BIOB10 Notes - PDF
Document Details
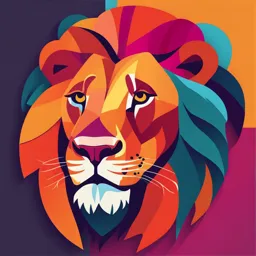
Uploaded by UnbeatableRecorder
University of Toronto Scarborough
Tags
Summary
These lecture notes cover cell biology, discussing the roles of different types of cells, and the process of cell division. Topics include the history of the discovery of cells, and cellular processes.
Full Transcript
Sep. 5 - Lecture 1 Why Study Cell Biology? - Professions: - It has very logical implications if one is interested in any health-related professions, in biotechnology, in understanding patent law; all of this requires an understanding of cell biology. - Society:...
Sep. 5 - Lecture 1 Why Study Cell Biology? - Professions: - It has very logical implications if one is interested in any health-related professions, in biotechnology, in understanding patent law; all of this requires an understanding of cell biology. - Society: - If one wants to make any informed decisions at all as a member of a city, country, etc. one must make decisions about whether their family consumes genetically modified plants, whether one will support the taxpayer dollars going towards lab-grown organs or meat, whether human genome editing is something that should be regulated, (and if so, how? And who should be able to get this privilege?), and why we should fund basic science and the point of it. Discovering Cells - Robert Hooke (1665) - Hooke looked at dried bark, (cork tree for example); cork is just dried cells, dehydrated tissue. He looked at it and asked, “What is this? What is this bark actually made of?”. Hooke came up with “cells”, after looking at cork tissue through his microscope, he saw a parallel between the cork tissue and the little rooms in monasteries, (where archways demarcated people, the little rooms were called cells). - Anton van Leeuwenhoek (1674) - Leeuwenhoek looked at pond water under a microscope, and within it, he saw things moving back and forth, which he called “animalcules”. He thought that if only he could make a better microscope he would bet on the fact that the animalcule was made of cells. - He also did an interesting experiment, where he swabbed his mouth, took out whatever product/extract there was, put that under a microscope, looked at it, and noticed lots of bacteria. There were tons of bacteria and was able to think about that maybe those cells are things that can move when they’re alive. - Bacteria at the time, were not well understood, but he thought that they could be single-celled organisms. - What can we really say about cells? - Matthias Schleiden (1838), Theodor Schwann (1839), and Rudolf Virchow (1855) proposed the Cell Theory: - 1. All organisms are made up of one or more cells - 2. Cells are the structural unit of life - 3. Cells can only arise from pre-existing cells - This info was made because they wanted to communicate it broadly in society. They wanted people, on average, who weren’t studying science to care. Universal Features of Cells - When people thought about cells a long time ago, there weren’t a lot of people working on them, they were more interested in what the commonalities were; if we take all cells and want to make a list of what all cells do, then there must be several foundational principles. If we understand what those foundational concepts are, then it can explain all of life. - Cells possess a genetic program and the means to use it. - Cells have a genetic program and that genetic program (in this case, DNA), has a hereditary material, which they then pass on to daughter cells when they divide. - Using that genetic program, they can then convert that information into other macromolecules, (in this case proteins), which then drive the functions of that particular cell. - Cells are capable of producing more of themselves. - That templated place of genetic information is important; it moves between cells from a parent cell to a daughter cell, and it can move in many different ways, (there are many different ways and steps that cell division can happen). - The whole point is that there is this original, templated material, and it can be split into 2 cells as an identical copy of the original, by creating new strands that are complementary to the old strands. Referred to as templated polymerization. - A long polymer made of individual subunits, where the template is prescribed by the original molecule found in the mother cell. - This templated replication is a foundational principle of all cells. - Cells carry out chemical reactions. - When we get to a daughter cell, and we have that identical information, we can use it now and produce what’s most important for that cell to live; to carry out all the chemical reactions and to catalyze those chemical reactions. For that, we must make proteins, (proteins are typically catalysts). - A subset of proteins that perform these catalytic reactions are referred to as enzymes. Enzymes function now in detailed processes that we know as cellular metabolic processes, which often require energy (ATP) to be able to do their job in the cells. - Example: Lysosomes identify their substrate and be able to cut it up. Lysosomes work on polysaccharides that are found on the outer cell walls of many bacteria. They are an antimicrobial secretion we have and are found in our saliva and our tears. - They offer an antimicrobial protection and are synthesized within cells and secreted out, and can perform catalytic functions outside of the cell from which they are synthesized. - Cells can acquire and utilize energy - We know that the templated information can be passed on to daughter cells and that the information is used to make proteins, which can be catalyzed. The proteins’ catalytic activity helps us build those polymers that are genetic material. If we want to take those subunits, (nucleotides), and build our DNA we can do that with the help of polymerases (proteins that have catalytic activity). The polymerases can then again make more proteins, (and so forth). - Sort of like an auto-catalytic sustaining system that relies on energy (ATP). In this case, the energy in an animal cell comes from the food that we consume, and that food/energy is used to build the compartment and hold together all of the stable structures within cells. Cells take free energy and make a stable, functioning compartment in which we can carry out all these different activities. - In an animal cell, the food (energy) is created by other cells that use light (photosynthesis), which is an important contributor to the way that we will eventually access/acquire the energy that we need in an animal cell. They all require ATP as the carrier of that free energy. - All cells are enclosed by a membrane - All the mentioned ideas, (hereditary materials, metabolic processes, free energy, organization of catalytic properties of proteins) are contained in a membrane. The membrane regulates everything that goes in and out of the cell: a semi-permeable barrier. - Cells respond to stimuli, carry out mechanical activities, and move! - We think about cells as if they just think internally (doing their thing, looking at their DNA, making proteins, keeping themselves alive). - However, we often don’t think that cells are very motile within our bodies, which are essential to our health. These cells respond to stimuli, carry out mechanical activities, and can move. - Cells have lots of cell-surface proteins called receptors and use those receptors to detect molecules in their environment which will then help them to move in a particular direction. Directional motility is another feature of cells and is typically universal. - Example: The neutrophil, a white blood cell, uses the receptors on its surface to detect a small bacterial invader that is present within the bloodstream. It uses the receptors to detect that there is a different structural protein in the bloodstream. It then follows the bacterium until it can engulf it using a process called phagocytosis, then digests it within a compartment called the lysosome. Random Thermal Motion (A Part of Universal Feature #6) - A lot of the processes that cells undergo are caused by random motion. Random thermal motion has so much to play in this context of a cell that seems structured and regulated. - Let’s say that this is the moving cell (for example, the neutrophil that was moving with its membrane moving forward towards the bacterium), it has to push that membrane forward somehow; these are caused by actin filaments, which are part of our cytoskeleton. As we build longer and longer filaments, it will push that plasma membrane in the direction that it wants to go out; however, their movement isn’t perfect. - How would it be possible to add a new actin filament to make it push the membrane forward? The answer is random Brownian thermal motion; for a moment, the plasma membrane has a bit of give. With the thermal movement, it will move a little. As it moves, by chance, (because the cell is a crowded place), another subunit of actin is able to add on to the end of that filament. When it comes back to its resting place, it won’t come back to where it was because it’s got another subunit in the way, and therefore the membrane moves forward. The same thing will happen yet again, where there’s more random thermal motion, another subunit is added, and the membrane moves forward. - Random Brownian motion has a lot to do with how cells work. Cells Differ in Many Ways - There are different types of cells, (and we’ve alluded to this in terms of their energy and where they get it from, whether it’s light or other organisms). We know that this free energy can be harvested by cells in different ways: - Obtain free energy in different ways - Phototrophic cells (sunlight) - Lithotrophic cells (inorganic chemicals in the environment, for example, sulfur) - Organotrophic cells (rely on organic chemicals; not possible unless we have phototrophic or lithotrophic cells). - Some cells specialize in fixing nitrogen and carbon dioxide and others rely on such cells/organisms - When we look at different cells, some cells can specialize in how they can function and produce this free energy. - Example: Plants fix carbon dioxide. However, some plants and animals require the help of nitrogen-fixing bacteria to help the plants fix their nitrogen in addition to their carbon dioxide. - We know that the nature of the free energy source often defines these specialized cells. The Tree of Life: Prokaryotes and Eukaryotes - We’ve drawn up a tree of life since we want to know all the different types of cells and the niches in our entire planet. - We can see that just by looking at the tree of life, there’s so much bacteria, too much to the point where we’ve run out of names for them. This is because they can inhabit almost every type of ecosystem niche that we can imagine on Earth. - Less popular are the archaea, and the least diverse are the eukaryotes. - Even though we talk a lot about eukaryotes and relate a lot of these functions to eukaryotic cell function, realistically, we’re only talking a tiny bit of the diversity of life, when we should be talking more about archaea and bacteria to understand all of the different ways in which cells contribute in terms of their ability to withstand environments. - Genome Diversification and the Tree of Life - Often, we are interested in what is common; this is because when something is common across three domains of life, it’s probably essential. It’s something that’s at the core of being a cell, and if manipulated or mutated, that’s no longer able to sustain life. - Therefore, we often look at those commonalities, and we know that there are at least 200 gene families that are common across all 3 domains of life. - However, if we look at the actual genomes, we know that they look different across the 3 different domains. Many of them are generated by gene duplication: When we have DNA replication and we’ve got a length of sequence that defines a particular gene, if you duplicate that region of DNA, you now end up with 2 copies of that gene. Over time, that sequence can mutate, and now you have sets of families of related genes that used to be just one gene that copied twice, but over time they were copied multiple times and mutations were selectively added to each of them, then they each become their own genes that are just a little different from each other. - That’s how gene families come to a rise, and the function of those genes can often be deduced just by looking at the DNA sequence. DNA sequencing has become easy and inexpensive in the last few decades and so we cannot sequence all of the bacteria around us fast enough. - We can compare genomes across the different domains, and we’ve learned something by looking at their genomes and looking at the morphology and structure of those different cells to learn some details about how those cells differ in those 3 domains. - Prokaryotes - Prokaryotes translate to “before nucleus” - Pro meaning “before” - Karyon means “nucleus” - All bacteria fit into this category - Example: Vibrio cholerae is a bacterium that causes severe intestinal distress in individuals who contract it, (a waterborne disease). It has a flagellum that allows it to move very quickly in aqueous environments. It has many characteristics that we would normally associate with prokaryotes. - Unique characteristics of prokaryotes: - It has a nucleoid, (its genetic material/nucleic acid is in a zone that is not necessarily held together by a membrane) - Is typically structurally simpler than eukaryotic cells - Mostly have less DNA than eukaryotic cells (whatever DNA they have, it tends to house it in a single circular chromosome) - They don’t perform mitosis or meiosis often (they instead perform their type of cell division called binary fission; cells expand and get bigger and eventually just split apart). - Two types of prokaryotes: - Archaea - Almost every extremophile is classified as an archaea and not a bacteria. These are thermophiles that can grow at very high temperatures, around 80-105 C°. - Example: Strain 121 can thrive at temperatures of around 121 C°. This temperature is important because that is the temperature at which we sterilize surgical equipment. It’s a very good thing that we don’t have any archaea that are parasites or pathogens of humans because if they ever mutate and decide to be harmful to us, it can be detrimental to human health. - Archaea are closer to eukaryotes than bacteria are in the tree of life. - Bacteria - Bacteria occupy many different niches. - Example: Cyanobacteria are very complex bacteria because they share these photosynthetic membranes with higher plants, and they’re incredibly organized within a single-celled context to be able to harvest light energy. - Types of prokaryotes: - Prokaryotes come in different shapes, they have lots of genetic information where they can transfer DNA between each other, and between various types of cells (horizontal transfer is possible). Some of them are usually tiny, but there can also be large ones where they are up to 7 mm in length. - Smallest cells: ex. Mycoplasma, Spiroplasma - Spherical cells: ex. Streptococcus - Rod-shaped cells: ex. Escherichia coli, Salmonella - Spiral cells: ex. Treponema pallidum - Eukaryotes - Eukaryote translates to “true nucleus” - Eu means “true” - Karyon means “nucleus” - Many categories fit into eukaryotes; plants, animals, fungi, and protists - Unique characteristics of eukaryotes: - They have a membrane-bound nucleus; they have a nuclear membrane as well as complex internal organelles, (have compartments within the cytosol that can locate or hide away certain components for the cell). Each of those internal organelles has a different function. - They’re structurally more complex; they rely on a complex cytoskeletal system that not only positions those organelles, but also allows the cell to have some sort of shape and structure, and allows them to be able to carry out movement in response to stimuli. - They have more DNA than prokaryotes, and many of these are in chromosomal structures, (linear forms of DNA, not circular). These often are rich in regulatory DNA. - Example: So if you look at the e. Coli genome, about 10-11% of its genome is considered regulatory; it doesn’t code for protein. But if we look at our genome, about 98+% are regulatory/not protein-coding; we’ve got much more regulatory DNA. - Cell division is by either mitosis or meiosis. - The ribosomes that fill the cytoplasm of a cell have no place for the ribosomes to move at all since it’s a gel-like environment inside a eukaryotic cell, it is very crowded. - Two types of eukaryotes: - Unicellular - Everything that this organism needs to survive is done by one cell. - Example: Protists are a good example of unicellular eukaryotic cells. They can do everything our cells can do but in just one cell. - There are carnivorous single-celled eukaryotes; an example is didinium, which is a carnivorous protist. It hunts organisms called paramecium, (which could be 50x the size of the didinium). The didinium has fringes (called cilia), and the cilia/microtubules beat in unison really quickly, and propel the didinium through the water at a very high speed. They go in a clockwise direction and bounce off of things; when they touch something that’s not food, they go backward but when they touch something that is food, the little tip they have can stun the paramecium until it cannot move. Then, the didinium will phagocytose (create a membrane around the prey and wait until it completely consumes the paramecium). - Multicellular - Different activities are carried out by different types of cells; we have specialized cells that dedicate themselves to certain functions and organize differently within the organism. - There are different types of cells, and therefore cell differentiation exists, which is essential for the multicellular development context. - Example: Neurons are different than neutrophils (type of white blood cell), in the sense that there are size differences, and morphology differences, which are all dedicated to different functions within the same organism. - Similarities between prokaryotes and eukaryotes: - The genetic code of prokaryotes and eukaryotes are almost identical - Most of the metabolic pathways, including how they synthesize free energy (ATP) - Certain structural elements (how we build up the cell membrane is not fundamentally different between prokaryotes and eukaryotes) - The Origin of Eukaryotic Cells - The “Endosymbiont” Theory: - The idea is that there is a combination of 2 cells living together in a symbiotic relationship, except one of the cells is living inside the other one. Hence “endo” meaning inside or within. - They believe that this relationship was developed between an original cell that was anaerobic, and it then established a symbiotic relationship with an aerobic organism, and that aerobic organism eventually just became an internal structure of the larger anaerobic organism. That’s how we originally started the precursor of all eukaryotic cells. - The prokaryote decides to organize its inner membrane in a certain way, and while it’s doing this it also decides to allow in a few other organisms that have aerobic properties that are beneficial to this original organism. Now, you’ve got potentially the start of a chain of cells that would now be called eukaryotes. - The original cell that set on the path toward eukaryotes was an archaea, not a bacteria. We know this by sequencing DNA information to look for similarities. - An archaeon makes an odd set of membrane protrusions go out, and those protrusions can sometimes interact with other bacteria in the area. The bacteria are called ectosymbionts, because they live outside the archaea, make contact with the membrane protrusions, and confer some benefit to the archaea; in turn, they get a