Biochem 3382 Exam Prep PDF
Document Details
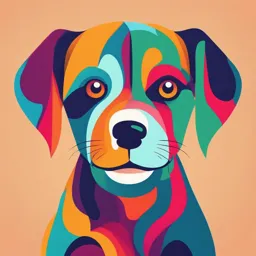
Uploaded by TriumphantLongBeach4234
Western University
Tags
Summary
This document covers the structure of nucleic acids, including DNA and RNA, and describes how factors such as base stacking, base pairing, and ionic interactions stabilize their structure. It explains the effects of intrinsic and environmental factors, such as solvent and ion concentration, on the stability of DNA and RNA. The content also covers DNA's supercoiling and the actions of topoisomerases.
Full Transcript
Nucleic Acid Structure Learning Outcomes 1. Compare and contrast the different helical structures formed by DNA. - DNA double helix - In aq solvent, two complementary DNA strands form double helix - 18 A across - ...
Nucleic Acid Structure Learning Outcomes 1. Compare and contrast the different helical structures formed by DNA. - DNA double helix - In aq solvent, two complementary DNA strands form double helix - 18 A across - Each base is 3.4 A thick with a 2.6 A gap (allows water to enter) - Gap must be closed. - Cannot just press down (energetically unfavourable) - Must stagger but leaves a lot of open surface area - Turning it covers as much surface area as possible using degrees of freedom and is energetically favourable to twist - B-DNA - Right-Handed - Helix width = 2.4 nm - Base pair spacing = 0.34 nm - One turn = 3.4 nm, 10 bp/turn - Major and Minor Grooves - Major has more distance between the phosphate backbone and is wider and deeper - Base tilt = -1.2° - B-DNA has a slight tilt - Propeller twist = 16° - A-DNA - Right-Handed - Helix width = 2.6 nm (longer) - Base pair spacing = 0.25 nm (shorter) - One turn = 2.5 nm, 11 bp/turn (shorter but one more base) - Much larger major groove - Base tilt = 19° (way more) - Propeller twist = 18° (slightly more) - Very temporary structure - Higher energy than B-DNA - Z-DNA - Left-Handed - 12 bp/turn (1 more than A, 2 more than B) - Alternating Pu-Py - Affects orientation of backbone - Pu x-angles are syn - Much more shallow major groove - Very temporary structure 2. Describe the forces that stabilize nucleic acid structure. - Base Stacking - GC bonds stronger than AT bonds - AT is worse at forming base stacks - Induced dipole + partial charge interactions between base rings - Sequence dependent - GC had more negative delta G = More stable = Favourable - Different Sequence means different partial charges and different energies - GC/GC does not equal CG/CG - Base pairing - DNA typically A-T and G-C - H-Bonds between these bases - Only advantage over H2O : COOPERATIVITY - Negative delta G for forming double-helix is more favourable than pairing w/ Water in our cells - Three separate bonds w/ water vs three bonds at the same time w/ bases - Not as important as base stacking though - Ionic Interactions - H-bonding plays a small role - Cations (floating around) shield repulsion between negative phosphates (in backbone) - Phosphates repel each other due to being in close proximity in the helix. - Cation-DNA interactions are mediated by H2O - Divalent Cations better at shielding than monovalent cations . Predict the effect of intrinsic and environmental factors on stability of a DNA 3 double helix. - DNA melting curves - Single strands absorb more energy than duplex - As it heats up, it denatures, and absorbs way more energy - Higher % GC content = higher melting point due to stronger bonds - Length and sequence of DNA strands - More GC = Higher melting point - GC often used at ends of primers, help primers stick on to the DNA - Longer length = Higher melting point - Nature of the solvent - High polarity solvent = Higher melting point - Concentration and types of ions present - pH . Explain how and why individual elements of DNA structure can deviate from 4 the average structure. - Many degrees of freedom of rotation in DNA - There are ways to “wiggle” DNA to reach the optimal formation - Intra-base pair coordinates (between two bases) - Shear - Buckle - Stretch - Propeller - Stagger - Opening - Inter-base pair coordinates (between stacked base pairs) - Shift - Tilt - Slide - Roll - Rise - Twist - What causes DNA to flex and utilize degrees of freedom? - DNA replication, transcription, wrapping around histones, etc 5. Describe the types of secondary and tertiary structures formed by RNA. - RNA vs DNA - RNA = Ribose, DNA = Deoxyribose - DNA more stable, RNA less stable - RNA has shorter lifespan - Hydroxide in RNA ribose more reactive - RNA = Uracil, DNA = Thymine - RNA = SS, DNA = DS - RNA can be enzymatic - RNA can self base-pair, DNA cannot typically - RNA has many base modifications - DNA = Long, RNA = Short - DNA stays in nucleus, RNA moves around - RNA forms complex structures - DNA has introns - RNA can recognize different molecules - RNA Structure - Stabilized by same forces as DNA (Base Stacking, Base Pairing, Ionic interactions) - RNA Structural elements (Can fold back on itself but may not base pair perfectly) - Duplexes (normal base pairing) - Single stranded regions (between base paired elements) - Hairpins - Hairpin stem = paired duplex region - Hairpin loop = Region with no pairing - Bulges - Can be a single base or multiple - Base that doesn’t pair within a duplex structure bulges out - Internal loops - Like bulges but on both side of duplex - Can be single or multi base - Can be asymmetrical or symmetrical - Junctions - Three Stem, Four Stem, etc - Pseudoknots - Loops around itself, looks like a knot - Relatively stable, good at interacting w/ other molecules - Can conformationally switch - Results in different functional properties - Can result in frameshifts, different regulation of expression, different control of initiation - Can result in general gene expression differences - Often involved in ribosome interactions - Unconventional base-pairing - Mostly GC / AU but other (non-perfect) pairing possible - Far less common but still occur Supercoiling Learning Outcomes 1. Determine the twist and handedness of example deoxyribonucleic acids - Twist (T) - Number of complete turns one strand makes around the axis of the helix - Depends on strand length - Right Hand turns = Positive, Left Hand turns = Negative - In B-DNA, 10 bp/turn = most energetically favourable - T = #bp / 10 - Changing the twist - Keep bottom of helix still and twist top clockwise - Twist would decrease / “underwound” (Lower T) - Twist top counterclockwise - Twist would increase / “overwound” (Higher T) - B-DNA “prefers” 10 bp/turn, therefore underwinding and overwinding introduces tension - Supercoiling - Relieves tension by reverting back to 10 bp/turn which generates supercoiling - Underwound RH DNA = negative supercoiling - Overwound RH DNA = positive supercoiling 2. Compare the mechanisms of action of the different classes of topoisomerase - Topoisomerases alter DNA coiling - Clips DNA, relieving tension and rebinds - Type I: Create transient single-strand breaks - IA - Moves in one direction - Strand in back comes to front - Relaxes negative supercoiling - No ATP used - IB - Moves in any direction - Helix rotates at cleavage site - No ATP used - Relaxes negative or positive supercoiling - Type II: Create transient double-strand breaks - trand in back comes to front S - Highly regulated - Moves in any direction - Uses ATP - Relaxes negative or positive supercoiling Type IA Type IB Type II Single-strand break Single-strand break Double-strand break No ATP No ATP Requires ATP Relaxes negative supercoils Relaxes any supercoil Relaxes any supercoil . Relate unwinding, underwinding, overwinding, and topoisomerase activity to 3 changes in DNA structure. - Needed to regulate supercoiling during transcription + replication - Needed to separate DNA during cell division - Example: Bacterial DNA Gyrase - Type II - Uses ATP to introduce negative supercoiling - 4 subunit protein interacts w/ DNA - DNA is cut + conformational change allows DNA to pass through - Regulated by Gyrase and then released Nucleotide Metabolism Learning Outcomes . Explain in general terms how eukaryotes synthesize and catabolize purine 1 nucleotides - Importance of NT metabolism - Imbalance in nts = less nucleic acid synthesis = genomic instability - Polymerase may stall / make errors - Hydrolysis of NTPs gives energy that drives many biochemical rxns - Higher energy nts have greater amounts (ATP, GTP, etc) - Involved in regulation - Target of anti-cancer therapies - Purine synthesis - tart w/ Ribose-5-Phosphate (from pentose phosphate pathway) S - 2 phosphates attach from ATP, Giving PRPP - PRPP becomes Phosphoribosyl-ß-1-amine - 9 steps to become IMP - ATP Path: - GTP gives a phosphate to reach Adenylosuccinate - Becomes AMP - Gains phosphate from ATP to become ADP - From Adenylate kinase - Gains another phosphate group to become ATP - From ATP synthase - GTP Path: - Becomes XMP - Gains two phosphates from ATP to become GMP - Gains a phosphate from ATP to become GDP - Guanylate kinase - Gains a phosphate from ATP to become GTP - Non-specific nucleoside diphosphate kinase - GTP is needed to form ATP and ATP is needed to form GTP - ATP from the ATP path feeds back into GTP path in a cycle when cell is in a good energy situation - A ll GXP and AXP products feed back to the PRPP → P-ß-1-amine step and inhibit allosterically - Stops Purine synthesis - GDP and ADP feed back to the R-5-P → PRPP step and inhibit allosterically - Stops Purine synthesis - GMP feeds back to the IMP → XMP step and inhibits competitively - Competes w/ ATP at active site because similar structure - AMP feeds back to the IMP → Adenylosuccinate step and inhibits competitively - Competes w/ GTP at active site because similar structure - Purine Degradation from monophosphates - Step 1: Remove phosphate - AMP can either remove phosphate first and then convert to Inosine or convert to IMP and then remove phosphate - Step 2: Remove sugar (ribose) - Step 3: Convert to Xanthine (XMP already became Xanthine) - Step 4: Convert to Uric Acid which is then excreted in Urine (removed from body) - Free purines can be re-added to ribose . Explain in general terms how eukaryotes synthesize and catabolize pyrimidine 2 nucleotides. - Pyrimidine Synthesis - etabolism leads to Carbamoyl Phosphate M - Add Aspartate and synthesize Orotate - Two phosphates leave PRPP, leaving PR which attaches to Orotate, forms OMP - OMP becomes UMP - Phosphate from ATP to become UDP - Phosphate from ATP to become UTP - Phosphate from ATP to become CTP - Uracil to Cytosine - Thymine uses a different pathway - U DP and UTP feed back to Glutamine → Carbamoyl Phosphate step and inhibit allosterically - PRPP feeds back to ATP in Glutamine → Carbamoyl Phosphate step and give positive feedback (enhancer) - dTMP is made from dUDP or dCDP - d CDP becomes dCMP and then dUMP - dUDP gets phosphorylated by Non-specific nucleotide kinase to dUTP and quickly converted to dUMP - Done quickly to prevent uracil from entering DNA - Dangerous, could replace dTTP and eventually replace w/ Cytosine - AT → AU → AC →GC - dUMP becomes dTMP then dTDP then finally dTTP - Pyrimidine degradation from free bases - N H4+ and CO2 removed from the pyrimidine (Cytosine,Uracil, Thymine) - Converted into Malonyl-CoA (Cytosine and Uracil) or Methylmalonyl-CoA (Thymine) - Used in Fatty Acid Metabolism - Purines - IMP leads to GTP and ATP formation - Used in RNA - Pyrimidines - UMP leads to UTP and CTP formation - Used in RNA - Ribonucleotide Reductase - Takes GDP, ADP, UDP and CDP (comes from CTP) and turns them into dNDPs - dADP → dATP - dGDP → dGTP - dCDP → CTP - dCDP → dUMP → dTMP → dTDP → dTTP - dUDP → dUTP → dUMP → dTMP → dTDP → dTTP - All dNTP (except dUTP) used in DNA - ll GXP and AXP products from IMP inhibit Purine synthesis A - UTP and UDP from UMP inhibit Pyrimidine synthesis - dATP inhibits Ribonucleotide Reductase enzyme - ATP promotes Pyrimidine synthesis and Ribonucleotide Reductase enzyme . Make predictions about the effects of changes in the system on flux through 3 nucleotide metabolism - Ribonucleotide reductase makes dNDPs - Reduces any NDP to dNDP (CTP → CDP → dCDP) - Works with ATP, GTP, and UTP too - One OH becomes an H (deoxy) - - e come from NADPH - dNDPs then phosphorylated into dNTPs - Ribonucleotide reductase is regulated - Activity site balances NDPs vs dNDPs - Activity-determining effectors: ATP, dATP - Specificity site balances dNDPs relative to each other - Specificity-determining effectors: ATP, dATP, dGTP, dTTP - Active site accepts substrates - Substrates: ADP, CDP, UDP, GDP Activity Site: ATP ATP ATP dATP Spec Site: ATP dTTP dGTP Removes ATP Active Site: CDP, UDP GDP ADP ENZYME OFF Forms: dCTP, dTTP dGTP dATP ENZYME OFF - Starts w/ ATP in Activity site and Spec. site - Enzyme accepts CDP and UDP - Results in formation of dCTP and dTTP - Some dCTP and dUTP used to synthesize dTTP - dTTP levels build up until - dTTP now in Spec. Site - Blocks formation of dCTP and dTTP - Enzyme accepts GDP - Results in formation of dGTP which builds up until - dGTP now in Spec. Site - Blocks formation of dGTP - Enzyme accepts ADP - Results in formation of dATP which builds up until - dATP now in ACTIVITY site - ATP removed from activity site - Enzyme now OFF, no more dNTPs being produced Regulation of DNA Replication Learning Outcomes . Describe the basic process of DNA replication in bacteria and eukaryotes, 1 including how supercoiling is created and regulated - Overview of DNA replication - Origin begins unwinding and helicase is recruited - Helicase unzips DNA - Primase recruited to DNA - RNA primers added to DNA - Sliding clamps recruited to DNA - Clamps recruited and hold DNA in place - Polymerase is recruited to DNA - New DNA begins forming - Bidirectional fork with two leading and two lagging strands - E. Coli replication fork - Uses DNA Gyrase (Bacterial enzyme) - Uses single-strand binding protein - Keeps strands separated and unfolded (won’t bind w/ itself) - Protect DNA from damage (w/out them DNA is very exposed) - Uses DNA polymerase III holoenzyme Function E. Coli Protein(s) Eukaryotic Protein(s) Helicase DnaB MCM2-6, CDC45, PCNA Sliding Clamp ß-Subunit Dimer PCNA Clamp Loader T-Complex Replication Factor C (RFC) Replicative Polymerase Pol III ol δ (lagging) Pol ε P (leading) Primase DnaG ol α + primase + P accessory protein Single-Strand Binding SSB eplication Protein A R (RPA) Topoisomerase NA Gyrase, D TOP1, TOP2α Topoisomerase IV - Replication fork dynamics - Lagging strand pol dissociates from helicase (must reconnect) - Leading strand polymerase sometimes pauses - Okazaki fragment maturation - E.Coli - Pol III dissociates at primer - Pol I digests primer while completing synthesis - Exonuclease activity - DNA ligase I repairs remaining nick in DNA - Eukaryotes - Pol δ displaces primer during synthesis - Pushed out of the way - Primer removed by flap endonuclease - Fen1 endonuclease cleaves off section - DNA ligase I repairs remaining nick in DNA - Negative supercoiling generated ahead of replication fork - Regulation of supercoiling during replication - Type IB or Type II topoisomerases used (e.g. DNA gyrase) - If coiling piles up, DNA can’t be unwound and Pol can’t work - Gyrase hydrolyzes DNA + introduces negative supercoils. - Unwinding favoured in vivo - In bacteria, DNA gyrase introduces negative supercoiling - Eukaryotic DNA is wrapped around histones . Explain how initiation of DNA replication is regulated in E. coli and Eukaryotes 2 E.Coli - OriC, the E. coli replication origin - Has one 245-bp origin - DNA adenine methyltransferase methylates GATC on both strands - DnaA = initiator protein - DNA binding protein binds next to AT rich sequence called “unwinding element” - AT because weaker base stacking = easier to pull apart - Initiation of E. coli replication - DnaA-ATP active, DnaA-ADP inactive - Has good affinity to binding sites when bound to ATP - Control: ATP hydrolysis and nt exchange - After ADP hydrolyzed, needs to be replaced with fresh ATP - Forms open complex w/ HU - DnaC loads DnaB helicase onto strands, forms prepriming complex. - Regulation of initiation by nucleotide exchange - ATP hydrolysis - during initiation (RIDA) - At DatA sites - IHF encourages DnaA-ATP to bind to datA - dat-A encourages hydrolysis of ATP to ADP (clean-up of cell) - Nucleotide exchange (to remove ADP from DnaA) promoted by: - Binding to DARS sites - When enough DnaA-ADP exists, DARS1 encourages it to let go of ADP - DnaA can now bind more ATP - Newly synthesized DnaA preferentially binds ATP - Acidic phospholipids - Regulation of initiation by exclusion of DnaA from origin - Newly replicated DNA = hemimethylated at oriC - Only methylated on old strand, not new strand - SeqA binds hemimethylated sites, blocking DnaA from binding - Cannot bind, replication cannot start - Full methylation by dam methylase in ~10 min - DnaA-ATP can bind after fully methylated Eukaryotes - Eukaryotic cell cycle - Regulated by cyclin-dependent kinases (CDKs) - Only passes to next phase if all checks are passed - Cyclins expressed only during a given phase - Specific cyclins for each phase - Stalled replication forks trigger DNA damage signal - Prevents cell cycle progression - Initiation of eukaryotic replication - Multiple Ori distributed throughout multiple chromosomes 3-300 kbp apart - No consensus sequence - Nucleosome-free regions favoured - Ori must be used at most ONCE per cell cycle - Using ori more than once = extra copies of DNA (not epic!) - Two stages - Licensing (recruitment of helicases) - Activation - Licensing of replication Oris - G1 phase 1. ORC hexamer binds DNA 2. Cdc6 binds ORC 3. Cdt1 binds MCM2-7 hexamer (helicase) 4. Cdt1-MCM2-7 binds ORC-Cdc6 5. MCM2-7 is loaded onto dsDNA 6. MCM2-7 hydrolyzes ATP, releasing Cdc6 & Cdt1 7. Cdc6 binds same or diff. ORC 8. Another Cdt1-MCM2-7 binds ORC-Cdc6 9. MCM2-7 loaded onto dsDNA 10.MCM2-7 hydrolyzes ATP, releasing ORC, Cdc6, Cdt1 - Ori is licensed but not activated - Prevention of licensing in S phase - Phosphorylated CDC6 - Exported to cytosol - Phosphorylated ORC2 - Reduced affinity for chromatin, less likely to bind DNA - Phosphorylated ORC1 - Degradation - Phosphorylated CDT1 - Degradation - CDT1 bound by geminin - Stops CDT and MCM from interacting - Geminin degraded in G1 phase - Activation of Eukaryotic Replisome - CDK2 + DDK active in S phase - Helicase activated - MCM2-7 + other proteins phosphorylated to load Cdc45 and GINS - RFC, PCNA, RPA and polymerases recruited - Most licensed Oris are not activated . Predict the impact of changes in function of specific proteins on the process of 3 DNA replication 4. Interpret experimental data in the context of current models of replication DNA Damage & Repair Learning Outcomes 1. Explain the difference between DNA damage and DNA repair - DNA damage = chemical alteration to DNA - Not necessarily leading to mutation which is a change in base pair - DNA repair mechanisms sense + correct DNA damage - Some introduce mutations - Error-prone repair = advantageous - Promotes genetic variation - Causes of DNA damage - Environmental agents: UV light, heat - UV light (200-300 nm) - Promotes cyclobutyl ring formation between thymines on same strand - Causes INTRASTRAND DNA dimers (Thymine dimers) - Pyrimidine dimers locally distort DNA structure + interfere w/ transcription and replication - Polymerase can’t go over formed kink - Kink on outside of DNA (backbone) - Chemical agents (mutagens): Benzene (metabolites = ROS) - Categorized into four general groups based on interaction mechanism w/ DNA - Base analogs - Molecules similar to bases that can replace them, pairing incorrectly - Base altering chemicals - Base change, e.g. cytosine to uracil from nitrous acid - Intercalating agents - e.g. Ethidium bromide - Agents altering DNA structures - Can cause inter and intra bonds between bases, changing DNA structure - Have two main effects on DNA: - Point mutations (one base replaced) - e.g. Cytosine deamination → Uracil - CG → UG → TG → TA - Insertions/Deletions (genetic material gained or lost) - e.g. Acridine orange the intercalating agent - Stacks between nitrogenous bases, creates atypical spacing - Disrupts base pairing during replication - Extra bases added or bases removed - Spontaneous mutations: Errors in DNA replication/repair - Errors not corrected by proofreading of DNA Pol - Mismatches, inserted base does not pair with base in template strand - Errors must be corrected before replication - Rate of spontaneous mutations correlated w/ efficiency of proofreading activity of DNA Pol (very low) - Good to have a very very low rate, better than no errors - Natural mutations may drive evolution - E.Coli error rate = 10-9 to 10-11 errors per basepair - Eukaryotic rate = ~10-10 errors per base pair - Combined action of Pol base selectivity, 3’-5’ exonucleolytic proofreading, mismatch correction, and DNA damage repair . Predict the consequences of DNA damage that can be caused by different 2 agents - Consequences of DNA damage - Silent mutations: - No biological consequence even though DNA sequence mutated - Could be in non-coding DNA region - Mutation may be a synonymous amino acid change (same AA) - Deleterious Mutations - Impact function - Could occur in regulatory DNA sequence - Could alter RNA secondary structure - Could occur in coding region - Mutations in coding region - Synonymous: Does NOT change codon identity - Nonsynonymous: DOES change codon identity - Nonsense: Creates TERMINATION codon - Readthrough: Converts termination codon to specify amino acid (doesn’t stop transcription) - Cytosine deamination - Cytosine loses its amino group due to nitrous acid and converts into Uracil - Uracil pairs w/ adenine instead of guanine - Does not stay very long in DNA; base excision repair pathway removes uracil - Result is a conversion of a CG to a TA base pair . Explain how different DNA repair pathways operate and the mechanisms for 3 sensing different types of DNA damage - 4 Different types of DNA repair systems - Direct repair: acts directly on damaged nucleotides - Excision Repair: excision of a base/segment of damaged DNA - Mismatch Repair: Corrects errors of replication - Nonhomologous end-joining: Used to repair double-strand breaks in DNA - UV light induces pyrimidine dimers in DNA - Direct and Excision repair both act on thymine dimers (REDUNDANCY!) - Photolyase Direct Repair - Experiment setup - E.Coli strain deficient in DNA repair carries plasmid w/ DNA photolyase gene - Exposed to varying doses of UV light then exposed to light/dark conditions - Survival measured as fraction relative to untreated cells - Results - Treatment w/ UV light results in cell death - Exposure to light AFTER UV promotes cell survival - Interpretation - DNA repair = essential process - DNA photolyase gene required to repair UV induced damage - Light is essential component of repair process - Photolyase reaction pathway 1. Formation of T=T dimer by UV < 300 nm 2. Recognition + binding of T=T by DNA Photolyase 3. Splitting of T=T + release of repaired DNA 4. Recycling of FADH-cofactor for next rxn cycle ○ Light stimulates e- transfer to activate FADH again - DNA is not cut - T=T flipped out of helix into enzyme active site and base pairs w/ adenine in FADH - Placental mammals do not do this, we use NER - Excision Repair - Base excision repair (BER): Repairs small, non-helical distortions - e.g. Uracil in DNA - Damaged base is cleaved off of backbone by DNA glycosylase, leaving single nucleotide gap (apurinic site / apyrimidinic site) - Base flipped out into active site of enzyme - DNA bent at 35 degrees in active site - DNA Pol + Ligase fill in gap - Different bad bases repaired by a unique glycosylase - E.g. Uracil DNA glycosylase (UDG) - Can work on both ssDNA and dsDNA - Incredibly sensitive, even slight molecular changes to substrate impact function - Requires C-N glycosidic bond (C-C bad) - Uses some info from ribose group as well as base - Nucleotide excision repair (NER): Repairs bulky, helical-distorting damage - e.g. Thymine dimers in DNA - Helicase opens helix - Endonuclease cuts backbone + removes nucleotides - Leaves large gap (multiple NTs removed) - DNA Pol fills gap, DNA ligase fills remaining nick - Thymine dimer repair: 1. T=T recognized by UvrA homodimer 2. UvrB recruited to form complex 3. UvrC recruited to make 5’ and 3’ incision on either side of T=T 4. UvrD displaces UvrC + DNA fragment via helicase activity 5. DNA Pol I and DNA ligase fill + seal gap - Human analogous protein = XP proteins (XPA-XPG) - XPC recognizes dimer - TFIIH associated w/XPC, opens dimer via helicase activity - XPA recruits more proteins - RPA = ss binding protein (protects section that won’t be removed) - XPF + XPG recruited by XPA to cleave DNA - Only cleave when both present - XPF = 5’ incision - XPG = 3’ incision - XPG, XPF, XPA and TFIIH leave, only RPA left - RPA leaves, Pol + Ligase recruited to fill gap - PCNA also, which is polymerase clamp - DNA repaired - Mismatch repair - Corrects errors of replication - Conserved pathway key for maintaining genome stability - Recognizes base-base mismatches/ insertions and deletions from replication - Critical activity = recognition of newly synthesized DNA strand w/mismatch - In bacteria, methylation is at N6 position of adenine residues in GATC - Every 44 bases = 256 bases - New DNA = hemimethylated (only methylated on template) - mutH, mutL, mutS, UvrD gene products required to repair - In vitro assay - Experimental setup - Plasmid w/ EcoRI and BamHI restriction sites - EcoRI site has mismatch, cannot be cleaved - Only 1 cut possible, long strand of DNA produced - Plasmid prepared w/ methylation on diff. Strands or both - Incubate w/ cell extracts from E.coli to initiate repair - Assay for repair by cleave w/ EcoRI and BamHI - Visualize on agarose gel - Expected results - If mismatch repair functional, EcoRI site repaired - Cleavage of plasmid = two products - If mismatch NOT functional, EcoRI site not repaired - Only BamHI cleaves = 1 product - Results - If methylation present on strand w/out mismatch - EcoRI site repaired - Methylation on BOTH strands - No repair, only BamHI cleaves - Important findings 1. Methylation must be on strand opposite to mismatch ○ Dictates which one is template 2. ATP required for process, NAD is not 3. No exogenous dNTPs needed, but there are dNTPs in cellular extract - Involved proteins in E.coli: - MutS: recognizes mismatches in DNA, recruits MutL - MutL + ATP: Initiates “looping” process to search for methylation - MutH: When methylated site found, it nicks daughter strand - UvrD + exonuclease: UvrD displaces strand, exonuclease degrades - DNA Pol/Ligase: Replicate + seal gap Homologous Recombination Transposons and NHEJ Learning Outcomes 1. Understand the differences between different types of recombination - Recombination: cellular processes that involve breakage and rejoining of polynucleotides - Genomes not static structures; constantly evolving and very dynamic - Potential source of extensive genome restructuring (promotes genetic variability) - Have mechanisms to both promote and suppress recombination - Deinococcus Radiodurans = Best DNA assembly in an organism - Shows that genome is undegraded over time after being degraded (is able to repair) - Mutations in recA lower the comeback repair - Types of recombination: - Homologous Recombination (general combination) - Occurs between segments of DNA molecules that share extensive sequence homology (identity) - E.g. sister chromosomes - Non-Homologous End Joining (NHEJ) - Cellular pathway for repairing double-strand breaks in DNA - Can result in insertions/deletions at break site - Transposition & Sight-Specific Recombination - Occurs between DNA molecules w/ only short regions of sequence similarity - Results in transfer of a DNA segment from one position in the genome to another - Recombination reminder: - Exchange of homologous segments between two DNA molecules - Not just for DNA repair, also for exchange + acquiring genetic info - Occurs in haploid and diploid organisms - Must either replicate or have sister chromatids - Nicked dsDNA Replication - Template strand gets nicked - Replication fork collapses at nicked site (cannot continue past it) - 5’ end at nicked site gets resectioned - 3’ end at nicked site invades the non-nicked dsDNA template strand (its sister copy) - Polymerase extends invading strand along template strand - Invading strand and template separate - Invading strand reinvades - Processive replication fork is made (creates missing segment using template strand) - Replication complete, nicked strand repaired - Homologous Recombination: Generally - Requires two homologous DNA molecules (sister chromatids/replication) - Must be close together 1. Proteins to make DNA w/ free 3’ end ○ RecBCD - Complex of 3 proteins (RecB, RecC, RecD) w/ helicase + nuclease activity - Initiates recombination 2. Proteins to promote alignment of homologous DNA strands ○ RecA - Mediates exchange between ssDNA and dsDNA by polymerizing on DNA 3. Proteins to resolve the Holliday Junction ○ RuvABC - Complex of proteins that mediates branch migration and resolution of the Holliday Junction - Results in 2 different possibilities depending on where Holliday Junction gets cut - Initiating recombination requires DNA strand w/ free 3’ end - Promotes loading of RecA + strand invasion w/ homologous strand - In E.Coli, generation of 3’ end = RecBCD complex’s function - Complex that binds to free ends of DNA - At dsBreak either from DNA exchange or damage - RecB = 3’-5’ helicase + nuclease - endo and exonuclease activity - Only happens at free DNA ends (heavily regulated) - RecD = 5’-3’ helicase - RecC = Regulatory protein - Controls how B and D function - Chi site = GCTGGTGG, occurs every 5kb - Not random, maintained in case of ds breaks - RecB exonuclease activity suppressed by RecC when Chi site is met - RecB nicks DNA on 5’-3’ strand (opposite strand) - Creates molecule w/ 3’ overhang (substrate for RecA) - RecBCD produces uniform molecule, improves efficiency of next step proteins - RecA promotes recombination - No sequence preference, RecA monomer binds 3 nucleotides - Many bind along the backbone - DNA in RecA filaments has extended structure of 18.6 nt per turn (B-DNA usually has 10.4) - Filament can contain 1000s of RecA monomers - RecA interacts w/ ssDNA: called initiation complex - RecA binds homologous dsDNA - Forms transient three-stranded helix to mediate correct pairing - Tolerates limited degree of mispairing - RecA rotates bases of aligned homologous strand - Mediates strand exchange - ATP depended process - “Spools in” homologous dsDNA template - Continues until RecA cannot mediate pairing (no homology) - Polymerase extends invading 3’ ends - Resolution of crossed-over junctions: - Gives two DNA segments w/ no breaks - Multiple ways to deal with crossed-over “Holliday Junctions” - Performed by RuvA, RuvB, RuvC - RuvA: recognizes + binds Holliday Junction - Tetramer (binds in four symmetry related groves) - Central pins = negatively charged; Facilitates separation of ssDNA segments - RuvB: Contacts RuvA, forms two hexameric rings on either side of Junction - ATPase - hexameric complex w/ central pore of ~30 A through which DNA passes - RuvC: Endonuclease that cleaves Holliday Junction - Branch migration not random, stops preferentially at: - Sequence 5’-(A/T)TT(G/C)-3’ - RuvAB dissociates - RuvC binds and cleaves Junction, resolving strands - Non-Homologous End Joining (NHEJ) - Most heavily used (cells can use it at any time) - Cellular pathway that repairs dsbreaks - Competes w/ homologous recombination but does not require complementary template (no homology needed) - Two NHEJ pathways w/ different repair outcomes: - Classical NHEJ (cNHEJ/NHEJ) - Done on blunt DNA ends/overhanging ends - Ku70/80 heterodimer senses dsBreaks - Bind to either side of break, holds and closes it together - Minimal end processing - DNA ends ligated back together via protein complex - Minimal loss of genetic information - 53BP1 + DNA-PK + MRE11 complex bind ends - Artmeis processes ends - XRCC4 Ligates ends - Alternative NHEJ (aNHEJ/MMEJ) - Some genetic info lost on either strands (or both) - DNA ends require processing - Generates “microhomologies” = complementary bases on ends that keep ends together - Uses MRE11 exonuclease complex (processes 5’ end) - More likely to cause DNA loss, insertions, deletions - Can cause chromosome translocations (especially in cancer) - Difference? - End processing is key factor in choosing with path to use - Blunt ends + compatible overhangs = cNHEJ - All other types = aNHEJ . Predict how perturbations in the recombination pathways effect the outcomes 2 of recombination events . Understand how DNA transposition and site-specific recombination can lead to 3 genome rearrangements - Mobile genetic elements/transposons - Transposable elements = ~45% of human genome - Source of genetic variability because of mobility - Mechanism of movement = site-specific recombination - Can cause disease in humans (~30 known genetic disorders) - Class I = Move through RNA intermediate - Retrotransposons (involves reverse transcriptase) - Class II = DNA intermediate - DNA Transposons - Course Focus - Two types: 1. Replicative transposition ○ Transposable element duplicated during transposition rxn ○ Increase in number of transposable elements ○ Involves two enzymes i. Transposase: Acts directly on transposon ii. Resolvase: Acts directly on duplicate 2. Nonreplicative transposition (cut & paste) ○ Transposable element moves from one position to another ○ Lost from donor site, gained at target site ○ Involves one enzyme: Transposase - Simplest form = Insertion Sequence (IS) - Encodes protein called Transposase + two other important sequence elements - Boundary of IS element = inverted DNA repeats - IS element flanked by duplicated target sequences - Transposition Pathway - Transposons move from one position to another in same genome - Often site-specific - Transposase protein targets specific sequence for insertion - Staggered cut made in DNA sequence (blunt ends) - Filling in gaps created by transposition reaction generates target site duplication (duplicated target sequence flanks) - IS elements often have more complicated structures - Encode genes not related to transposition (e.g. antibiotic resistance genes) - Composite transposons = Carry more than just transposition gene - larger + have gene-rich central region flanked by two IS-like modules - IS modules can be same or opposite orientation (direct vs inverted repeats) - Tn5 = composite transposon - Encodes three antibiotic resistance genes - Flanked by inverted IS modules ending in 19-base pair sequences called outside ends - Undergoes transposition by cut-and-paste mechanism - Monomer of Tn5 transposase binds to one outside end DNA - Synapsis rxn: transposase monomer + outside end DNA dimerize, form catalytically active synaptic complex - Transposon DNA held between transposase subunits - Yields free 3’OH group on one strand of DNA - Attacks opposite strand on outside end DNA - Forms hairpin structure - Excises transposon from DNA - Hairpin hydrolyzed, yields blunt-ended dsDNA at each end of transposon - Reintegration: synaptic complex binds to target DNA - 3’OH of transposon attacks target DNA on opposite strands (9 bp apart) - Repair of oppositely located single-strand gaps Protein-DNA Interactions Learning Outcomes . Understand the principles of how proteins recognize and interact with nucleic 1 acids - Why Study protein-nucleic interactions? - Key events in regulation of gene expression - Examining how transcription factors bind specific sequences gives clues into transcriptional regulation (important for cellular processes) - Allows prediction of binding sites - Can use info about protein-DNA interactions to change specificity of interactions for applications like gene editing - DNA’s two faces: Major and Minor - Proteins have 3D shape structurally and chemically complementary to surface of DNA - Can interact directly w/ residues in major/minor groove + phosphate backbone - Information content = available chemical interactions possible in specific sequence to describe specificity of an interaction - Structure of DNA dictates two types of interactions that read content: 1. Non-specific Interactions w/ DNA: Indirect readout 2. Specific interactions w/ DNA bases: Direct readout - Specificity + strength of an interaction = sum of direct and indirect contributions - Major + Minor groove = Chemically Distinct - Because of structure + geometry, diff. Chemical groups available in each groove for interactions - More information available in major groove interactions - # of specific molecular contacts is higher, therefore providing more specificity - Proteins use combination of specific + non-specific interactions to bind DNA - Non-specific protein-DNA interactions: - Do not discriminate what base is being contacted - Can be H-bonding to phosphate backbone, oxygen in DNA deoxyribose, and stacking interactions between aromatic residues and the ribose ring - Can happen through bridging water molecules - Many occur through minor groove - Specific go to major groove because it is just better, so nonspecific tend to go to minor groove. - Specific interactions harder to reach here, but still possible and can help enhance interactions - Specific protein-DNA interactions: - Discriminate what base is being contacted - Often H-bonding to acceptor/donor groups by amino acid side chains to bases - Many occur through major groove because this maximizes “information” about DNA binding site - Specificity of protein-DNA interactions is influenced by: - Nature of contacts - Specific vs non-specific - Length of target site - More DNA interacted with = Higher strength of interaction - Potentially higher specificity but not always - 12bp target site has more potential info than 11bp target site - How the protein interacts w/ the substrate - Is protein a monomer or dimer? (Dimer = double specificity) - e .g. EcoRV being a dimer, each subunit has 10 direct contacts + multiple indirect contacts = 20 contacts in 6bps - Helix-turn-helix motif very common - α-helix fits into major groove (same diameter as width of major groove) - Can interact with a lot of things in major groove because of this. - An example of structural and chemical complementarity between protein and DNA . Explain and interpret how protein-DNA interactions can be examined through 2 different experimental techniques - Structural information is “snapshot” of protein-DNA interactions - Many are dynamic, need to be probed via other methods - Typically require purified protein + prior knowledge of binding site - Can provide information about - Interaction binding strength - Cooperative binding - Minor vs Major groove contact - Often limited to one protein-DNA interaction at a time - Protein-DNA interactions can be very strong (Biotin is gold standard) - Proteins constantly binding and unbinding DNA/ligand in the cell - Strength represents how long it is in the bound state vs the unbound state - Method 1: Electrophoretic Mobility Shift Analysis (EMSA) / Gel-Shift Assay - DNA visualized by labelled w/32P or fluorescent marker,protein = invisible - Non-denaturing polyacrylamide gel - We don’t want to disrupt complexes - Polyacrylamide instead of agarose for better resolution + substrates are short DNA fragments - Smaller molecules (free DNA) move to bottom, larger molecules (bound DNA) are further up - Can test a wide range of protein concentration (low to high), keeping DNA concentration constant - At each concentration of protein [B], determine fraction of DNA [A] that is in complex with the protein [AB] - Plot fraction Abound vs [B] - Equation: KD = [P][L] / [PL] = kd / ka - Method 2: Quantitative DNaseI Footprinting - Based on DNaseI footprinting - Technique to map binding site of protein - DNaseI = non-specific nuclease; cuts DNA indiscriminately unless sequence is protected - Protein “protects” DNA from DNaseI degradation - Titrate conc. Of protein and generate binding curve describing amount of protection from DNaseI cleave vs. [protein] - No protein = DNaseI cutting DNA at all sites - Yes protein = DNaseI skipping region of protein that is protected - Endonuclease since it can jump to another site within sequence - Empty gap called “protein footprint” - Can be done over a wide range of protein concentrations - Generates protein-dependent change in protection signal - Compare to control region to generate binding curve - Shape of curve can tell us the affinity and type of interaction - Advantages: - Measures interactions under conditions of equilibrium (EMSA is non-equilibrium, and misses weak/unstable binding rxns) - Reports information on binding at individual sites (EMSA can’t) - Disadvantages: - Time consuming, only examining one interaction at a time (EMSA could potentially do more) - Doesn’t provide info on specific positions within footprinted region - Can be sterically hindered (protecting protein can keep DNaseI further away from the binding sequence than it actually is, giving larger footprint) - Other methods: - Chemical footprinting: provides single nucleotide resolution (band = 1 nt) - Use Hydroxy radicals to cleave phosphate backbone - Can provide more precise footprints (get much closer to protected region) - Disadvantage: Protein may not be able to prevent small molecules from slipping through and cleaving within the footprinted region - DMS footprinting - Treating DNA w/ DMS methylates N1 of adenine and N3 of cytosine - Positions can be probed by DMS and cleaved at unprotected AAs - Middle ground; chemical modification but not single nucleotide . Understand how modular proteins can be programmed to interact with 3 user-defined DNA sequence - Large amount of variability in Protein-DNA interactions - All kinds of ways proteins can interact w/ DNA - One residue could interact in many ways on its own - No good way to know exactly what sequence a protein should interact with - No amino acid-DNA “code” - No single AA contacts only one base - AA sequence gives no info about what DNA sequence it could contact - Many DNA-binding proteins can tolerate nucleotide substitutions in binding sites (difference of 1-2 or more nucleotides throughout length) - Interactions need to be displayed in easy to interpret and understand way - Helps discover other binding sites - Transcription factor binding sites in human genome - Additional information on what is important for interactions - Consensus sequence - Count the most frequent nt in a position, use arbitrary cut off value for how identical they need to be (e.g. 80% identical) - N = any nt, T/A = W (weak), C or T = Y (pyrimidine) - Useful, but information lost about relative frequency of each base - Sequence logos - Graphical representation of sequence conservation - Height of letter = frequency of letter - Total height = information content in bits - Calculations based on entropy at each position - High entropy = no information - Low entropy = more information - Four possible bases = log2( 4) = 2 max bits of information - Positions w/ high information likely critical for binding - Sequence logo does not tell why positions are critical - Provides more information about binding site than consensus sequences - Derived from a count table of nt frequencies at each position Regulation of Transcription: Prokaryotes Learning Outcomes . Understand key steps in initiation and termination of transcription by RNA 1 polymerase - Prokaryotes (Bacteria) - Single cell - No nucleus - No membrane-enclosed organelles - Inhabit diverse environment - Some are human pathogens - Can respond very quickly to changes in environmental conditions - Mostly due to transcription/repression of genes - Turn off genes not needed or turn on previously not needed genes - RNA is synthesized by a single type of DNA-dependent RNA polymerase, RNAP - Complex of proteins - Strand of DNA that is read/transcribed = template strand - Strand not transcribed = non-template/coding strand - Identical to any produced transcripts - RNA Polymerase - Core enzyme: α2ß ß’ω (alpha2, beta, beta prime, omega) - Omega likely structural, non essential - Positively-charged cleft binds DNA (backbone is negative) - Mg2+ in active site - Bacterial promoters - Nt sequences that determine start site of transcription initiation - ~40 nts on 5’ side of transcription start site - 2 consensus elements (conserved regions): - -10 region = Pribnow bow - Very AT rich - -35 region - Binding of σ subunit here determines transcription efficiency - σ Factors - E.coli has 7 - B.subtilis has 20 - Different σ factors allow regulation of different sets of genes - Respond to environmental conditions - Each factor recognizes different -35 region (σ24 =heat stress response) - Bacterial transcription: - Binding - σ subunit of RNAP recognizes specific sequences called promoters - Promoter complex goes from closed to open (DNA is unwound) - Open = melted, Kd becomes much higher for RNAP (higher binding affinity) - RNAP protects nt sequence from -70 to +20 (+1 = transcription start site) - Transcription start site not the same as translation initiation site - Key spots are -35 and -10 - Initiation and Elongation - Strand separation by promoter melting before initiation and elongation - RNAP binds two NTPs at initiation site - ATP/GTP preferred - Elongation site binds 2nd NTP - 3’OH of first NTP attacks α-P of 2nd NTP - Forms new phosphodiester bond (eliminates PPi) - 6-10 nts of elongation later, sigma subunit dissociates - σ subunit has high affinity for -35 and -10 region, therefore must dissociate or RNAP will be stuck there - Elongation - Core RNAP (no σ) = elongation enzyme - 1 error in 10,000 nts transcribed - Rate = ~50-90 nt/second - Transcription causes supercoiling, needs topoisomerase to remove negative and Gyrase to relieve positive supercoiling - Termination - Mediated by either intrinsic transcriptional terminator in RNA (hairpin) or Rho (protein) - Intrinsic terminators - Built into sequence - Can terminate at specific sites called termination sites - Inverted repeat sequence rich in GC bases forms stable stem-loop structure in RNA transcript - Small loop region between inverted repeats - Stretch of As in DNA template (U in RNA) - AU pairing destabilizes RNAP binding, terminating transcription - Rho-dependent termination - Rho = ATP-dependent helicase (unwinds mRNA) - Untangles from DNA - Rho translocates along RNA transcript until transcription bubble is encountered - If RNAP stalls in G rich region, Rho catches up - Causes termination of transcription - Initial binding site is extended ss region = rut - Rho utilization site - ~70 nts, sometimes 80-100 - Rho closes around RNA, advances towards RNAP - Unwinds RNA from template DNA (freeing it) - Causes allosteric changes in RNAP that release template when it catches up . Understand how transcriptional regulators sense cellular conditions to regulate 2 transcription - RNAP recognizes sequence elements -35 and -10 boxes = basic promoter - Efficiency of transcription related to strength of interaction - Always a basal level of transcription (never fully off) - These are points for potential regulation of gene expression - Transcription regulated by repressors and activators - Repressors - Bind to operators that overlap with promoter region to repress transcription - Often within promoter - Activators - Bind sites upstream of the promoter to activate transcription - Bacterial genes are negatively or positively regulated - Involves both induction or repression of expression - Genes under negative control expressed until switched off - Negative control = fail-safe mechanism (if repressor gene disrupted, genes turned on and cell not deprived) - Negative control can be relieved by inducer that prevents repressor from binding operator site - Can be regulated by the presence of a corepressor molecule that promotes binding to operator - Genes under positive control only possible when active regulator protein is present - C an be activated by inducer that promotes binding of activator to DNA, enhancing transcription - Can be repressed by a corepressor that prevents activator from binding DNA, reducing rate of transcription - Bacterial genes arranged in operons - Operon structure allows transcriptional regulation of set of genes functioning in same biochemical pathway - lac operon - Glucose = preferred carbon source of E.coli - Glucose + Lactose in media = glucose metabolized, and lactose unused - Glucose repression / catabolite repression - Prevents wasteful duplication of energy-producing enzyme systems, repressing transcription of lac + other operons - High glucose = low cyclic AMP (cAMP) - Low glucose = high cAMP - Adenylate cyclase inhibited when glucose transported into cell - Lac operon under negative regulation - Lacl = repressor - Controlled by its own promoter (very weak) - Tetramer (~10 tetramers per cell) - Binds operator sequence, interfering w/ transcription - Lacl has three operator binding sites: - One strong operator site (O1 upstream of lacZ) - Situated & overlaps promoter - Two weaker sites (O3 upstream & O2 downstream) - Can bind two operator sequences at once - Creates loop in DNA, preventing transcription - Three functional regions - Helix-turn-helix: recognize + bind operator sequence - Inducer-binding site: bind inducer from site which binds operator DNA - Oligomerization region: involved in quaternary structure of repressor - Binding of inducer changes repressor conformation - No longer binds to operator w/ high affinity - Still good affinity, but it drops with inducer present - Slightly less picky on where it binds - Lac operon is inducible - Induction = synthesis of enzymes in response to specific substrate - Very rapid - Basal expression level = 5 molecules LacZ - Induced expression = ~5000 molecules LacZ - Lac mRNA extremely unstable - 3 minute half-life - Induction rapidly reversible - Transcription stops when inducer removed, mRNA destroyed and cell returns to basal state - Second layer of regulation - Lac promoter relatively weak - CAP protein (catabolite activator) helps activate transcription from promoter (“recruits” RNAP) - Carboxy-terminal domain of RNAP α subunit interacts w/ CAP to stabilize interaction w/ promoter region - CAP regulated by cAMP levels - Yes lactose + No glucose + Yes cAMP = CAP → RNAP - Regulation of Arabinose Operon - Proteins required to catabolize arabinose: AraA, B, D - Regulation: similar to lac - No arabinose = OFF - Arabinose + glucose = OFF - Just Arabinose = ON - AraC regulates araBAD operon - araC gene transcribed from pc promoter - araC and araBAD genes in opposite orientations - 4 potential binding sites for AraC (O2, O1, I1, I2) - Binding sites same sequence, but spaced apart - araO1 is compound, contains araO1L and araO1R - Similar to araI12 site - AraC = dimeric repressor - No L-arabinose = dimerization of AraC - Most energetically stable form binds I1 and O2 sites - ~210 bp apart, creates loop in DNA - No RNAP access to pBAD promoter = no transcription - Yes L-arabinose = conformational change in AraC - protein-DNA affinity unchanged - Most energetically stable form binds I1 and I2 sites(next to each other) - R NAP can access pBAD promoter, binds pc promoter but competes with AraC - Yes transcription - AraC autoregulates its transcription - High levels of AraC protein bind araO1 sequence - RNAP cannot access promoter - High AraC = no RNAP on AraC promoter = no more AraC - CAP-cAMP = activator of araBAD operon - AraC absent = transcription of araC - araBAD transcribed at basal level - RNAP not strongly recruited - cAMP + L-arabinose low = AraC repression - No araBAD transcription - cAMP + L-arabinose high = araBAD transcription - AraC in non-loop conformation . Understand how transcription and translation can be coupled to regulate gene 3 expression - Attenuation: Intrinsic terminators - Transcription can terminate at specific termination sites in DNA - RNA is dynamic and can form several alternative secondary structures w/ same primary sequence - Regulation of tryptophan biosynthesis - Need all gene products to make trp (same promoter) - Attenuation = mechanism for post-transcriptional regulation of gene expression - Regulation after it has started (can stop transcription after initiation) - For tryptophan biosynthetic operon, attenuation regulates transcription termination or pausing - Coupling of transcription and translation = transcription linked to metabolic status of cell - Trp operon regulated by TrpR repressor - Tryptophan = corepressor molecule - TrpR binds trp when high conc and binds operator site to down-regulate