Biochemistry 14.3 Chromatography PDF
Document Details
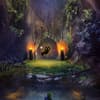
Uploaded by iiScholar
Arizona State University
Tags
Related
- Protein Chromatography Lecture 2 - BMS2043 - Feb 2024 PDF
- Chapter 5 - Protein Purification PDF
- Protein Purification and Characterization - Textbook Chapter PDF
- Protein Purification Principles and Practice PDF
- Principles of Protein Isolation and Purification PDF
- BCH 201 General Biochemistry I Methods of Protein Isolation, Purification, and Identification PDF
Summary
This document provides an overview of chromatography techniques, focusing on their principles and applications in biochemistry. It describes size exclusion chromatography, highlighting its mechanisms and differences from other separation methods like SDS-PAGE.
Full Transcript
# Chromatography ## Introduction Many laboratory techniques in biochemistry separate proteins or other biomolecules from each other for analysis or purification. The techniques discussed so far in this chapter — gel electrophoresis and blotting — are primarily used for analysis of a protein's prop...
# Chromatography ## Introduction Many laboratory techniques in biochemistry separate proteins or other biomolecules from each other for analysis or purification. The techniques discussed so far in this chapter — gel electrophoresis and blotting — are primarily used for analysis of a protein's properties, but are not well suited for purifying large amounts of protein. To study many aspects of a protein, the protein must first be expressed in large amounts in an organism or in cell culture. The cells expressing the protein must then be lysed (ie, broken open) to release the protein of interest. However, these cells also contain many other proteins and biomolecules that are not of interest to the study. These impurities must be removed while retaining the protein of interest. This lesson discusses several chromatography techniques, each of which separates mixtures of proteins and other biomolecules by various physical properties. Chromatography techniques that are commonly used to separate small molecules are discussed in *Organic Chemistry Lesson 13.3*. ## 14.3.01 Principles of Chromatography Like electrophoresis, chromatography is a set of techniques that separate molecules by one or more physical properties. Every chromatography technique includes a stationary phase and a mobile phase. The stationary phase typically consists of small beads or a gel packed within a column. The mobile phase consists of a solution that carries the molecules of interest. The mobile phase is allowed to flow through the stationary phase, and the molecules in the mobile phase are separated based on how strongly they interact with the stationary phase (Figure 14.33). * **Column:** The column contains the stationary phase * **Stationary phase:** The beads or gel that the molecules interact with. * **Mobile phase:** The solution that carries the molecules of interest. **Figure 14.33** Column chromatography separates molecules in the mobile phase. Molecules that interact strongly with the stationary phase move through the column more slowly than molecules that interact poorly with the stationary phase. Therefore, the molecules that interact poorly exit the column (ie, elute) sooner. Some biomolecules have color that can be detected by the human eye, but most are colorless and must be detected by other means. The side chains of tryptophan and tyrosine strongly absorb 280 nm light. Therefore, to detect proteins eluting from a column, a spectrophotometer that detects absorbance of 280 nm light is commonly included as part of the experimental setup. The light source and detector are positioned at the end of the column where the buffer exits, and absorbance can be plotted as a function of time (Figure 14.34). **Figure 14.34** Detection of protein in several aliquots collected from a column using 280 nm light. Small volumes (aliquots) of the buffer exiting the column are collected, and those with strong absorbance at 280 nm (A280) most likely contain protein. The measurement of A280 does not show whether the protein in a given aliquot is the specific protein of interest, however. Each aliquot that contains protein can subsequently be tested for the presence of the protein of interest by assays that detect specific protein activity (eg, enzymatic function, ligand binding). Importantly, none of the chromatography techniques described in this lesson are perfect. All allow some impurities to be included with the protein of interest. To increase the purity of a sample, several chromatography techniques may be used in succession to separate proteins based on different properties. ## 14.3.02 Size Exclusion Chromatography Size-exclusion chromatography (SEC), also called gel filtration chromatography, uses beads that contain pores through which some of the proteins may migrate. In contrast to other forms of chromatography, none of the proteins in a mixture bind to SEC beads. Instead, small proteins enter the pores, whereas large proteins cannot and are excluded from the pores. In this way, small proteins have more interactions with the beads. Different beads have different pore sizes, or cutoffs, that accommodate different sizes of proteins. Because proteins move through an SEC column at different rates depending on whether and to what extent they can enter the pores, SEC separates proteins by their physical size. In this way SEC is similar to SDS-PAGE (see Lesson 14.1), but it differs in several important ways. * **First and most importantly, the correlation between size and rate of migration in SEC is the opposite of the correlation in SDS-PAGE.** Large proteins move through SEC columns more quickly than small proteins, so large proteins elute first. In contrast, large proteins move more slowly in electrophoresis. **Figure 14.35** Large proteins do not fit in the pores in a size-exclusion chromatography column and travel a shorter path than small proteins. **The reason for this difference is that whereas electrophoresis forces all proteins to move through the pores, in SEC only sufficiently small proteins will do so.** Proteins that are too large to fit inside the pores simply do not enter them, and instead traverse only the portions of the column not occupied by beads, known as the void volume, and so travel a shorter path. Small proteins that can enter all the pores must cross more volume (the total volume) and travel a longer path. Because large proteins travel a shorter path, they elute more quickly. Separation of large and small molecules by SEC is shown in Figure 14.35. **Figure 14.36** Separation of proteins of various sizes by size-exclusion chromatography. **Most proteins resolved by an SEC column are of intermediate size: small enough to enter some (but not all) pores, and large enough that they only partially enter them.** These proteins travel at an intermediate rate depending on their size, which allows SEC to provide resolution of a range of proteins from small to medium to large, as shown in Figure 14.36. However, proteins that differ in size by only a few amino acids are unlikely to be separated well by SEC. **The second important way in which SEC and electrophoresis differ is the force that causes proteins to migrate.** Electrophoresis uses an electric current to force proteins through a gel matrix. In contrast, SEC relies only on gravity or, in some cases, the action of a pump to move the mobile phase carrying the proteins through the column. All proteins in an SEC column migrate in the same direction, regardless of their charge. Therefore, proteins of the same size move through the column at the same overall rate, even if their charges differ. **Finally, SEC does not typically denature the proteins in the mixture.** Denaturants and reducing agents may be added for specialized purposes, but they are generally not necessary. Therefore, the proteins in the column usually retain their secondary, tertiary, and quaternary structures. This allows proteins collected by SEC to remain functional, which allows for further study. ## 14.3.03 Ion-Exchange Chromatography Most proteins are electrically charged (ie, they are ions) at physiological pH. **Ion-exchange chromatography exploits this fact by using electrically charged beads as a stationary phase.** Positively charged proteins bind to negatively charged beads, and vice versa. Bound proteins can then be made to unbind by the gradual addition of salt to the mobile phase. * **When cationic (positive) proteins are bound to negatively charged beads, the addition of salt provides new cations that compete with the protein for binding.** When the salt concentration is sufficiently high, the cations in the salt outcompete the protein and the protein elutes. Therefore, the column exchanges cationic proteins for cations from the added salt, and this form of chromatography is called *cation-exchange chromatography*. * **The same principle applies to anion-exchange chromatography, but anionic proteins bind to positively charged beads and exchange with anions from the salt.** Any protein with an *isoelectric point (pl)* below the pH of the buffer has a net negative charge, and any protein with a *pl* above the pH of the buffer has a net positive charge. **A larger difference between pH and *pl* yields a greater charge magnitude.** For example, at pH 7 a protein with a *pl* of 2 has a greater negative charge than a protein with a *pl* of 4, and a protein with a *pl* of 10 has a larger positive charge than a protein with a *pl* of 8. **A protein with a large magnitude charge can bind to an ion-exchange column more tightly than a protein with a smaller magnitude charge but of a similar size.** Therefore, a greater salt concentration is required to compete with highly charged proteins. Accordingly, proteins with small charges elute at lower salt concentrations than proteins with large charges, and the order of elution can be predicted from the isoelectric points of each protein in a mixture. **Figure 14.37 depicts both cation- and anion-exchange chromatography.** **Figure 14.37** General procedures for cation- and anion-exchange chromatography. **Uncharged proteins do not bind the column and elute even with no salt added.** Proteins with the same charge as the beads are repelled by the beads and exit the column even more quickly than uncharged proteins. These proteins are said to be "sped on" by the column. ## 14.3.04 Affinity Chromatography In affinity chromatography, the beads in the column are linked to chemical groups that bind only certain proteins (or other molecules of interest) with high specificity. In many commonly used setups, the molecules attached to the beads are antibodies. Other molecules may also be used. Any molecule that does not specifically bind is washed out of the column, while molecules that do bind the beads are retained. Various methods may be used to elute the protein of interest. Each method involves adding a new buffer to the column that alters the environment and, consequently, the binding interactions. Changes in salt concentration or pH may alter binding interactions between an antibody and a protein, for example, allowing the protein to unbind and exit the column. Alternatively, another ligand that acts as a *competitive inhibitor* of the binding interaction may be added to the mobile phase to disrupt the protein-bead interaction. **The steps of affinity chromatography are shown in Figure 14.40.** **Figure 14.40** The steps of affinity chromatography. **Affinity chromatography is highly advantageous because it can select for a specific protein of interest while eliminating other proteins in a single step.** In contrast, size-exclusion chromatography cannot separate the protein of interest from other proteins of similar size, and ion-exchange chromatography cannot separate the protein of interest from other proteins with the same charge. Therefore, when possible, affinity chromatography is desirable. However, each specific protein that a researcher may wish to purify requires a column with unique beads. Whereas a single SEC column or a single ion-exchange chromatography column can be used to help isolate any protein of interest, an affinity chromatography column with beads that bind a certain protein can only be used to purify that protein. Producing different affinity columns for every possible protein of interest is not practical. To solve this problem, many proteins of interest are expressed recombinantly (see Biology Chapter 4) with extra amino acids added to either the N- or C-terminus. These additions are typically referred to as "tags." Common tags include the Myc tag and polyhistidine tags. Myc tags (and other similar tags) are each targets of specific antibodies (Figure 14.41), and beads with antibodies that bind these tags are sold commercially. Myc-tagged proteins can be eluted by the addition of short peptides expressing the myc sequence. Similarly, the imidazole side chain of histidine binds tightly to nickel, and columns with beads that contain nickel are also sold commercially. Polyhistidine tags are eluted by addition of free imidazole. **Figure 14.41** A protein may be tagged with an extra amino acid sequence that can be bound by specific antibodies. These affinity columns can separate any protein that has the appropriate tag from all proteins that do not. However, the addition of the tag may alter the properties of the protein itself. Consequently, proteins purified in this way must have their tags removed after purification or control experiments must be performed to show that the tag does not interfere with or alter protein function. Another important aspect of affinity chromatography is that a protein of interest may bind to other proteins. If the protein that binds to the beads also binds to another protein, both proteins are retained by the column until elution occurs. This fact may be used to determine which proteins in a system interact with each other. To look for binding interactions, a variation of affinity chromatography called *co-immunoprecipitation (coIP)* may be used. In this method, the beads are commonly not packed into a column but are instead placed in the bottom of a test tube. The protein mixture is allowed to interact with the beads, during which time antigenic proteins interact and bind with the antibodies on the beads. By binding to the beads, the antigenic proteins (ie, proteins that bind the antibody) fall out of the bulk solution (immunoprecipitation). **Proteins that bind the protein of interest but do not directly bind the antibody are said to have *co-immunoprecipitated*.** The overlaying solution (supernatant) is removed, the beads are washed several times to remove impurities, and the remaining, bound proteins are then eluted. Any proteins that bound the tagged, antigenic protein remain in the tube and can be detected by western blot or other techniques (Figure 14.42). **Co-immunoprecipitation often helps identify various proteins that participate in a complex**