Biocehm 7.2 Complex Carbohydrates PDF
Document Details
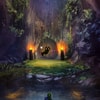
Uploaded by iiScholar
Arizona State University
Tags
Summary
This document provides an introduction to complex carbohydrates, their structures, and properties. It explores glycosidic bonds and their formation between monosaccharides and other molecules. The document also includes figures and concept checks related to the topic.
Full Transcript
# Complex Carbohydrates ## Introduction Lesson 7.1 discussed various biologically important monosaccharides and the systems used to classify them. Just as amino acids combine to form polypeptides and proteins, monosaccharides can combine to form more complex carbohydrates. The linkage between two...
# Complex Carbohydrates ## Introduction Lesson 7.1 discussed various biologically important monosaccharides and the systems used to classify them. Just as amino acids combine to form polypeptides and proteins, monosaccharides can combine to form more complex carbohydrates. The linkage between two monosaccharides is called a glycosidic bond (or a glycosidic linkage). Monosaccharides can combine through glycosidic bonds to form disaccharides, trisaccharides, and longer chains, including highly complex polysaccharides with multiple branch points. Polysaccharides are also commonly known as glycan chains. In addition to forming glycosidic bonds with other carbohydrates, a carbohydrate may form a glycosidic bond with another biomolecule such as a protein, a nitrogenous base, or a lipid. In this way, many biomolecules may be modified by linkages to complex glycan chains. This lesson explores the ways in which monosaccharides can form glycosidic bonds and the important structures formed by these linkages. ## 7.2.01 Glycosidic Bonds A glycosidic bond is a chemical linkage between the **anomeric carbon** of a carbohydrate and a nucleophilic atom of another molecule. Often the other molecule is another carbohydrate but it may also be a protein, a lipid, or the nitrogenous base of a nucleoside. This concept covers several types of glycosidic bonds and their classification. ### Glycosidic Bonds Between Two Carbohydrates Two monosaccharides form a glycosidic bond and become a disaccharide when the hemiacetal or hemiketal carbon on one monosaccharide condenses with a hydroxyl group on the other, as shown in Figure 7.26. Formation of the glycosidic bond releases water and therefore constitutes a condensation reaction, which can be reversed by hydrolysis. Just as amino acids become amino acid residues upon peptide bond formation (see Lesson 2.1), monosaccharides become monosaccharide residues (also called monosaccharide units) when glycosidic bonds form. ![Figure 7.26] An example of glycosidic bond formation between two monosaccharides to form a disaccharide. A disaccharide can become a trisaccharide by combining with a third monosaccharide. This process may be repeated to form carbohydrates consisting of many monosaccharide residues. These complex carbohydrates are known as oligosaccharides (meaning "a few sugars"), polysaccharides (meaning "many sugars"), or simply as glycans. Due to the loss of water, carbohydrates consisting of more than one monosaccharide residue do not have the general formula (CH2O)n. Instead, disaccharides have the formula Cn(H2O)n-1, trisaccharides have the formula Cn(H2O)n-2, and so on. Figure 7.27 shows structures of carbohydrates that follow these molecular formulas. ![Figure 7.27] Examples of changes in the general molecular formula when glycosidic bonds form. **Concept Check 7.7** What is the molecular formula of a tetrasaccharide formed from one glucose molecule (C6H12O6), one ribose molecule (C5H10O5), one erythrose molecule (C4H8O4), and one fructose molecule (C6H12O6)? **Solution** Glycosidic bond formation converts the hemiacetal (of a cyclic aldose) or the hemiketal (of a cyclic ketose) into an acetal or a ketal, respectively. The acetal or ketal can no longer open to linearize or allow for mutarotation. Therefore, once a monosaccharide unit has contributed its anomeric carbon to a glycosidic bond, it is locked in cyclic form and has a fixed anomeric configuration (see Figure 7.28). ![Figure 7.28] Monosaccharide units that contain an acetal (or ketal) group because of a glycosidic bond are locked in a cyclic form. Unlike amino acids, which generally form linear polymers, glycans often have branches. For instance, a single aldohexose unit may be linked to one carbohydrate through its anomeric carbon (carbon 1), to another carbohydrate through carbon 4, and to yet another through carbon 6. Each of these monosaccharide units may also have branches, resulting in highly complex structures. Figure 7.29 shows an example. ![Figure 7.29] A single monosaccharide unit may participate in multiple glycosidic bonds, forming complex branched structures. To distinguish glycosidic bonds at different positions within a monosaccharide unit, these are classified based on the positions of each carbon and the stereochemistry (a or ẞ) of any anomeric carbons involved. For example, if the anomeric carbon of an aldose (ie, carbon 1) is in the a configuration and linked to carbon 4 of another monosaccharide unit, the linkage is classified as an a-1,4-glycosidic bond. Similarly, a ketose linked through a ẞ-anomeric carbon (carbon 2) to carbon 6 of another monosaccharide has a ẞ-2,6-glycosidic linkage. Figure 7.30 shows examples of different glycosidic linkages. ![Figure 7.30] Examples of a-1,4-glycosidic and ẞ-2,6-glycosidic linkages. **Concept Check 7.8** Characterize each of the glycosidic linkages in the complex carbohydrate shown. All monosaccharide units are D-sugars displayed in a standard Haworth projection. ## Reducing and Nonreducing Sugars To form a glycosidic bond, one of the carbohydrates involved must have a free anomeric carbon (ie, an anomeric carbon not involved in a glycosidic bond). Historically, carbohydrates with free anomeric carbons have been classified as **reducing sugars** because they can reduce silver ions (Ag+) to silver metal. The reaction that produces this effect is called the Tollens test. The phrase "reducing sugar" is a misnomer in some ways because any primary or secondary alcohol group can potentially act as a reducing agent. However, when the phrase was first introduced, it referred only to the ability of carbohydrates to reduce Ag+, which carbohydrates can accomplish only through an aldehyde group. All free monosaccharides are reducing sugars. For aldoses, reduction occurs when the aldehyde group of a linear monosaccharide donates electrons to the silver ions, and the aldehyde becomes a carboxylic acid. The ketone group in a ketose cannot be oxidized any further, but ketoses can act as reducing sugars because under the Tollens test reaction conditions they can tautomerize, which converts them to aldoses. Carbohydrates with no free anomeric carbon are classified as **nonreducing sugars**. Trehalose (shown in Figure 7.31) is an example of a nonreducing sugar because both anomeric carbons are involved in the glycosidic bond, so neither is free. Note that to align the anomeric carbons, the Haworth projection of one of the glucose units is rotated 180 degrees. ![Figure 7.31] Structure of trehalose, a nonreducing sugar. Because nonreducing sugars have a glycosidic bond involving two anomeric carbons, characterization of the glycosidic bond requires indicating the configurations of both. For instance, in the case of trehalose, the glycosidic bond is an a-1,a-1 linkage. Reducing and nonreducing terminology can also be used to describe the polarity of complex sugars. Just as peptides have N-terminal and C-terminal ends, complex carbohydrates have **reducing** and **nonreducing ends**. The **reducing end** of a complex carbohydrate is the end that terminates with an anomeric carbon. The other end, with no free anomeric carbons, is the nonreducing end. Figure 7.32 shows the reducing and nonreducing ends of carbohydrates. Each monomer within a complex carbohydrate also has reducing and nonreducing ends. ![Figure 7.32] Nonreducing and reducing ends of complex carbohydrates. (A) The reducing end of the chain can open and reduce Ag+. (B) Reducing and nonreducing ends of a monosaccharide unit. For two carbohydrates to link through a glycosidic bond, at least one of them must have a free reducing end. However, note that glycosidic bond formation is a condensation reaction and not a redox reaction. Consequently, although ketoses must tautomerize to aldose form to give a positive Tollens test (which involves a redox reaction), they do not need to tautomerize to form glycosidic bonds. **Concept Check 7.9** Classify the carbohydrate shown as reducing or nonreducing. ## Glycosidic Bonds Between a Carbohydrate and a Noncarbohydrate In addition to glycosidic linkages between two carbohydrates, a glycosidic bond can also form between the reducing end of one carbohydrate and the nucleophile in another molecule, creating a molecule known as a glycoside. Some important examples include the glycosylation of proteins discussed in Lesson 2.4, the formation of a bond between ribose and a nitrogenous base (see Chapter 8), and the formation of a bond between a carbohydrate and a lipid (see Chapter 9). In some cases, the anomeric carbon in these glycosides is bound to one oxygen and one nitrogen instead of two oxygens. Examples are shown in Figure 7.33. ![Figure 7.33] Examples of glycosidic linkages between carbohydrates and noncarbohydrates. Protein glycosylation yields glycoproteins, which facilitate many interactions between cells. In addition, viruses and other pathogens may bind to glycoproteins in the first step of cell infection. Lipid glycosylation yields glycolipids which, like glycoproteins, are commonly found on the extracellular side of cell membranes. ABO blood types stem from different carbohydrates linked to lipids in red blood cell membranes. Defects in glycolipid metabolism can lead to various disease states such as Tay-Sachs disease. Nucleoside synthesis results in a glycosidic bond between the anomeric carbon of ribose (or deoxyribose) and one of the nitrogenous bases found in DNA or RNA. ## 7.2.02 Disaccharides Disaccharides are the smallest, simplest type of complex carbohydrate. They consist of two monosaccharide units linked to each other through a single glycosidic bond. Several disaccharides play biologically important roles, but for the exam, the most important disaccharides are maltose, sucrose, and lactose. Maltose is a disaccharide consisting of two glucose units linked by an a-1,4-glycosidic bond. Maltose is derived from the digestion of starch (see Concept 7.2.03) and can be further digested to yield individual glucose units. The linkage between the glucose units is critical. When the linkage is changed to a ẞ-1,4-glycosidic bond, the sugar becomes cellobiose. Although cellobiose is identical to maltose in every way except the orientation of the linkage, animals can digest maltose but they lack the enzymes needed to digest cellobiose. Maltose and cellobiose are shown in Figure 7.34. ![Figure 7.34] Maltose and cellobiose differ only by the configuration of the glycosidic linkage. Sucrose is a nonreducing sugar consisting of one glucose unit and one fructose unit linked to each other through their anomeric carbons. The glucose unit is in the a configuration, and the fructose unit is in the ẞ configuration. In addition, glucose is in the pyranose form, whereas fructose is in the furanose form. Because the anomeric carbon of glucose is carbon 1 and that of fructose is carbon 2, the glycosidic linkage in sucrose is an a-1,ẞ-2-glycosidic bond. Sucrose can be digested to its fructose and glucose components, which can then be metabolized for energy or other purposes. Because the anomeric carbons of each monosaccharide in sucrose link to each other, the Haworth projection of one of the monosaccharides (usually fructose) must be flipped along its vertical axis to align the anomeric carbons. Every group that pointed up in the typical depiction points down, and vice versa, in the flipped depiction. The structures of fructose, glucose, and sucrose are shown in Figure 7.35. ![Figure 7.35] Structure of sucrose, assembled from glucose and fructose. Lactose consists of a galactose unit linked through its anomeric carbon to carbon 4 of a glucose unit. The galactose unit is in the ẞ configuration, and therefore the linkage is a ẞ-1,4-glycosidic bond. Consequently, lactose is identical to cellobiose except for the orientation of carbon 4 at the nonreducing end. Although humans do not have enzymes that break down cellobiose, most humans can digest the highly similar lactose molecule using the enzyme lactase. This fact illustrates the specificity of some enzymes: a change in the orientation of a single chiral center can significantly impact interactions between enzymes and substrates. Individuals with lactase deficiency may experience lactose intolerance, which can be treated with lactase enzyme supplements. The structure of lactose is shown in Figure 7.36. ![Figure 7.36] Structure of lactose. **Concept Check 7.10** Which monosaccharide unit in lactose is the reducing end? ## 7.2.03 Polysaccharides Polysaccharides are carbohydrates that consist of multiple monosaccharides. All eukaryotes and many prokaryotes use polysaccharides both for structure and energy storage. Storing carbohydrates in this way decreases the glucose molarity (and therefore total cellular osmolarity) within the cell as several small glucose molecules become one much larger molecule. Because polysaccharides can include branch points, the diversity of ways to assemble a polysaccharide with a given number of monosaccharide units can be enormous. For example, accounting for all possible positions, anomeric carbon orientations (a or ẞ), and ring forms (furanose or pyranose), just two glucose units can link to each other in at least 30 different ways. The number of possible linkages increases exponentially as more monosaccharide units are included. Consequently, polysaccharides can perform a wide variety of functions, many of which are not currently understood. General features of a few important polysaccharides should be remembered for the exam. ### Starch Starches are polysaccharides consisting entirely of glucose units. The simplest starch is amylose, in which all glucose units are linked to each other through a-1,4-glycosidic bonds in a linear chain. These chains can be broken down into maltose units, which can be further degraded to their glucose constituents. Amylose is a major component of many staple foods and an important part of human diets. Note that amylose does not have a specific number of glucose units. Instead, amylose is any linear chain of a-1,4-linked glucose units, and different amylose molecules can differ in size. Figure 7.37 shows the structure of amylose. ![Figure 7.37] Structure of amylose, the simplest form of starch. Plants also contain a form of starch called amylopectin (see Figure 7.38). Like amylose, amylopectin contains glucose units in a-1,4-glycosidic linkages; however, amylopectin also contains branch points that involve a-1,6-glycosidic linkages. On average, amylopectin contains a branch point approximately every 25 glucose units. Animals can digest amylopectin into glucose units. ![Figure 7.38] General structure of amylopectin. ### Glycogen Glycogen is the primary form of glucose storage in animals. Like amylopectin, glycogen consists of glucose units linked by a-1,4-glycosidic bonds within linear chains and a-1,6-glycosidic bonds at branch points. The most notable difference between amylopectin and glycogen is that glycogen is more branched, with branch points approximately every 10 to 15 glucose units (Figure 7.39). In addition, glycogen chains in living cells are linked to the protein glycogenin by a glycosidic bond to a tyrosine residue. Therefore, glycogen does not have a free reducing end, whereas amylopectin does. Note that due to branching, both molecules have multiple nonreducing ends. Having multiple nonreducing ends facilitates adding glucose units to the polymer (as in glycogenesis; see Concept 11.4.01) or removing glucose units from the polymer (as in glycogenolysis; see Concept 11.4.02). ![Figure 7.39] Comparison of amylopectin and glycogen. ### Cellulose Cellulose is related to amylose in the same way that cellobiose is related to maltose: they are identical in every way except the orientations of the glycosidic bonds. Cellulose is a linear chain of glucose units linked by ẞ-1,4-glycosidic bonds and is a major component of plant cell walls. Because animals lack enzymes that hydrolyze ẞ-1,4-glycosidic bonds between glucose units, they cannot digest cellulose. Animals that rely on diets high in cellulose (eg, ruminants) require a symbiotic relationship with certain microflora (eg, bacteria), which do have the necessary enzymes, to digest their food. The glucose units in cellulose are linked by ẞ-1,4-glycosidic bonds; as such, the bond from each anomeric carbon to the oxygen of the glycosidic bond might be expected to point upward in a Haworth projection. Similarly, the bond from carbon 4 to the glycosidic bond oxygen would be expected to be shown pointing downward. However, connecting the anomeric carbon of one unit to carbon 4 of another without altering the Haworth projection would lead to visual depiction of a chain of monosaccharides moving diagonally up the page. To convert the projection to display a horizontal chain, it is convenient to draw cellulose by flipping the Haworth projection of every other glucose unit in the chain (see Figure 7.40). ![Figure 7.40] Two depictions of cellulose. Flipping the Haworth projection of every other glucose unit facilitates a horizontal depiction. ### Other Polysaccharides Although other polysaccharides do not need to be memorized, it is important to be aware of them. These polysaccharides primarily include the glycan chains found on glycoproteins and glycolipids. They commonly consist of several types of monosaccharide units, including various sugar derivatives, and often contain multiple branch points with various types of glycosidic linkages. An example of such a polysaccharide is shown in Figure 7.41. Note that this molecule serves only to illustrate the complexity of carbohydrates and need not be memorized. ![Figure 7.41] A typical glycan found on the proteins of many human cells. This glycan includes N-acetyl derivatives, an L-deoxysugar, and a carboxylic acid derivative.