduction Energy Double- Lipid and strengthening the protocell’s stranded membrane membrane. Consequently, RNA After life got started, protocells begin to reproduce competition among on their own. Ribozyme Nu life-forms fueled the tri drive toward ever more ents complex organisms. We may never know the exact RNA is details of early evolution, duplicated but here is a plausible New sequence of some of the strand 1 EVOLUTION STARTS major events that led 3 METABOLISM BEGINS from the first protocell The first protocell is just a Ribozyme Other ribozymes catalyze to DNA-based cells such sac of water and RNA and metabolism—chains of chemical as bacteria. requires an external stimulus reactions that enable protocells (such as cycles of heat and to tap into nutrients from the cold) to reproduce. But it environment. will soon acquire new traits. A 5 PROTEINS TAKE OVER Amino Proteins take on a wide range of acid chain tasks within the cell. Protein- DNA based catalysts, or enzymes, gradually replace most Ribosome ribozymes. Ribozymes Enzymes Folded protein Folded Waste Energy protein 4 PROTEINS APPEAR N 6 THE BIRTH OF DNA Complex systems of RNA Enzyme Other enzymes begin to make 7 BACTERIAL WORLD ut catalysts begin to translate rie DNA. Thanks to its superior Organisms resembling modern strings of RNA letters nts stability, DNA takes on the role bacteria adapt to living virtually (genes) into chains of amino of primary genetic molecule. everywhere on earth and rule acids (proteins). Proteins RNA’s main role is now to act unopposed for billions of years, later prove to be more as a bridge between DNA and until some of them begin to evolve efficient catalysts and able proteins. into more complex organisms. to carry out a variety of tasks. B FIGURE 1.11 From membrane-coated RNA to bacteria. (A) Ribozymes start to catalyze a number of reactions, giving rise to metabolism. (B) Proteins eventually take over most of catalysis. DNA becomes the primary coding molecule. (Reprinted with permission. Copyright © 2009 Scientific American, a division of Nature America, Inc. All rights reserved.) The earliest cells that evolved must have been very simple, having the mini- mum apparatus necessary for life processes. The types of organisms living to- day that probably most resemble the earliest cells are the prokaryotes. This word, of Greek derivation (karyon, “kernel, nut”), literally means “before the nucleus.” Prokaryotes include bacteria and cyanobacteria. (Cyanobacteria were formerly called blue-green algae; as the newer name indicates, they are more closely related to bacteria.) Prokaryotes are single-celled organisms, but groups of them can exist in association, forming colonies with some differentiation of cellular functions. Copyright 2010 Cengage Learning. All Rights Reserved. May not be copied, scanned, or duplicated, in whole or in part. Due to electronic rights, some third party content may be suppressed from the eBook and/or eChapter(s). Editorial review has deemed that any suppressed content does not materially affect the overall learning experience. Cengage Learning reserves the right to remove additional content at any time if subsequent rights restrictions require it. 1.4 The Biggest Biological Distinction—Prokaryotes and Eukaryotes 15 What is the difference between a prokaryote and a eukaryote? The word eukaryote means “true nucleus.” Eukaryotes are more complex organ- isms and can be multicellular or single-celled. A well-defined nucleus, set off from the rest of the cell by a membrane, is one of the chief features distinguish- ing a eukaryote from a prokaryote. A growing body of fossil evidence indicates that eukaryotes evolved from prokaryotes about 1.5 billion (1.5 × 109) years ago, about 2 billion years after life first appeared on Earth. Examples of single-celled eukaryotes include yeasts and Paramecium (an organism frequently discussed in beginning biology courses); all multicellular organisms (e.g., animals and plants) are eukaryotes. As might be expected, eukaryotic cells are more complex and usually much larger than prokaryotic cells. The diameter of a typical prokaryotic cell is on the order of 1 to 3 µm (1 × 10–6 to 3 × 10–6 m), whereas that of a typical eukaryotic cell is about 10 to 100 µm. The distinction between prokaryotes and eukaryotes is so basic that it is now a key point in the classification of living organ- isms; it is far more important than the distinction between plants and animals. The main difference between prokaryotic and eukaryotic cells is the existence of organelles, especially the nucleus, in eukaryotes. An organelle is a part of the cell that has a distinct function; it is surrounded by its own membrane within the cell. In contrast, the structure of a prokaryotic cell is relatively simple, lacking membrane-enclosed organelles. Like a eukaryotic cell, however, a prokaryotic cell has a cell membrane, or plasma membrane, separating it from the outside world. The plasma membrane is the only membrane found in the prokaryotic cell. In both prokaryotes and eukaryotes, the cell membrane consists of a dou- ble layer (bilayer) of lipid molecules with a variety of proteins embedded in it. Organelles have specific functions. A typical eukaryotic cell has a nucleus with a nuclear membrane. Mitochondria (respiratory organelles) and an inter- nal membrane system known as the endoplasmic reticulum are also common to all eukaryotic cells. Energy-yielding oxidation reactions take place in eukary- otic mitochondria. In prokaryotes, similar reactions occur on the plasma mem- brane. Ribosomes (particles consisting of RNA and protein), which are the sites of protein synthesis in all living organisms, are frequently bound to the endo- plasmic reticulum in eukaryotes. In prokaryotes, ribosomes are found free in the cytosol. A distinction can be made between the cytoplasm and the cytosol. Cytoplasm refers to the portion of the cell outside the nucleus, and the cytosol is the aqueous portion of the cell that lies outside the membrane-bounded or- ganelles. Chloroplasts, organelles in which photosynthesis takes place, are found in plant cells and green algae. In prokaryotes that are capable of photosynthe- sis, the reactions take place in layers called chromatophores, which are extensions of the plasma membrane, rather than in chloroplasts. Table 1.3 summarizes the basic differences between prokaryotic and eukary- otic cells. TABLE 1.3 A Comparison of Prokaryotes and Eukaryotes Organelle Prokaryotes Eukaryotes Nucleus No definite nucleus; DNA present but Present not separate from rest of cell Cell membrane Present Present (plasma membrane) Mitochondria None; enzymes for oxidation reactions Present located on plasma membrane Endoplasmic reticulum None Present Ribosomes Present Present Chloroplasts None; photosynthesis (if present) is Present in localized in chromatophores green plants Copyright 2010 Cengage Learning. All Rights Reserved. May not be copied, scanned, or duplicated, in whole or in part. Due to electronic rights, some third party content may be suppressed from the eBook and/or eChapter(s). Editorial review has deemed that any suppressed content does not materially affect the overall learning experience. Cengage Learning reserves the right to remove additional content at any time if subsequent rights restrictions require it. 16 CHAPTER 1 Biochemistry and the Organization of Cells 1.5 Prokaryotic Cells Although no well-defined nucleus is present in prokaryotes, the DNA of the cell is concentrated in one region called the nuclear region. This part of the cell directs the workings of the cell, much as the eukaryotic nucleus does. How is prokaryotic DNA organized without a nucleus? Cell membrane The DNA of prokaryotes is not complexed with proteins in extensive arrays with specified architecture, as is the DNA of eukaryotes. In general, there is only a single, closed, circular molecule of DNA in prokaryotes. This circle of Ribosomes DNA, which is the genome, is attached to the cell membrane. Before a prokary- otic cell divides, the DNA replicates itself, and both DNA circles are bound to the plasma membrane. The cell then divides, and each of the two daughter Cell wall cells receives one copy of the DNA (Figure 1.12). In a prokaryotic cell, the cytosol (the fluid portion of the cell outside the A. B. Dowsett/SPL/Photo Researchers, Inc. Nuclear region nuclear region) frequently has a slightly granular appearance because of the (lighter area presence of ribosomes. Because these consist of RNA and protein, they are also toward center of cell) called ribonucleoprotein particles; they are the sites of protein synthesis in all or- ganisms. The presence of ribosomes is the main visible feature of prokaryotic cytosol. (Membrane-bound organelles, characteristic of eukaryotes, are not found in prokaryotes.) Every cell is separated from the outside world by a cell membrane, or plasma membrane, an assemblage of lipid molecules and proteins. In addition to the FIGURE 1.12 Electron micrograph of a cell membrane and external to it, a prokaryotic bacterial cell has a cell wall, bacterium. A colored electron microscope image of a typical prokaryote: the bacterium Escherichia which is made up mostly of polysaccharide material, a feature it shares with coli (magnified 16,500×). The pair in the center eukaryotic plant cells. The chemical natures of prokaryotic and eukaryotic cell shows that division into two cells is nearly complete. walls differ somewhat, but a common feature is that the polymerization of sug- ars produces the polysaccharides found in both. Because the cell wall is made up of rigid material, it presumably serves as protection for the cell. 1.6 Eukaryotic Cells Multicellular plants and animals are eukaryotes, as are protista and fungi, but obvious differences exist among them. These differences are reflected on the cellular level. One of the biggest differences between eukaryotes and prokary- otes is the presence of subcellular organelles. Three of the most important organelles in eukaryotic cells are the nucleus, the mitochondrion, and the chloroplast. Each is separated from the rest of the cell by a double membrane. The nucleus contains most of the DNA of the cell and is the site of RNA synthesis. The mitochondria contain enzymes that cata- lyze important energy-yielding reactions. Chloroplasts, which are found in green plants and green algae, are the sites of photosynthesis. Both mitochondria and chloroplasts contain DNA that dif- fers from that found in the nucleus, and both carry out transcription and pro- tein synthesis distinct from that directed by the nucleus. Plant cells, like bacteria, have cell walls. A plant cell wall is mostly made up of the polysaccharide cellulose, giving the cell its shape and mechanical stabil- ity. Chloroplasts, the photosynthetic organelles, are found in green plants and algae. Animal cells have neither cell walls nor chloroplasts; the same is true of some protists. Figure 1.13 shows some of the important differences between typical plant cells, typical animal cells, and prokaryotes. What are the most important organelles? The nucleus is perhaps the most important eukaryotic organelle. A typi- cal nucleus exhibits several important structural features (Figure 1.14). It is Copyright 2010 Cengage Learning. All Rights Reserved. May not be copied, scanned, or duplicated, in whole or in part. Due to electronic rights, some third party content may be suppressed from the eBook and/or eChapter(s). Editorial review has deemed that any suppressed content does not materially affect the overall learning experience. Cengage Learning reserves the right to remove additional content at any time if subsequent rights restrictions require it. 1.6 Eukaryotic Cells 17 Typical Animal Cell Cell membrane Endoplasmic reticulum with ribosomes attached Nucleus Typical Plant Cell Lysosome Chloroplast Endoplasmic reticulum Mitochondrion Ribosomes Nucleus Cell wall Golgi apparatus Vacuole Cell membrane Prokaryotic Cell DNA Ribosomes Plasma membrane Cell wall FIGURE 1.13 A comparison of a typical animal cell, a typical plant cell, and a prokaryotic cell. surrounded by a nuclear double membrane (usually called the nuclear envelope). One of its prominent features is the nucleolus, which is rich in RNA. The RNA of a cell (with the exception of the small amount produced in such organelles as mitochondria and chloroplasts) is synthesized on a DNA template in the nucleolus for export to the cytoplasm through pores in the nuclear membrane. This RNA is ultimately destined for the ribosomes. Also visible in the nucleus, frequently near the nuclear membrane, is chromatin, an aggregate of DNA and protein. The main eukaryotic genome (its nuclear DNA) is duplicated before cell division takes place, as in prokaryotes. In eukaryotes, both copies of DNA, which are to be equally distributed between the daughter cells, are associated with protein. When a cell is about to divide, the loosely organized strands of chromatin become tightly coiled, and the resulting chromosomes can be seen under a microscope. The genes, responsible for the transmission of inherited traits, are part of the DNA found in each chromosome. A second very important eukaryotic organelle is the mitochondrion, which, like the nucleus, has a double membrane (Figure 1.15). The outer membrane has a fairly smooth surface, but the inner membrane exhibits many folds called cristae. The space within the inner membrane is called the matrix. Oxidation processes that occur in mitochondria yield energy for the cell. Most of the Copyright 2010 Cengage Learning. All Rights Reserved. May not be copied, scanned, or duplicated, in whole or in part. Due to electronic rights, some third party content may be suppressed from the eBook and/or eChapter(s). Editorial review has deemed that any suppressed content does not materially affect the overall learning experience. Cengage Learning reserves the right to remove additional content at any time if subsequent rights restrictions require it. 18 CHAPTER 1 Biochemistry and the Organization of Cells Double Pore in Nucleolus membrane membrane Courtesy of Dr. Sue Ellen Gruber, Mt. Holyoke College FIGURE 1.14 The nucleus of a tobacco leaf cell Vacuole Chromatin Immature (magnified 15,000×). granules choroplasts Outer membrane Inner membrane Courtesy of Dr. Sue Ellen Gruber, Mt. Holyoke College FIGURE 1.15 Mouse liver mitochondria Matrix Cristae Ribosomes Rough endoplasmic (magnified 50,000×). reticulum enzymes responsible for these important reactions are associated with the in- ner mitochondrial membrane. Other enzymes needed for oxidation reactions, as well as DNA that differs from that found in the nucleus, are found in the internal mitochondrial matrix. Mitochondria also contain ribosomes similar to those found in bacteria. Mitochondria are approximately the size of many bac- teria, typically about 1 µm in diameter and 2 to 8 µm in length. In theory, they may have arisen from the absorption of aerobic bacteria by larger host cells. The endoplasmic reticulum (ER) is part of a continuous single-membrane system throughout the cell; the membrane doubles back on itself to give the appearance of a double membrane in electron micrographs. The endoplasmic reticulum is attached to the cell membrane and to the nuclear membrane. It oc- curs in two forms, rough and smooth. The rough endoplasmic reticulum is studded Copyright 2010 Cengage Learning. All Rights Reserved. May not be copied, scanned, or duplicated, in whole or in part. Due to electronic rights, some third party content may be suppressed from the eBook and/or eChapter(s). Editorial review has deemed that any suppressed content does not materially affect the overall learning experience. Cengage Learning reserves the right to remove additional content at any time if subsequent rights restrictions require it. 1.6 Eukaryotic Cells 19 Mitochondria Courtesy of Dr. Sue Ellen Gruber, Mt. Holyoke College “Double” membranes Ribosomes (formed by doubling back FIGURE 1.16 Rough endoplasmic reticulum of single membranes) from mouse liver cells (magnified 50,000×). with ribosomes bound to the membrane (Figure 1.16). Ribosomes, which can also be found free in the cytosol, are the sites of protein synthesis in all organisms. The smooth endoplasmic reticulum does not have ribosomes bound to it. Chloroplasts are important organelles found only in green plants and green algae. Their structure includes membranes, and they are relatively large, typi- cally up to 2 µm in diameter and 5 to 10 µm in length. The photosynthetic apparatus is found in specialized structures called grana (singular granum), membranous bodies stacked within the chloroplast. Grana are easily seen through an electron microscope (Figure 1.17). Chloroplasts, like mitochon- dria, contain a characteristic DNA that is different from that found in the nu- cleus. Chloroplasts and mitochondria also contain ribosomes similar to those found in bacteria. What are some other important components of cells? Membranes are important in the structures of some less well-understood organ- elles. One, the Golgi apparatus, is separate from the endoplasmic reticulum but is frequently found close to the smooth endoplasmic reticulum. It is a series of membranous sacs (Figure 1.18). The Golgi apparatus is involved in secretion of proteins from the cell, but it also appears in cells in which the primary function is not protein secretion. In particular, it is the site in the cell in which sugars are linked to other cellular components, such as proteins. The function of this organelle is still a subject of research. Other organelles in eukaryotes are similar to the Golgi apparatus in that they Biophoto Associates/Photo Researchers, Inc. involve single, smooth membranes and have specialized functions. Lysosomes, for example, are membrane-enclosed sacs containing hydrolytic enzymes that could cause considerable damage to the cell if they were not physically sepa- rated from the lipids, proteins, or nucleic acids that they are able to attack. Inside the lysosome, these enzymes break down target molecules, usually from outside sources, as a first step in processing nutrients for the cell. Peroxisomes are similar to lysosomes; their principal characteristic is that they contain en- zymes involved in the metabolism of hydrogen peroxide (H2O2), which is toxic to the cell. The enzyme catalase, which occurs in peroxisomes, catalyzes the Double membrane Grana conversion of H2O2 to H2O and O2. Glyoxysomes are found in plant cells only. FIGURE 1.17 An electron microscope image They contain the enzymes that catalyze the glyoxylate cycle, a pathway that con- of a chloroplast from the alga Nitella (magnified verts some lipids to carbohydrate with glyoxylic acid as an intermediate. 60,000×). Copyright 2010 Cengage Learning. All Rights Reserved. May not be copied, scanned, or duplicated, in whole or in part. Due to electronic rights, some third party content may be suppressed from the eBook and/or eChapter(s). Editorial review has deemed that any suppressed content does not materially affect the overall learning experience. Cengage Learning reserves the right to remove additional content at any time if subsequent rights restrictions require it. 2 CHAPTER 1 Biochemistry and the Organization of Cells How did living things originate? The fundamental similarity of cells of all types makes speculating on the origins of life a worthwhile question. How did the components of our bodies come to be and to do the things that they do? What are the molecules of life? Even the structures of comparatively small biomolecules consist of several parts. Large biomolecules, such as proteins and nucleic acids, have complex structures, and living cells are enormously more complex. Even so, both molecules and cells must have arisen ultimately from very simple molecules, such as water, methane, carbon dioxide, ammonia, nitrogen, and hydrogen (Figure 1.1). In turn, these simple Body system of organism Organ Atoms Oxygen and hydrogen Bone Tissue Molecules O H H Water Bone tissue Cell Macromolecules Nucleus Protein Plasma Golgi membrane Bone cell Nucleus Organelles Mitochondria FIGURE 1.1 Levels of structural organization in the human body. Note the hierarchy from simple to complex. Copyright 2010 Cengage Learning. All Rights Reserved. May not be copied, scanned, or duplicated, in whole or in part. Due to electronic rights, some third party content may be suppressed from the eBook and/or eChapter(s). Editorial review has deemed that any suppressed content does not materially affect the overall learning experience. Cengage Learning reserves the right to remove additional content at any time if subsequent rights restrictions require it. 20 CHAPTER 1 Biochemistry and the Organization of Cells Don W. Fawcett/Photo Researchers, Inc. FIGURE 1.18 Golgi apparatus from a Stack of flattened mammalian cell (magnified 25,000×). membranous vesicles The cytosol was long considered nothing more than a viscous liquid, but re- cent studies by electron microscopy have revealed that this part of the cell has some internal organization. The organelles are held in place by a lattice of fine strands that seem to consist mostly of protein. This cytoskeleton, or microtrabe- cular lattice, is connected to all organelles (Figure 1.19). Many questions remain about its function in cellular organization, but its importance in maintaining the infrastructure of the cell is not doubted.