BioMol Notes PDF
Document Details
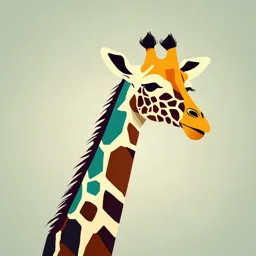
Uploaded by RaptMandolin3405
UiTM
Tags
Summary
This document is a set of notes on molecular biology, covering the history of the field and the discovery of DNA structure. It discusses key figures, experiments, and concepts related to these topics such as the one gene one enzyme hypothesis and the role of histones. The notes also outline the packaging hierarchy of DNA, and discuss the organization of chromatin; including euchromatin and heterochromatin.
Full Transcript
CHAPTER 1 History CHAPTER OBJECTIVES: – Define Molecular Biology – List out works and their relations to chromosome inheritance studies (Genetics) before DNA discovery – Explain how DNA structure is first discovered (the works that helps DNA discovery and the scientists involved) Molecular Biol...
CHAPTER 1 History CHAPTER OBJECTIVES: – Define Molecular Biology – List out works and their relations to chromosome inheritance studies (Genetics) before DNA discovery – Explain how DNA structure is first discovered (the works that helps DNA discovery and the scientists involved) Molecular Biology is the study of gene structure and function at the molecular level - Biological phenomena in molecular terms - Consists of genetics and biochemistry discipline Early works related to Molecular Biology Absence of knowledge on molecular nature of genes Therefore is being known as transmission genetics Studied by Gregor Johann Mendel on pea plants Chromosomes were recognized as a unit that carry genes in the nucleus. In 1910, Thomas Hunt Morgan himself provided evidence for the chromosome theory of inheritance. Morgan worked with the fruit fly (Drosophila melanogaster), a more convenient organism than the garden pea for genetic studies. Barbara McClintock and Harriet Creighton were able to demonstrate recombination in 1931. By examining maize chromosomes microscopically, they could detect recombination between two easily identifiable features of a particular chromosome. Therefore, they established a direct relationship between a region of a chromosome and a gene whenever this physical recombination occurred. Curt Stern observed the same phenomenon in Drosophila. So recombination could be detected both physically and genetically in animals as well as plants. Miescher collected bandages from a nearby clinic and washed off the pus. Friedrich Miescher discovered a mixture of compounds in the cell nucleus- nuclein in 1869. He determined that nuclein was made up of hydrogen, oxygen, nitrogen and phosphorus. There was a unique ratio of phosphorus to nitrogen. He was able to isolate nuclein from other cells and later used salmon sperm (as opposed to pus) as a source. Avery-McLeod A genetic trait (virulence) was transferred from one strain of bacteria to another. It was transferred by mixing dead virulent cells with live avirulent (nonlethal) cells. The substance that caused the transformation from avirulence to virulence in the recipient cells was the gene for virulence, because the recipient cells passed this trait on to their progeny. Beadle & Tatum The one gene, one enzyme hypothesis is the idea that each gene encodes a single enzyme. Sir Archibald Garrod, a British medical doctor, was the first to suggest that genes were connected to enzymes. Beadle and Tatum confirmed Garrod's hypothesis using genetic and biochemical studies of the bread mold Neurospora crassa. Beadle and Tatum identified bread mold mutants that were unable to make specific amino acids. In each one, a mutation had "broken" an enzyme needed to build a certain amino acid. GAMBAR Phoebus Levene Years of work using hydrolysis to break down and analyze yeast nucleic acids, Levene proposed that nucleic acids were composed of a series of nucleotides - each nucleotide was in turn composed of just one of four nitrogen-containing bases, a sugar molecule, and a phosphate group. Levene proposed a tetranucleotide structure, where the nucleotides were always linked in the same order (i.e., G-C-T-A-G-C-T-A ) Erwin Chargaff - Indicated that DNA is symmetrical but failed to make a connection to the DNA structure. - Concluded that the amount of adenine = the amount of thymine, cytosine = guanine Watson & Crick Chargaff's realization that A = T and C = G, combined with X-ray crystallography work by English researchers Rosalind Franklin and Maurice Wilkins, contributed to Watson and Crick's derivation of the three-dimensional, double-helical model for the structure of DNA. Watson and Crick's discovery was also made possible by recent advances in model building, or the assembly of possible three-dimensional structures based upon known molecular distances and bond angles, a technique advanced by American biochemist Linus Pauling. Early Foundations 1. Friedrich Miescher (1869) ○ Miescher, a Swiss physician, discovered a substance he called "nuclein" (now known as DNA) in the nuclei of white blood cells. He identified it as a unique molecule containing phosphorus but did not determine its role. 2. Phoebus Levene (1909–1929) ○ A Russian-American biochemist, Levene identified the components of DNA: the sugar (deoxyribose), phosphate group, and four nitrogenous bases (adenine, thymine, cytosine, guanine). However, he mistakenly proposed that DNA was a simple, repetitive structure called a "tetranucleotide," which downplayed its potential as the genetic material. 3. Oswald Avery, Colin MacLeod, and Maclyn McCarty (1944) ○ These researchers demonstrated that DNA, not proteins, is the carrier of genetic information. They showed that DNA extracted from one strain of bacteria could transfer genetic traits to another—a process known as transformation. Structural Studies of DNA 4. Erwin Chargaff (1950) ○ Chargaff, an Austrian-American biochemist, established two key rules: The amount of adenine (A) is equal to thymine (T), and cytosine (C) is equal to guanine (G) in DNA (Chargaff's Rule). The proportion of A, T, C, and G varies among species, hinting at DNA's complexity and its potential as the genetic material. 5. Maurice Wilkins and Rosalind Franklin (1951–1953) ○ Working at King's College London, Franklin and Wilkins used X-ray diffraction techniques to study DNA. ○ Franklin's famous "Photo 51" revealed DNA's helical structure and provided critical data on its dimensions. ○ Wilkins shared this data with James Watson and Francis Crick without Franklin's explicit permission. 6. Linus Pauling (1953) ○ A Nobel laureate, Pauling proposed an incorrect triple-helix model of DNA. Although wrong, his work motivated other scientists to refine their understanding. The Double Helix 7. James Watson and Francis Crick (1953) ○ Using Franklin's X-ray data and Chargaff's rules, Watson and Crick proposed the double-helix model of DNA at Cambridge University. ○ Their model showed two strands running in opposite directions, held together by complementary base pairing (A-T and C-G). This structure explained how DNA could replicate and carry genetic information. In 1962, Watson, Crick, and Wilkins received the Nobel Prize in Physiology or Medicine for their discovery of DNA’s structure. Rosalind Franklin’s contributions were not fully acknowledged during her lifetime due to her early death in 1958 and gender biases of the era. She is now recognized as a key contributor. CHAPTER 2 DNA NUCLEOSOME Describe DNA location in Chromosomes Describe the Lowest Level of Chromosome Organization Differentiate Chromosomes and Chromatin Describe how different levels of chromatin organization allows chromosome to be fitted inside nucleus Differentiate heterochromatin (constitutive and facultative) and euchromatin Refresh on mosaicism DNA is organized into giant molecules called chromosomes Each chromosome is a single DNA molecule containing many genes Each gene gives the directions for making 1 protein In humans each chromosome has approximately 2000 genes (46,000 genes) Every gene has its place, or locus, on a chromosome How does a chromosome fit into a cell nucleus (10 µm in diameter) & perform its functions? Chromatin fibers and chromosome are two types of DNA present in different stages of the cell of the human body (interphase & complete mitosis) Chromosomes – is the highest condensed structure of DNA double helix present inside the nucleus. Chromatin fibers contains highly extended nucleoprotein fibers (DNA, histone proteins) Human DNA: average human cell has ~6.4 billion bp of DNA divided among 46 chromosomes (2 meters long) where each base pair is 0.34nm in length) Nucleosomes - the lowest level of chromosome organization DNA + associated proteins chromatin (nucleoprotein fibers) Histones are basic positively charged proteins that are associated with negatively charged DNA. Histones help package the DNA into structural units called nucleosome Nucleosome structure Roger Kornberg (1974, Harvard) – proposed that DNA & histones are organized into repeating subunits (nucleosomes) This assembly type increased the DNA packing ratio to 40 fold Ionic bonds (Non covalent bond) holds negatively charged phosphate backbones of DNA and positively charged histones Each nucleosome consists of a nucleosome core particle. Each particle consists of eight core histones (H2A, H2B, H3, H4) assembled into an octamer. The remaining histone (H1) is called the linker histone that binds to the linker DNA. Together the core interact with 148 bp DNA and the linker with additional 20 bp (Total 168 bp DNA) Chromosomes and Chromatin: Higher Levels of Chromatin Structure Lowest structural level of DNA is 10 nm nucleosome core In EM, see “dots” 30 nm in diameter (3X the nucleosome diameter; chromatin cut in cross-section) Linker histones & core histones implicated in higher-order chromatin structure (fiber-like structure) Next stage of packaging - 30 nm fiber is gathered into series of big, supercoiled loops Typically, the DNA loops begin & end with AT-rich sequences of variety of proteins Included among proteins is type II topoisomerase that regulates the degree of DNA supercoiling They untangle DNA of different loops when they intertwine Loops are usually not visible, only revealed during mitosis 1. DNA Double Helix (2 nm) ○ At the simplest level, DNA exists as a double-stranded helical molecule. 2. Nucleosome Formation (11 nm) ○ DNA wraps around histone proteins to form nucleosomes, which are the basic units of chromatin. ○ Each nucleosome consists of DNA wrapped 1.65 times around a core of eight histone proteins. 3. Chromatosome ○ A chromatosome is formed when an H1 histone binds to the nucleosome. 4. 30-nm Fiber ○ Nucleosomes are further folded and compacted into a 30-nm fiber, creating a more condensed structure. 5. 300-nm Loops ○ The 30-nm fiber forms loops averaging 300 nm in length, providing additional organization. 6. 250-nm-Wide Fiber ○ The 300-nm loops are further compressed and folded to produce a 250-nm-wide fiber. 7. Chromosome (700–1400 nm) ○ Finally, the 250-nm fibers are tightly coiled to produce the chromatid of a chromosome during cell division. Chromatin Two types of chromatin after mitosis in the diffuse interphase condition: euchromatin and heterochromatin. Euchromatin Heterochromatin Less condensed More condensed Gene expressing Silenced genes (methylated) Gene rich (higher GC content) Gene poor (higher AT content) Stains lighter Stains darker Euchromatin The euchromatin is less intense and is dispersed Euchromatin is a lightly packed form of chromatin (DNA, RNA and protein) rich in gene concentration Often under active transcription. Most active portion of the genome within the cell nucleus - 92% of the human genome is euchromatic, remainder is called heterochromatin Heterochromatin Heterochromatin (10%) remains condensed throughout interphase and stains intensely, indicating tighter packing Tightly packed form of DNA the part of a chromosome that is inactive in gene expression (no transcriptional activity) but may function in controlling metabolic activities and cell division. Two types ; constitutive heterochromatin and facultative heterochromatin Constitutive heterochromatin Exist in a compacted state most of the time and permanently silenced. Constitutive heterochromatin is usually repetitive (few genes) and forms structural functions such as centromeres or telomeres Position effect - If an active gene is accidentally moved adjacently to a constitutive heterochromatin; (as a result of transposition and translocation) they will become transcriptionally silenced Facultative heterochromatin only silenced during a certain phase of an organism’s life, it can be activated at certain stage. The case of the XX chromosome vs the XY chromosome (Females have more genes?) Only one X chromosome is transcriptionally active; the other one is a heterochromatic clump called the Barr body - X chromosome inactivation: X chromosome inactivation - Mary Lyon (British geneticist, 1961) studied inheritance of coat color in mice has made the following hypothesis: Heterochromatization of X chromosome in female mammals occurs during embryo gastrulation; therefore inactivates genes on X chromosome It is random in any given cell (could be paternally or maternally derived X chromosome) Inactive X chromosomes reactivate in germ cells before meiosis, so both X chromosomes become euchromatin & active during oogenesis. Therefore, all gametes get a euchromatic X chromosome. Mosaicism Paternal and maternal genes on X chromosomes are different Adult females are genetic mosaics (with different alleles functioning in different cells) Reflected in the patchwork coloration of the fur of some mammals (calico cats) Pigmentation genes in humans are not found on the X chromosome. Mosaicism in calico cats Calico Cats and X-Chromosome Inactivation ○ The coat color in calico cats is determined by genes located on the X chromosome, specifically the O (Orange) gene. ○ The O gene has two alleles: O (dominant): Produces orange fur. o (recessive): Produces black fur. ○ Female mammals (including cats) have two X chromosomes (XX), while males have one X and one Y chromosome (XY). ○ In calico cats, the genotype for coat color is heterozygous (Oo), meaning one X chromosome carries the O allele, and the other carries the o allele. In female mammals, to prevent overexpression of X-linked genes, one X chromosome in each cell is randomly inactivated during early embryonic development. This process is called X-chromosome inactivation or Lyonization. The inactivated X chromosome forms a condensed structure called a Barr body and its genes are silenced. ○ In calico cats: In some skin cells, the X chromosome with the O allele remains active, resulting in orange fur. In other skin cells, the X chromosome with the o allele remains active, resulting in black fur. This random inactivation creates a patchwork (mosaic) pattern of orange and black fur. The white fur in calico cats is caused by a separate gene, often related to the S (Spotting) gene. This gene controls the distribution of pigment-producing cells (melanocytes) during development: ○ In areas where melanocytes fail to populate, the fur remains white. The combination of black, orange, and white patches gives calico cats their distinctive appearance. Males typically have one X chromosome (XY) and thus can only express one coat color (orange or black, depending on the allele on their single X chromosome). Rare exceptions include: ○ XXY Males: Due to a genetic condition called Klinefelter Syndrome, male cats with two X chromosomes can display the calico pattern. However, these cats are typically sterile. Mosaicism in color blind women Mosaicism has been demonstrated in women who are carriers for red-green color blindness (patches of retinal cell with defective color vision among patches of normal vision) CHAPTER 3 Vectors and oligonucleotides Objectives: - Restriction Endonuclease: origin, nomenclature, types of cuts and RM system - Plasmid vectors – pBr 322 and pUC vectors - Ori site, MCS (directional cloning), Antibiotic selection, Blue-white selection, vector religation - Phage vectors - Cloning process - Cosmid vectors – Cloning vectors - Other types of vectors and capacity - M13, phagemids, YAC, BAC Restriction Enzymes are classified as endonucleases (enzymes that cuts within). Their biochemical activity is the hydrolysis ("digestion") of the phosphodiester backbone at specific sites in a DNA sequence. “Specific" means that an enzyme will only digest a DNA molecule after locating a particular sequence. RESTRICTION ENZYME DISCOVERY o In the 1950s, a group of scientists found out that bacteriophage λ cannot grow well in certain E.coli strains. o Three scientists below were awarded with Nobel prize for i. Restriction enzyme was first discovered in the late 1960s by Linn and Arber in E. coli. The mechanism is by cutting phosphate backbone ii. Discovery of HindIII iii. Restriction enzyme can be used for DNA mapping An Experiment Using Restriction Endonuclease: Boyer and Cohen - This early experiment used EcoRI to cut 2 plasmids. - Each plasmid had 1 EcoRI site - Cutting converted circular plasmids into linear DNA with the same sticky ends - The ends base pair - Some ends re-close while others joint together - DNA ligase joins 2 pieces with covalent bonds - This is an early experiment on recombinant molecule creation. - Restriction endonuclease EcoRI cleaves double-stranded DNA. - The recognition site for EcoRI is the hexameric sequence GAATTC: 5'..NNNNG|AATTCNNNN... 3' 3'..NNNNCTTAA|GNNNN... 5' - Cleavage occurs at the G residue on each strand so that the DNA is cut in a staggered fashion, leaving 5'-overhanging single-stranded ends (sticky ends) The Role of Restriction Endonucleases - Restriction endonuclease are discovered because it prevented invasion by foreign DNA (such as virus) from cutting E.coli DNA into pieces - These enzymes cut at sites within the foreign DNA instead of chewing from the ends (cutting within..right?) - By cutting DNA at specific sites they function as finely honed molecular knives. Naming Restriction Endonucleases Restriction endonucleases are named using the 1st three letters of their name from the Latin name of their source microorganism: (eg Hind III) First letter is from the genus H from Haemophilus Next two letters are the 1st two letters of the species name in from influenzae Sometimes the strain designation is included “d” from strain Rd If microorganism produces only 1 restriction enzyme, end the name with Roman numeral I; Hind I If more than one restriction enzyme is produced, the others are numbered sequentially II, III, IV, etc. Nomenclature of RE RE names are based on a species-of-origin. Taq polymerase (Thermophilus aquaticus, or Thermusaquaticus). ○ Eg. EcoR1 (from Escherichia coli) ○ BamHI (from Bacillus amyloliquifaciens) ○ Sma I (from Serratia marcescens) ○ Mlu I (from Micrococcus luteus) ○ Hpa I (from Haemophilus parainfluenzae) RE Recognition sequence and Type of ends in product ⚫ BamHI G^GATCC 5' overhang ⚫ SacI GAGCT^C 3' overhang ⚫ SmaI CCC^GGG blunt “Cohesive ends" or "sticky ends" - meaning that they could hydrogen bond to other compatible complementary strands Some RE leave a blunt end What do we call a DNA molecule that has ends that line up evenly with each other (i.e. neither end is overhanging)? These are "blunt" (meaning "not sharp") or "flush" (meaning "level or even") DNA ends. Blunt-end ligation o Blunt-end ligation using phage T4 DNA ligase, which catalyzes the ATP-dependent ligation of DNA molecules. o AMP and PPi are byproducts. Restriction Endonuclease Specificity Restriction endonucleases recognize a specific DNA sequence, cutting ONLY at that sequence ⚫ They recognize 4-bp, 6-bp, 8-bp palindromic sequences. ⚫ They cut DNA reproducibly in the same place. o Palindromic sequences are sequences that read the same in the forward and reverse direction. Restriction Endonuclease frequency ⚫ Four base cutter (44= 256 bp) are more frequently found than six base cutter (46= 4096 bp). Some 8 base cutter eg NotI (48= ≈ 65,000 bp) ⚫ The frequency of cuts lessens as the recognition sequence is longer Restriction-Modification System ⚫ What prevents these enzymes from cutting up the host DNA? ⚫ They are paired with methylases ⚫ Theses enzymes recognize, methylate the same site ⚫ Together they are called a restriction-modification system, R-M system ⚫ Methylation protects DNA ⚫ After replication the parental strand is already methylated Boyer & Cohen used EcoR1, to restrict DNA from an adult African clawed toad, Xenopus laevis. ⚫ The two ends of the pSC101 plasmid had joined to the two ends of the toad DNA fragment that contained the ribosomal RNA gene due to EcoR1 function, thus making it a functional plasmid Why do you use restriction enzymes? treat DNAs from 2 sources with the same restriction enzyme Restriction enzymes leave all cut fragments with staggered cuts (sticky ends); some make blunt cuts Sticky ends are single-stranded regions that pair with sticky ends on other DNAs cut by same enzyme —> restores double-stranded molecule. Blunt ends also ligated with ligase Plasmids & transformation Plasmids need to be introduced to cells via transformation Can be achieved ○ i) Chemically – Incubate cells in concentrated salt solution. Membranes will be leaky thus DNA uptake is possible ○ ii) electrically - High voltage to drive DNA into cells; a method called electroporation Requirements for a cloning vector: i. Should be small and easy to isolate ii. Should be capable of replicating in host cell iii. Should have an origin of replication iv. Should have convenient RE sites for inserting DNA of interest v. Should have a selectable marker (antibiotic resistance/blue white screening) to indicate which host cells received recombinant DNA molecule Vectors Vectors function as DNA carriers to allow replication of recombinant DNAs - Typical experiment uses 1 vector + foreign DNA. The inserted and foreign DNA depends on the vector for its replication as it does not have an origin of replication, the site where DNA replication begins. Foreign DNA cannot replicate on its own. - There are 2 major classes of vectors: Plasmids phages Plasmids Naturally occurring extrachromosomal DNA Plasmids are small circular dsDNA Plasmids contain several restriction sites can be cleaved by restriction enzymes, leaving sticky ends Artificial plasmids can be constructed by linking new DNA fragments to the sticky ends of plasmid Contain genes for antibiotic resistance, to allow selection by growth on media containing (lacking) antibiotics Can be replicated (amplified) in bacteria pBR 322 is a plasmid one of the first widely used E. coli cloning vectors. 4361 base pairs in length Developed by Boyer and Cohen Has ampicillin and tetracycline resistance gene Has an origin of replication Clones are plated on antibiotic medium. Clones that grow will definitely contain a cloning vector in them (that carries antibiotic resistance gene)and most probably have the gene of interest inserted in the cloning vector. Gene of interest spliced into the tetracycline gene cluster will lose their tetracycline resistance. Plasmids As Vectors pBR plasmids were developed early but are rarely used today pUC series is similar to pBR 40% of the DNA has been deleted Cloning sites are clustered together into one area called the multiple cloning site (MCS) MCS allows one to cut the vector and foreign gene with two different restriction enzymes and use a directional cloning technique to know the orientation of the insert ○ MCS- A site where multiple restriction enzyme sites (thus cloning can be carried out) are clustered together Directional cloning problem prevent inserts to be cloned in an opposite orientation used two different REs (located in the MCS) pUC vectors Allows blue-white screening pUC vectors and host cells can complement each other to produce β-galactosidase enzyme (expressed from lacZ gene) Plated on plates with indicator (X-gal), the β-galactosidase enzyme cleaved X-gal and produced blue coloured colonies Interrupting the pUC plasmid lacZ gene (inserting a gene into the MCS) will inactivate the gene. Complementation with the host cell The β-galactosidase gene is not possible so X-gal cannot be cleaved. Colonies produced will be white in color Other issues - Vector religation problem Solution -> Used alkaline phosphatase - removes 5’ phosphates (sticky) necessary for ligation in vectors. - Ligase joints 5’ phosphate group of DNA inserts to 3’ hydroxyl group of vector DNA λ Phage Vectors Lambda phage is a bacterial virus that infects E. coli Can either multiply within cells leading to cell lysis Viral DNA can integrate into the bacterial genome in a process called lysogeny. If lambda gene transcription is activated, then lytic infection occurs leading to phage release and infection of nearby E. coli cells. First phage vectors were constructed by Fred Blattner and colleagues (Charon phages) ○ Modifications included removal of the middle region and retention of the genes needed for phage replication ○ Foreign DNA will replace removed phage genes Advantage: Phage vectors can receive larger amounts of foreign DNA (up to 20kb of DNA) ○ Traditional plasmid vectors take much less Phage vectors require a minimum size of foreign DNA piece (12 kb) inserted to package into a phage particle (limitation is phage head) Bacteriophages are natural vectors that transduce bacterial DNA from one cell to another Phage vectors infect cells much more efficiently than plasmids transform cells Clones are not colonies of cells using phage vectors, but rather plaques, a clearing of the bacterial lawn due to phage killing the bacteria in that area Digestion with EcoR1 yields two arms (with cos sites) and two stuffer fragments. The arms are purified, stuffers discarded Ligate arms to insert and packaged with vector head and tail. Plate them on E.coli lawn. Plaques formed are positive clones. Arms cannot religate because two little DNA therefore cannot be packaged Cosmids Cosmids are designed for cloning large DNA fragments ○ Behave both as plasmid and phage and contain cos sites, cohesive ends of phage DNA that allow the DNA to be packaged into a λ phage head Plasmid origin of replication permitting replication as plasmid in bacteria ○ Nearly all λ genome removed so there is room for large inserts (40-50 kb) ○ Very little phage DNA cause them unable to replicate, but they are infectious and carry their recombinant DNA into bacterial cells Cosmids are hybrids of phages and plasmids that can carry DNA fragments up to 45 kb. They can replicate like plasmids but can be packaged like phage lambda. M13 Phage Vectors Long, thin, filamentous phage Contains: ○ Gene fragment with b-galactosidase ○ Multiple cloning site like the pUC family Advantage ○ This phage’s genome is single-stranded DNA ○ Fragments cloned into it will be recovered in single-stranded form ○ Useful in site directed mutagenesis study and for sequencing processes M13 Cloning to Recover Single-stranded DNA Product After infecting E. coli cells, single-stranded phage DNA is converted to double-stranded replicative form (RF) Use the replicative form for cloning foreign DNA into MCS Recombinant DNA infects host cells resulting in single-stranded recombinant DNA (+ve strand) Phage particles, containing single-stranded phage DNA is secreted from transformed cells and can be collected from media Phagemids - pBluescript Like cosmids have aspects of both phages and plasmids Has MCS inserted into lacZ’ gene to screen blue/ white colonies Has origin of replication of single-stranded phage f1 to permit recovery of single-stranded recombinant DNA MCS has 2 phage RNA polymerase promoters, 1 on each side of MCS Summary Two kinds of phage are popular cloning vectors ○ ‐ λ phage ‐ Has nonessential genes removed making room for inserts up to 20 kb - Cosmids can accept DNA up to 50 kb ○ - M13 phage - Has MCS - Produces single-stranded recombinant DNA Plasmids called phagemids also produce single-stranded DNA in presence of helper phage Engineered phage can accommodate inserts up to 20 kb, useful for building genomic libraries Eukaryotic Vectors and Very High Capacity Vectors There are vectors designed for cloning genes into eukaryotic cells Other vectors are based on the Ti plasmid to carry genes into plant cells Yeast artificial chromosomes (YAC) and bacterial artificial chromosomes (BAC) are used for cloning huge pieces of DNA There are vectors designed for cloning genes into eukaryotic cells Other vectors are based on the Ti plasmid to carry genes into plant cells Yeast artificial chromosomes (YAC) and bacterial artificial chromosomes (BAC) are used for cloning huge pieces of DNA BAC libraries are also used for cloning very large DNA fragments. BAC vectors can accommodate DNA inserts up to 300 kb, fulfill the needs of cloning large genes or map and sequence complex genomes. YACs can accommodate more (1-2 Mb) BACs are used more extensively than YACs. Most of the human genome has been sequenced using BAC rather than YAC clones. Cloning a Specific Gene Choosing a cloning vector: plasmids - usually < 10,000 bp (10 kb) inserts lambda phage - 10 - 20 kb inserts cosmids - combination of plasmids + phage; around 45 kb inserts BACs Bacterial Artificial Chromosomes - up to 300,000 bp (300 kb) YACs Yeast Artificial Chromosomes - up to 1,000,000 bp (1000 kb/1 Mb). HACs Human Artificial Chromosomes - up to 20,000,000 bp (so far!) (20,000 kb/20 Mb) Expression vectors - used for expressing proteins CHAPTER 4 TECHNIQUES IN MOLECULAR BIOLOGY a) AMPLIFICATIOn OF DNA and DNA analysis Describe the principles of PCR Explain the steps in PCR process Elaborate the applications of PCR Describe RT-PCR (reverse transcriptase vs Real time PCR) Polymerase Chain Reaction (PCR) used to amplify DNA and can be used to yield a DNA fragment for cloning The small sample of DNa serves as template for DNA polymerase Invented by Kary Mullis and colleagues in 1980s Special heat-stable polymerases are now used that are able to work after high temperatures Researchers no longer need to add fresh DNA polymerase after each round of replication as before Oligonucleotides complementary to a given DNA sequence prime the synthesis of only that sequence (primer) Use enzyme DNA polymerase to copy a selected region of DNA ◦ Employs a heat-stable DNA polymerase (Taqpolymerase), originally isolated from Thermus aquaticus, a bacterium that lives in hot springs at temperatures >90° (higher than normal) Heat-stable Taq DNA polymerase survives many cycles of heating. Theoretically, the amount of the specific primed sequence is doubled in each cycle. The steps involve: Mix DNA sample with 4 deoxyribonucleotides (dNTP - dATP, dTTP, dCTP, dGTP) Taq polymerase Also add a large excess of 2 short synthetic DNA fragments (oligonucleotides) complementary to DNA sequences at the 3' ends of the DNA region to be amplified These short oligonucleotides serve as primers to which nucleotides are added during the following replication steps Then heat the mixture to ~93°C, melting (denaturing) the DNA in mix & separating the strands – DENATURING STEP The mix is then cooled to ~60°C —> allows primers to bind 3' ends of both target DNA strands – ANNEALING STEP Raise the temperature to ~72°C —> allows the thermophilic polymerase to add complementary nucleotides to the 3' end of the primers – ELONGATION STEP As the polymerase extends the primers, it selectively copies the target DNA to form new complementary DNA strands Raised temperature causing newly formed & original strands to separate from each other The sample is then cooled to allow synthetic primers in mixture to bind once again to the target DNA, which is now present at twice the original amount Repeat cycle over & over again, doubling the amount of the specific DNA region flanked by the bound primers each cycle Generates large DNA amounts of this specific DNA region from minute amounts (billions of copies) in just a few hours Uses a thermal cycler that automatically changes the temperature of the reaction mixture, allowing each step in the cycle to take place PCR can generate large amounts of DNA from miniscule starting samples Troubleshooting If the bands obtained are numerous (unspecific annealing), raise the annealing temperature. If no bands are obtained (too stringent), lower the annealing temperature. A working annealing temperature should be around 2-6oC decrease from the lower Tm value of the primer pairs. ○ Tm is the temperature at which half of the oligonucleotide molecules are single-stranded and half are double-stranded where the primer is 50% annealed to its exact complement. Accurate prediction of Tm identifies duplexes formation at specific temperatures, allowing you to determine appropriate thermal cycling parameters. Both primers in PCR should be chosen to have a similar Tm. Therefore, selection of an annealing temperature should be 2–6°C below the lowest primer Tm. The application of PCR PCR has been used in criminal investigations to generate DNA quantities from a spot of dried blood left on crime suspect's clothing or from DNA present as part of single hair follicle left at crime scene For this purpose, one selects regions of genome for amplification that are highly polymorphic (i. e., vary at high frequency within the population) Same procedure can be used to study DNA fragments from well-preserved fossil remains that may be millions of years old Using Reverse Transcriptase PCR (RT-PCR) in cDNA Cloning Cloning a cDNA from just one mRNA whose sequence is known, is called reverse transcriptase PCR (RT-PCR) Difference between PCR and RT-PCR ◦ Starts with an mRNA, not dsDNA ◦ Begin by converting mRNA to DNA ◦ Use reverse primer to produce 1st strand DNA ◦ Use forward primers to convert ssDNA to dsDNA ◦ Continue with standard PCR ◦ Adding oligo dT adaptor/anchor Real-Time PCR (also known as Quantitative PCR/QPCR) Real-time PCR quantifies the amplification of the DNA as it occurs It monitors the amplification of a targeted DNA molecule during the PCR, i.e. in real-time, and not at its end, as in conventional PCR. As the DNA strands separate, they anneal to forward and reverse primers, PLUS a fluorescent-tagged oligonucleotide complementary to a part of one DNA strand that serves as a reporter probe A fluorescent-tagged oligonucleotide serves as a reporter probe ◦ Fluorescent tag at 5’-end ◦ Fluorescence quenching tag at 3’-end and the quencher tag will absorb the fluorescence as long as they are in close proximity As PCR progresses from the forward primer, the F tag is separated from the Q tag and allows the F tag to fluoresce Increased fluorescence can be detected and quantitated by fluorimeter Increase of fluorescence intensity is quantitated by the Real time PCR machine b) DNA Separation - Gel Electrophoresis & Pulse Field Gel Electrophoresis Explain the importance of gel electrophoresis Differentiate two types of gel electrophoresis Understand the principles of gel electrophoresis Gel Electrophoresis ❑ Gel electrophoresis provides a powerful method to separate, analyze and sometimes purify proteins and nucleic acids. ❑ Separations are based on sizes, charges and conformation. Two main types of gels are agarose and polyacrylamide ○ Polyacrylamide forms a tighter matrix and is typically used to separate proteins; smaller biomolecules such as short sequences of DNA and RNA. ○ Agarose forms a looser, more open matrix that enables separation of DNA molecules from around 200-50,000 bp Agarose gel electrophoresis Agarose gels are prepared in a box equipped with a removable comb. Once agarose has gelled, the comb will be removed to expose slots/wells for DNA to be placed or pipetted in together with dyes. Ladders are placed for size referencing. Agarose is placed inside the electrophoresis apparatus and filled with a running buffer (placed in horizontal position). Electricity moved the DNA (negatively charged) to the positive pole (anode). DNA were separated based on difference in sizes. The principle of movement is friction. Small DNA molecules encountered less frictional drag therefore moving faster. Larger DNA molecules had lower mobility therefore will be at a higher position. DNA is stained with a fluorescent dye (often EtBr) and visualized under UV illumination. Polyacrylamide Gel Electrophoresis (PAGE) A cross-linker polymer of acrylamide. SDS-PAGE is usually used to separate proteins in vertically positioned apparatus. SDS (Sodium dodecyl phosphate) will be used denature protein subunits so that they do not bind to one another ◦ Coat negative polypeptide so they move to anode ◦ Masks the natural charge of protein so they move according to mass The gel needs to be prepared between two glass plates. Oxygen will inhibit the polymerization process. A typical setup consists of a gel slab sandwiched between two glass plates, with the ends enclosed in upper and lower reservoirs of buffer Samples to be run are loaded in wells at the top of the gel, in conjunction with tracking dye. An electrical voltage is applied between the upper and lower reservoirs, causing the samples to migrate down through the gel. Stained with Coomassie Blue. (Pulse Field Gel Electrophoresis – PFGE) Pulsed Field - any electrophoresis process that uses more than one electric field alternatingly Pulsed Field Gel Electrophoresis (PFGE) is a technique used to separate especially long strands of DNA (up till Mb) by length in order to tell differences among samples. It operates by alternating electric fields to run DNA through a flat gel matrix of agarose. PFGE has traditionally been used for gene mapping and medical epidemiology. Voltage are periodically switched among three directions ○ i) Runs in the central axis ○ ii) Runs at the angle of 60 on the left side ○ iii) Runs at the angle of 60 on the right side Takes longer than normal electrophoresis Larger sizes DNA (30-50 kb) will run as a single, large diffuse band. Various length of DNA can be separated with alternating field direction eg yeast chromosomes with 0.2 till 2.2 Mb. c) DNA hybridisation & fingerprinting Describe DNA hybridisation Explain the process of DNA hybridisation Describe the types of DNA hybridisation – Southern, Northern, Western. Elaborate the applications of DNA hybridisation in DNA fingerprinting DNA hybridisation Helps in identifying specific DNA fragments Definition The ability of one stranded nucleic acid to bind to another single strand of complementary base sequence Southern Blotting A method developed by Edward Sothern Used restriction enzyme (preferably 6 base cutters eg EcoR1, HindIII) to cut genomic DNA. Electrophoresis of the fragments. Denature with an alkaline solution Transfer to a membrane (nitrocellulose/nylon). Hybridize to a specific probe. Probes are used to identify a desired gene segment from among the thousands of irrelevant ones Two types are widely used ◦Polynucleotides (also called oligonucleotides) ◦Antibodies Polynucleotide Probes Researchers used the cloned homologous gene from another organism ○ Consider there is enough sequence similarity to permit hybridization ○ Need to lower stringency of hybridization conditions to tolerate some mismatches ○ High temperature, high organic solvent concentration and low salt concentration are factors that caused the hybridization of a probe and a DNA with minimal mismatches Protein-based Polynucleotide Probes If homologous DNA from another organism is absent.. If amino acid sequence is known, deduce a set of nucleotide sequences to code for these amino acids Construct these nucleotide sequences chemically using the synthetic probes Can use several synthetic probes ◦ Genetic code is degenerate with most amino acids having more than 1 nucleic acid triplet ◦ Must construct several different nucleotide sequences for most amino acids Northern Blotting If you want to know how actively expressed a gene is in different tissues. Steps.. ✔ Collect RNAs from different tissue samples ✔ Electrophorese them and blot in a technique called Northern blotting ✔ Hybridise to a labelled cDNA probe ✔ Detection of labelled band through X-ray film Can detect in which tissue is the gene expressed Abundance of transcript is based on band intensity Immunoblots (Western blotting) Electrophoreses proteins. Used antibodies/antiserum as probes. Used a second antibody that is labelled for detection. DNA fingerprinting During the study of human blood protein, α-globin by Alec Jeffreys, he found fragments containing a sequence of bases repeated several times. This kind of repeated DNA is called a minisatellite. These highly variable DNA (known to not contribute to the functions of genes, are repeated within genes. Each individual has a unique pattern of minisatellites (the only exceptions being multiple individuals from a single zygote, such as identical twins). Minisatellites (Fragments that contain repeated sequences of bases (10-60 bases) that are repeated many times (5-50 times). All individuals differ in the basic patterns. Using Southern blotting, he detected the minisatellites using probes. Usage: DNA fingerprinting can be used to identify criminals. DNA obtained from a crime scene that matches with the detained suspect will nail the suspect (no two individuals have the same patterns..) DNA fingerprints have extreme sensitivity. DNA fingerprinting can also be used to establish parentage. Parents will have similar VNTR patterns with their offspring and so is between siblings. e.g. a Ghanaian boy with a British mother wants to find his mother (mitochondrial DNA is maternally inherited). d) DNA sequencing Know the types of DNA sequencing Understand the principles of DNA sequencing Explain the process of DNA sequencing Elaborate the applications of DNA sequencing Maxam-Gilbert base destruction method DNA sequencing is the determination of the precise sequence of nucleotides in a sample of DNA Bases of a DNA molecule are selectively destroyed Not used very much anymore because reagents are highly toxic and very dangerous Used purified DNA (need not to be cloned) Radioactive labelling at 5’ end (adding gamma-32P ATP. Chemical treatment (four different chemicals) generates breaks at a small proportion of one or two of the four nucleotide bases in each of four reactions (G, A+G, C, C+T). The most popular method is called the dideoxy method or Sanger method (named after its inventor, Frederick Sanger, who was awarded the 1980 Nobel prize in chemistry [his second] for this achievement) The Procedure The DNA to be sequenced is prepared as a single strand (needs denaturation by heat) This template DNA is supplied with a mixture of all four normal (deoxy) nucleotides ○ dATP ○ dGTP ○ dCTP ○ dTTP A short primer sequence complementary to the 3’ end. Different tubes of all four dideoxynucleotides, (modified nucleotides) each present in limiting quantities and each labeled with a "tag" that fluoresces a different color / radioactively labelled (OH group absent) ○ ddATP ○ ddGTP ○ ddCTP ○ ddTTP DNA polymerase I Because all 4 normal nucleotides are present,chain elongation proceeds normally until, by chance, DNA polymerase inserts a dideoxynucleotide (shown as colored letters) instead of the normal deoxynucleotide If the ratio of normal nucleotides to the dideoxy versions is high enough, some DNA strands will succeed in adding several hundred nucleotides before insertion of the dideoxy version halts the process. At the end of the incubation period, the fragments are separated by length from longest to shortest. The resolution is so good that a difference of one nucleotide is enough to separate that strand from the next shorter and next longer strand. Each of the four dideoxynucleotides fluoresces a different color when illuminated by a laser beam and an automatic scanner provides a printout of the sequence. Overview of Sanger dideoxy method The dideoxy chain termination procedure developed by Frederick Sanger begins with a primer of precisely defined length that has a radioactive 32P-phosphate label at its 5' end. The primer is designed to hybridize at the 3'-end of the template strand whose sequence is to be determined. Synthesis of a new strand of DNA is initiated from the primer, and a chain termination process (incorporation of a 2'-,3'-dideoxynucleotide, which leaves no 3'-OH group for further chain growth) is used to stop synthesis selectively at any one of the four DNA nucleotides. This generates a series of fragments whose relative lengths can then be used to read the nucleotide sequence. Gene to be sequenced is used as a template for the synthesis of new DNA strands, each randomly terminating due to the incorporation of a chain terminating dideoxynucleotide in 4 different reaction tubes. This produces a population of molecules, each terminating at a different site. Running the products in each tube on a gel allows the determination of where each chain terminating dideoxynucleotide was incorporated. CHAPTER 5 TRANSGENESIS ◼ Define transgenesis ◼ Describe gene transfer method ◼ Explain the transgenesis processes ◼ Describe characteristics in transgenic animals, plants and therapeutic proteins. Transgenesis is the process of introducing a foreign gene (transgene) into a living organism so that the organism will exhibit a new property and transmit that property to its offspring. Methods of Gene Transfer ◼ Gene transfer can be transient or stable ◼ In transient transfection, DNA is not integrated to the host chromosome (temporary but high expression of target gene) ◼ In stable transfection, the foreign DNA is integrated into the chromosomal DNA and the genetics of the recipient cell will be forever changed ◼ There are three types of genetic material, double stranded gene segments, oligonucleotides and cDNA sequences. ◼ Transfection is the method of introducing new DNA into a eukaryotic cell. Physical methods Chemical methods Biological methods Microinjection Lipids and liposome mediated gene transfer Conjugation Biolistic Non-liposomal Transformation Electroporation Transduction Agrobacterium mediated transfer (plant) Biological methods Conjugation Conjugation typically involves plasmids, which are small, circular DNA molecules separate from the bacterial chromosome. The F plasmid (fertility plasmid) is one of the most studied and allows a bacterium to act as a donor during conjugation. The donor cell contains the conjugative plasmid (e.g., F plasmid or R plasmid, where "R" denotes antibiotic resistance) while the recipient cell does not have the plasmid. The donor cell produces a sex pilus (or conjugative pilus), a thin protein structure that connects the donor to the recipient. Once contact is established, a single strand of the plasmid DNA is transferred from the donor to the recipient. The donor and recipient cells replicate the DNA strand to complete their plasmids, so both cells end up with a full plasmid. In some cases, the F plasmid integrates into the donor's chromosomal DNA, creating an Hfr cell. During conjugation, part of the donor's chromosomal DNA is transferred to the recipient, potentially incorporating new genetic traits into the recipient's genome. Steps in the Conjugation Process: 1. Contact Initiation: ○ The donor cell attaches to the recipient using the pilus. 2. Pilus Retraction: ○ The pilus pulls the two cells together, forming a conjugation bridge. 3. Plasmid Transfer: ○ A relaxase enzyme initiates the transfer by nicking the plasmid at the origin of transfer (oriT). ○ One strand of the plasmid DNA moves through the conjugation bridge into the recipient cell. 4. DNA Replication: ○ Both the donor and recipient replicate their plasmid DNA, completing the process. 5. Separation: ○ The two cells separate, each now potentially capable of acting as a donor in future conjugation events. Transformation The DNA involved in transformation is "naked," meaning it is free in the environment and not contained within a cell or virus. Bacteria must be in a physiological state called competence to undergo transformation. Competence can be natural (in species like Streptococcus pneumoniae and Bacillus subtilis) or induced artificially (e.g., using calcium chloride or electroporation in the lab). The acquired DNA may integrate into the recipient's chromosome through homologous recombination or exist as a plasmid. Process: DNA from lysed cells is released into the environment. This DNA may include chromosomal fragments or plasmids. Competent bacteria bind to the extracellular DNA using specific surface proteins. A single strand of DNA is transported into the bacterial cell, while the complementary strand is degraded. The internalized DNA may integrate into the bacterial genome via homologous recombination if it shares similarity with the recipient's DNA. Alternatively, plasmid DNA may remain as an independent replicating entity. If successfully integrated, the acquired genes can be expressed, leading to new traits in the recipient bacterium. Transduction: genetic material is transferred from one bacterium to another by a bacteriophage (a virus that infects bacteria) Bacteriophages act as carriers of DNA between bacterial cells. Unlike conjugation, transduction does not require direct physical contact between donor and recipient bacteria. The transferred DNA may integrate into the recipient bacterium's genome, leading to new genetic traits. 1. Generalized Transduction: Definition: Any random piece of the donor bacterium's DNA is packaged into the phage during the lytic cycle. Process: 1. A bacteriophage infects a donor bacterium and degrades its genome. 2. During assembly, the phage mistakenly packages fragments of bacterial DNA instead of viral DNA. 3. The phage carrying bacterial DNA infects a recipient bacterium. 4. The bacterial DNA may recombine with the recipient’s genome. Outcome: Transfer of random bacterial genes. 2. Specialized Transduction: Definition: Only specific genes near the phage integration site are transferred, occurring during the lysogenic cycle. Process: 1. A temperate phage integrates its genome into the host's chromosome at a specific site (prophage stage). 2. When the prophage excises to enter the lytic cycle, it may take adjacent bacterial genes with it. 3. The phage infects a new bacterium, transferring both viral and bacterial DNA. 4. The bacterial DNA integrates into the recipient’s genome. Outcome: Transfer of specific bacterial genes near the phage insertion site. - Agrobacterium mediated transfer Physical methods Microinjection ◼ Microinjection is the use of a glass micropipette to inject a liquid substance into a living cell but may also include intercellular space. ◼ Microinjection usually involved an inverted microscope with a magnification power of around 200x. ◼ Sometimes it is performed using a dissecting stereo microscope at 40x-50x or a traditional compound upright microscope. ◼ Inverted microscopy is a very popular technique for live cell imaging. ◼ Living cells are observed through the bottom of a cell culture vessel. ◼ To transfer genes into animals; ❑ Inject the rDNA with a fine glass needle into the pronuclei present in the embryo, 12 – 14 hours after fertilization ❑ A pronuclei is the nucleus of a sperm or an egg cell during the process of fertilization, after the sperm enters the ovum, but before they fuse. ❑ Sperm and egg cells are haploid, meaning they carry half the number of chromosomes. ◼ Transfer the embryo to a recipient mother where it can grow and develop into a normal animal ◼ The embryo will be implanted on a surrogate/foster mother to grow and develop. ◼ The F1 generation will be tested for transgene, and breeding continues to produce homozygosity (true breed). Biolistic ◼ Microparticle gun / A gene gun or a biolistic or bioballistics particle delivery system – particle bombardment where accelerated microprojectiles deliver DNA straight into cells ◼ DNA to be transformed are coated into microscopic beads coated with gold/tungsten ❑ The coated beads are attached to the end of the plastic bullet and loaded into the firing chamber of the gene gun. ❑ An explosive force fires the bullet with DNA coated beads towards the target cells that lie just beyond the end of the barrel. ❑ Some of the beads pass through the cell wall into the cytoplasm of the target cells Electroporation ◼ Electroporation – DNA can be delivered directly into cells using an electrical pulse. ◼ Electroporation caused an electrical field to be applied to cells in order to increase the permeability of the cell membrane (pore formation). ◼ This allowed chemicals, drugs, or DNA to be introduced into the cell. Chemical methods Lipids and liposome mediated gene transfer ❑ Liposomes are spheres of lipids that can transport molecules into cells ❑ Promote transport after fusing with cells. - involves the use of lipid-based vesicles to deliver genetic material into cells. Liposomes are spherical vesicles composed of lipid bilayers that can encapsulate DNA, RNA, or other biomolecules. - DNA or RNA is encapsulated within the liposome. - The liposome fuses with the cell membrane, delivering the genetic material into the cytoplasm. - In some cases, endocytosis facilitates uptake. Process: Lipids (often cationic) are dissolved and mixed in an aqueous solution to form liposomes. The genetic material (DNA or RNA) is added to the liposome preparation, allowing it to encapsulate or form complexes with the nucleic acids. Positively charged lipids interact electrostatically with negatively charged DNA or RNA to form stable liposome-DNA complexes (lipoplexes). The lipoplexes are added to the culture medium of target cells. The liposome binds to the cell membrane through electrostatic interactions. In some cases, ligands on the liposome surface may target specific receptors on the cell. The liposome fuses with the cell membrane or is taken up by the cell via endocytosis. Once inside the cell, the liposome breaks down, releasing the encapsulated genetic material. The genetic material escapes from the endosome into the cytoplasm. The genetic material reaches the nucleus (if necessary) and is transcribed and translated into proteins. Non liposomal ◼ Isolated DNA were mixed with a mixture of Calcium chloride and Potassium phosphate. ◼ The solution were incubated with the cells ◼ A fraction of the cells will take the calcium phosphate precipitate via endocytosis. - DNA is mixed with a calcium chloride solution. - The mixture is added to a phosphate buffer, forming a calcium phosphate-DNA precipitate. - The precipitate is added to cells, facilitating DNA uptake via endocytosis. Preparation of DNA Solution: A solution containing the plasmid DNA or other genetic material to be transferred is prepared in a buffer. DNA is mixed with the calcium chloride solution under gentle agitation to avoid clumping. The calcium-DNA mixture is added to a phosphate buffer The interaction between calcium ions and phosphate ions leads to the formation of a calcium phosphate-DNA precipitate. The calcium phosphate-DNA precipitate is immediately added to the cultured cells in the growth medium. The cells are incubated, allowing the precipitate to settle on the cell surface. Cells take up the DNA precipitate through endocytosis or phagocytosis. A brief shock may be applied to increase transfection efficiency by destabilizing the cell membrane temporarily. After a few hours (typically 4–24 hours), the medium containing the precipitate is removed and replaced with fresh growth medium to prevent cytotoxicity. The DNA enters the cell's nucleus, where it can be transcribed and translated to produce the desired protein or function. Biological method of gene transfer ◼ Widely used strategy to get genes into eukaryotic cells, leading to transcription & translation. ◼ Four methods i. Conjugation ii. Bacterial transformation Direct uptake of exogenous DNA through the cell membrane by a competent cell. iii. Retroviral transduction ◼ Transduction is the process by which DNA is transferred from one bacterium to another by a virus. ◼ It also refers to the process whereby foreign DNA is introduced into another cell via a viral vector. ◼ Transduction is the process by which foreign DNA is introduced into a cell by a virus or viral vector. ◼ Transduction is a common tool used by molecular biologists to stably introduce a foreign gene into a host cell's genome (both bacterial and mammalian cells). ◼ Transgene is inserted into lentiviral DNA (retrovirus). ◼ Virals infect vector. ◼ Viral particles will be injected into blastoderm embryo producing transgenic chicks iv. Agrobacterium mediated transfer ◼ Agrobacterium tumefaciens is a soil borne gram negative bacterium. ◼ It invades many dicot plants when they are injured at the soil level and causes crown gall disease. ◼ The ability to cause crown gall disease is associated with the presence of the Ti (tumour inducing) plasmid within the bacterial cell. ◼ Ti plasmid can be used to transport new genes into plant cells. ◼ Bacterium infects plant, transfers Ti plasmid to host cells ◼ T-DNA integrates into the plant DNA causing abnormal proliferation of plant cells ◼ T-DNA genes direct the synthesis of unusual organic acids, opines which can serve as an energy source to the infecting bacteria but are useless to the plant Transgenic Transgenic Animals ◼ Ralph Brinster & Richard Palmiter, 1981 - introduced gene for rat growth hormone (GH) into fertilized mouse eggs ◼ Injected DNA constructed to have rat GH gene coding region just downstream from mouse metallothionein gene promoter (a strong promoter) ◼ Treat mice with metals or glucocorticoids —> GH induced, mice got very big ◼ Transgenic animals useful for determining effects of overexpression of particular DNA sequence ◼ Transgenic animals also being developed as part of agricultural biotechnology ◼ Place foreign growth hormone genes into cow —> grow much leaner, milk production ◼ Excess growth hormone stimulates conversion of nutrients into protein rather than fat (leaner) Transgenic plants ◼ Introduce gene into cultured plant cells —> grow into mature plants with foreign gene ◼ During infection, Ti plasmid of bacterium enters plant cell & incorporates into plant chromosomes ◼ Induces cell to proliferate & provide nutrients for bacteria ◼ Ti plasmid can be isolated from bacteria & linked with foreign genes —> make recombinant plasmid The benefit ◼ Used to introduce bacterial genes for insect-killing toxins; protect plants from insect predators ◼ Significant increase in photosynthetic efficiency —> great increases in crop production ◼ Bacterial genes involved in nitrogen fixation activity & introduce them into chromosomes of leguminous plants that now depend on added fertilizer for reduced nitrogenous compounds ◼ Identify genes responsible for N2 fixation in Rhizobium —> deliver to plants —> less fertilizer Therapeutic Proteins/Antibodies ◼ Therapeutic proteins are proteins that are engineered in the laboratory for pharmaceutical use. ◼ Insulin was the first therapeutic protein to be introduced to treat diabetes in the 1920s. ◼ The fastest growing class of therapeutic proteins is antibodies. - Human insulin production. CHAPTER 6 RECOMBINANT/DNA REPAIR ✔ Describe the Holliday Model for Homologous Recombination ✔ DNA damage - Alkylation of bases - Ultraviolet damage - Gamma and X-rays ✔ DNA repair - Excision repair (Base and nucleotide) - Mismatch repair (Wrong base entered) Homologous Recombination Occurs between homologous chromosomes during meiosis The process scrambles the genes of maternal and paternal chromosomes resulting in nonparental combinations in the offspring Meiotic recombination forms physical links between homologous chromosomes that allow them to align properly during meiotic prophase so they separate properly during meiotic metaphase It also plays an important role in allowing cells to deal with DNA damage by recombination repair Holliday Model for recombination The mechanism of homologous recombination was mainly obtained from the study of E. coli. Although bacteria do not undergo meiosis, homologous recombination could occur during or immediately after DNA replication. When two homologous DNA molecules line up/two nonsister chromatids line up during meiosis. There are cuts in one strand of both DNAs. The cut strands cross and join homologous strands, forming the Holliday structure (or Holliday junction). Resulted in the formation of heteroduplex (A DNA molecule with two constitutive strands that derived from distinct sources) by branch migration. DNA strands may be cut along either the vertical line or horizontal line. The vertical cut will result in crossover between f-f' and F-F' regions. The heteroduplex region will eventually be corrected by mismatch repair. The horizontal cut does not lead to crossover after mismatch repair. However, it could cause gene conversion. DNA DAMAGE & REPAIR DNA can be damaged in many different ways, if left unrepaired this damage can lead to mutation, changes in the base sequence of DNA DNA damage is not the same as mutation though it can lead to mutation If a particular kind of DNA damage is likely to lead to a mutation, we call it genotoxic DNA damage is chemical alteration (from G-C to ethyl G-C) DNA mutation (From G-C to A-T) ⚪ DNA damage is a chemical alteration ⚪ Mutation is a change in a base pair Common examples of DNA damage Base modifications caused by alkylating agents Pyrimidine dimers caused by UV radiation Damage Caused by Alkylation of Bases ⚪ Alkylation is a process where electrophiles: ⚪ Encounter negative centers (in yellow) ⚪ Attack them ⚫ Add carbon-containing groups (alkyl groups) ⚫ Favourite sites of alkylation is N7 guanine and N3 adenine ⚫ N7 alkylation of guanine did not change the base-pairing properties N3 alkylation produced 3-methyladenine [3mA] that cannot base pair with any other bases ⚫ thus stalling DNA replication (non coding base) ⚫ Lead to mutation Cells that lose control over replication may lead to cancer ⚪ Alkylating agents like ethyl methane sulfonate (EMS) add alkyl groups to bases ⚪ Some alkylation don’t change base-pairing Others cause DNA replication to stall Cytotoxic ⚪ Lead to mutations if cell attempts to replicate without damage repair Third type change base-pairing properties of a base, so are mutagenic ⚫ Damage Caused by Radiation ⚪ Ultraviolet rays ⚪ Comparatively low energy Result in formation of pyrimidine dimers, also called cyclobutane pyrimidine dimers (CPDs) ⚫ UV radiation damage to DNA can be directly repaired by photoreactivation - a photolyase, ⚫ Uses energy from near-UV to blue light to break bonds holding 2 pyrimidines together which is actually two separate enzymes that catalyze repair of CPDs ⚫ Two enzymes responsible are CPD photolyase and (6-4) photolyase ⚫ CPD photolyase binds to the lesions, absorb light in the UV-A to blue region, breaking the bonds (not in placental mammals) ⚫ Gamma and x-rays ⚪ Much more energetic ⚪ Ionize molecules around the DNA ⚪ Form highly reactive free radicals that attack DNA Alter bases Break strands ❑ These lesions (dimers) block DNA replication (non-coding) ❑ What base to insert? ❑ Wrong bases inserted may lead to mutations. ⚫ Damage by Gamma and X-rays ⚫ Ionizing the molecules esp water ⚫ Form free radicals which are substance without paired electron ⚫ 8-oxoguanine will be read as thymine genotoxic mutation (altering base /breaking strands) Humans, another kind of damage by Gamma and X rays, O6-methyl guanine methyltransferase by accepting the methyl/ethyl group (suicide enzyme). DNA repair (Excision repair) Two basic ways to do it ❑ Directly underdoing it ❑ Remove damaged section and replace a new one ⚫ Percentage of DNA damage products that can be handled by direct reversal is small ⚫ Most damage involves neither pyrimidine dimers nor O6-alkylguanine ⚫ Another repair mechanism is required, excision repair is the process that removes most ⚪ Damaged DNA is removed damaged nucleotides ⚪ Replaced with fresh DNA ⚪ Base and nucleotide excision repair are both used, BER and NER, respectively ⚫ Base Excision Repair in E. coli DNA glycosylase removed base from the sugar – apurinic or apyrimidinic ⚫ AP endonuclease cuts or nicks DNA strand ⚫ Phosphodiesterase removes the AP sugar phosphate ⚫ DNA Polymerase I performs repair synthesis ⚫ DNA ligase seals the gap ⚪ Base excision repair (BER) acts on subtle base damage Begins with DNA glycosylase Extrudes a base in a damaged base pair Clips out the damaged base ⚪ Leaves an apurinic or apyrimidinic site that attracts DNA repair enzymes DNA repair enzymes Remove the remaining deoxyribose phosphate Replace it with a normal nucleotide ⚫ Nucleotide Excision Repair (NER) Nucleotide excision repair typically handles bulky damage that distorts DNA double helix ⚫ (pyrimidine dimers) NER in E. coli begins when damaged DNA is clipped by an endonuclease on either side of ⚫ the lesion, sites 12-13 nucleotide apart Allows damaged DNA to be removed as part of resulting 12-13-base oligonucleotide ⚫ In E. coli ⚫ NER system recognizes damaged nucleotide ⚫ uvrABC endonuclease cuts the bulky damaged sites DNA polymerase and ligase in action ⚫ Mismatch repair after replication in E.coli (wrong base entered) ⚫ Methylated adenines determines parental strands ⚫ mutH, L and S introduce nicks. ⚫ Exonuclease removed strand. ⚫ DNA pol and ligase. Methyl transferase adds the CH3 Coping with DNA Damage Without Repair ⚫ Direct reversal and excision repair are true repair processes ⚫ Eliminate defective DNA entirely ⚫ Cells can cope with damage by moving around it ⚪ Not true repair mechanism ⚪ Better described as damage bypass mechanism CHAPTER 7 Mobile genetic elements Define mobile genetic elements Describe the discovery of mobile genetic elements Explain the two mechanisms of transposition Differentiate autonomous and non autonomous transposons Explain how transposon shaped evolution – P elements in Drosophila What is transposon? Organism’s DNA do not remain unchanged. Can be damaged or mutated / undergo recombination. Transposon is a small piece of DNA that can insert itself into another place in the genome. Also known as the jumping genes. Barbara McClintock (1902-1992; Nobel - 1983) in 1940s planted self pollinating maize plants (use the pollen from the individual to pollinate the same plant’s flower). Observed different characteristics - leaf pattern, kernel color) Why do the genes get dispersed throughout the genome? Barbara McClintock initially ignored this phenomenon ❑ Found certain mutations in patterns & markings in leaf & kernel coloration of maize (corn) ❑ Some unstable, appearing & disappearing from one generation to next or even in same plant ❑ Concluded some genes had moved from 1 site to another in chromosome affecting gene expression ❑ Called this genetic rearrangement transposition & the moving genes transposable elements. McClintock worked with what is known as the Ac/Ds system in maize. Through these experiments, McClintock recognized that breakage occurred at specific sites on maize chromosomes. The first transposable element she discovered was a site of chromosome breakage, aptly named "dissociation" (Ds). Movements of Ds are regulated by an autonomous element called "activator" (Ac), which can also promote its own transposition. Bacterial Transposons A transposable element moves from one DNA address to another Originally discovered in maize, transposons have been found in all kinds of organisms ○ Bacteria ○ Plants ○ Humans Shapiro et al studied phage mutations in 1960s ○ - phage coat is made of protein and always has the same volume DNA is much denser than protein (More DNA in phage, denser phage) gal- phages are denser, suggesting addition of extra DNAs that inactivate the gal gene. These extra genes are called insertion sequences (ISs) Insertion sequence contained special sequences at the transposon’s both end Set of genes that catalyzes transposition called the transposase gene Cohen discovered that DNA sequences flanking the transposon were inverted. One strand can base-pair and produce a loop structure. Mechanisms of transposition The repeated sequences flanking a transposon did not exist before insertion. Transposase cuts the DNA in a staggered fashion Transposon insertion left gaps After insertion, gaps are filled. In late 1960s, transposons were found in bacteria ❑ They were found to encode a protein (transposase) that facilitates insertion of mobile element into the target DNA site; transposase catalyzes breakage & reunion of DNA required for insertion ❑ Transposase catalyzes transposon excision from donor DNA site & insertion at target DNA site Complex Transposons The term “selfish DNA” implies that insertion sequences and other transposons replicate at the expense of their hosts, providing no value in return Some transposons do carry genes that are valuable to their hosts, antibiotic resistance is among the most familiar Antibiotic Resistance and Transposons – an example Donor plasmid has Kanr, harboring transposon Tn3 with Ampr Target plasmid has Tetr After transposition, Tn3 has replicated and there is a copy in target plasmid Target plasmid now confers both Ampr, Tetr Transposition Mechanisms Transposons are sometimes called “jumping genes”, DNA doesn’t always leave one place for another When it does, nonreplicative transposition: (CLASS II TE) ○ “Cut and paste” ○ Both strands of original DNA move together from 1 ○ place to another without replicating Transposition frequently involves DNA replication (CLASS I TE) ○ 1 copy remains at original site ○ New copy inserts at the new site ○ Replicative transposition ○ “Copy and paste” Cut-and-paste (Class II TE) Studies on bacterial transposition indicate that this mechanism is mediated by 2 separate transposase subunits that bind to specific sequences at 2 ends of transposon The 2 subunits then come together to form an active dimer that catalyzes a series of reactions leading to transposon excision Transposase-transposon complex then binds to target DNA where transposase catalyzes reactions required to integrate transposon into its new residence Terminal repeats recognized by transposases & required for transposition into target DNA (found in both bacteria & eukaryotes) Eukaryotic genomes contain large numbers of transposable elements - ~40% of DNA in human cell nucleus is derived from transposable elements Vast majority of these elements cannot move from place to place; they have either been crippled by mutation or their movement is suppressed by the cell Retrotransposons Retrotransposons replicate through an RNA intermediate Retrotransposons resemble retroviruses ○ Retroviruses can cause tumors in vertebrates ○ Some retroviruses cause diseases such as AIDS Before studying retrotransposons, look at replication of the retroviruses Class of virus is named for its ability to make a DNA copy of its RNA genome This reaction is the reverse of the transcription reaction – reverse transcription Virus particles contain an enzyme that catalyzes reverse transcription reaction, called reverse transcriptase Viral genome is RNA, with long terminal repeats at each end Reverse transcriptase makes linear, ds-DNA copy of RNA ds-DNA copy integrates back into host DNA = provirus Host RNA polymerase II transcribes the provirus into genomic RNA Viral RNA packaged into a virus particle Transposons in human Thus, many transposons can insert themselves within center of protein- coding gene Humans - some hemophilias result from transposon jumping into center of key blood-clotting gene ○ ~1 in 500 human mutations is result of transposable element insertion Some are replicated & DNA copy is inserted into target site, leaving donor site unchanged (bacteria) Retrotransposons Several eukaryotic transposons transpose in a way similar to retroviruses ○ TY of yeast ○ copia of Drosophila Start with DNA in the host genome Make an RNA copy Reverse transcribe it within a virus-like particle into DNA that can insert into new location HERVs (human endogenous retroviruses) likely transposed in the same way until the ability to transpose was lost TY of yeast Example of a eukaryotic transposable element is the TY element of yeast. This element resembles a primitive retrovirus. A retrovirus is a RNA virus where after being uncoated in the host cell, converts its RNA to a DNA copy by the enzyme reverse transcriptase. The DNA copy of the retrovirus is inserted into the eukaryotic genome, and it remains there as a provirus until it is excised and undergoes transcription to produce new viral particles. Transposition involves an RNA intermediate that is generated by transcription of the TY element A reverse transcriptase (encoded by the TyB gene of the element) makes a DNA copy of the element which is then inserted into a new site in the yeast genome Autonomous transposon Variegation in the color of maize kernels is caused by multiple reversions of an unstable mutation in the C locus, responsible for kernel color Mutation and its reversion result from Ds (dissociation) element ○ Transposes into the C gene ○ Mutates it ○ Transposes out again, revert to wild type Ds and Ac were transposable elements Ds transposes to C and mutate it, causing the gene to be mutated Ac was an autonomous transposon, can induce Ds out of gene C → causing reversion Ds no not have the transposase gene, so needed Ac transposase to help it transpose Role of mobile genetic elements in evolution 1. No function - a genetic parasite; invades & spreads through organism & offspring, if no serious adverse effects on ability of host to survive & reproduce 2. Regardless of origin, once DNA is present in genome it has potential to be used in evolution so some think that transposons are a key mechanism in creating genomic changes that fuel evolution Transposable elements can carry adjacent parts of host genome with them as they move from one site to another, so 2 unlinked segments of host genome can be joined to form new, composite segment May be primary mechanism in evolution of proteins that are composed of domains derived from different ancestral genes P Elements in D. melanogaster Transposable element of Drosophila melanogaster (P element) is example of evolution shaped by transposons Examination of lab fruit flies and the natural populations of flies at the start of the 1900s are devoid of the P element In contrast, every member of the species caught in wild today has multiple copies of P element Thought to have been introduced into single D. melanogaster within past 80 years, probably by transmission from individual of another Drosophila species Then it spread rapidly through entire species population Transmission of genetic material from one species to another, whether between different fruit flies or different types of vertebrates is likely mediated by parasites (virus) They pick up DNA fragments from one host & transfer it to subsequent host The P-M system of hybrid dysgenesis in Drosophila is caused by conjunction of 2 factors: ○ Transposable element (P) contributed by the male ○ M cytoplasm contributed by the female allows transposition of the P element Hybrid offspring of P males and M females suffer multiple transpositions of P element causing them to be sterile P females contained a suppressor of the P element. Offspring of either P males or M males with P females are fertile. Nowadays P elements are used as mutagenic elements for transpotional studies. CHAPTER 8 Regulations of gene activity Describe the mechanism of transcription in prokaryotes Describe control of transcription ▪ The lac operon ▪ The trp operon ▪ The ara operon Prokaryotic transcription In E.coli, the RNA polymerase consisted of the core enzyme and a σ- factor. The core polymerase enzyme consisted of the β, β’, α2 and ω (basic transcription machinery). The holoenzyme included the σ- factor. This causes the ability to transcribe specific genes. RNA polymerase subunit The σ-factor allows initiation of the transcription process by binding to specific promoters. Therefore, the σ-factor can select the genes to be transcribed. Without the σ-factor , the core enzyme will lack specificity. The core enzyme binds loosely to a closed promoter complex (promoter searching) The σ-factor will allow the holoenzyme to bind tightly to the promoter complex (10-17 bp), causing it to be opened from a closed double stranded form. The opening process involved the melting of a short region of the DNA and followed by the tight binding of the open promoter complex. There are two core promoter regions, -35 and -10, a common sequence as TTGACA and TATAAT. Some very strong promoters had an extra sequence further upstream called the UP element (enhancing RNA polymerase binding). The core subunit β lies near the active site of the RNA polymerase where phosphodiester bonds are formed. The α subunit had independently folded N- and C-terminal domains. The C-terminal domains can recognized and bind to the promoter’s UP elements. This caused tight binding of the promoter-polymerase complex. DNA is bound to the σ-subunit Transcription initiation Binding ○ The binding of polymerase core enzymes to the σ-factor will cause the complete holoenzyme to open the DNA at the specific initiation site. Initiation ○ The binding will cause the double helix DNA to separate and initiate transcription at the proper start site. Elongation ○ As the RNA chain is elongated, the σ-factor will be released. The σ-factor only initiates the transcription process, but not the elongation. It is released from the open promoter complex randomly (stochastic model) leaving the core enzyme to carry the transcription process. The σ-factor can be used by a different core enzyme. Transcription elongation The RNA polymerase causes the melting of a 10-17 bp of the transcription start site. This creates a transcription bubble that will move during transcription, each time exposing the template strand to be transcribed. Topoisomerases relax the transcribing DNA strand. As it moves, the polymerase maintains a short melted region approximately 11-16 bases long and contains a DNA-RNA hybrid about 9 bp long The movement allows the DNA to unwind ahead of the advancing polymerase. The DNA closes up again behind it. Binding happens one at a time Transcription termination Transcription termination in prokaryotes occurs through two primary mechanisms: rho-dependent termination and rho-independent termination. Rho-Dependent Termination (needs rho to for the transcription to stop) Rho Protein Role: Rho is a hexameric helicase protein that binds to the RNA molecule at a specific sequence called the rut site (Rho utilization site), which is rich in cytosines and lacks secondary structure. Process: 1. The Rho protein binds to the rut site on the newly synthesized RNA strand. 2. Using energy from ATP hydrolysis, Rho moves along the RNA towards the RNA polymerase. 3. Downstream of the rut site, RNA polymerase encounters a termination signal (a GC-rich region or other pause-inducing sequence) and pauses. 4. Rho catches up to the paused RNA polymerase and uses its helicase activity to unwind the RNA-DNA hybrid within the transcription bubble. 5. This unwinding disrupts the transcription complex, causing RNA polymerase, the RNA transcript, and the DNA template to dissociate, terminating transcription. Whenever the transcript has grown long enough, the Rho protein binds at the upstream rut site. The Rho protein translocates down the mRNA Interaction with the RNA polymerase complex will stimulate release of the transcript. Rho-Independent Termination This mechanism relies on specific sequences in the RNA transcript that form a secondary structure and destabilize the transcription complex. Process: 1. A palindromic GC-rich region in the RNA transcript folds back on itself, forming a stable hairpin structure. 2. Following the hairpin, the RNA transcript typically contains a series of uracil residues (poly-U tail). The RNA-DNA hybrid in this region is weaker due to the A-U base pairing (only 2 hydrogen bonds compared to 3 in G-C pairs). 3. The combination of the hairpin structure pulling on the RNA polymerase and the weak A-U base pairs causes the RNA-DNA hybrid to destabilize. 4. RNA polymerase releases the RNA transcript and detaches from the DNA template, terminating transcription. ρ-independent terminators consist of inverted repeats that cause a hairpin to form. This destabilizes the RNA-DNA complex A string of TTTTTs in the non template DNA strand results in a weak rU-dA base pair Feature Rho dependent Rho independent Dependence on Rho Requires rho protein No requirement for rho protein Sequence Rut site on RNA GC-rich hairpin and poly-U Requirement tail Energy Requirement Requires ATP for Rho activity No external energy required Mechanism of Helicase unwinds RNA-DNA Hairpin loop destabilizes Termination hybrid transcription complex Operons Lac operon The lac operon (lactose operon) is an operon required for the transport and metabolism of lactose in Escherichia coli and some other enteric bacteria. It has three adjacent structural genes, lacZ, lacY, and lacA. The genes encode β-galactosidase, lactose permease, and galactoside acetyltransferase, respectively. The lacI gene encodes for the repressor protein. Further downstream there are the promoter region and operator region. In its natural environment, the lac operon allows for the effective digestion of lactose. Lactose permease, which is embedded in the cytoplasmic membrane, transports lactose into the cell. β-galactosidase, a cytoplasmic enzyme, subsequently cleaves lactose into glucose and galactose. Together with galactoside transacetylase, their transcription can produce a mRNA called polycistronic message. Negative control In a cell, it would be wasteful to produce the enzymes when there is no lactose available or if there is a more preferable energy source available, such as glucose. Without lactose in the cell, the repressor protein binds to the operator and prevents the read through of RNA polymerase into the three structural genes (lac Z, lacY and lacA). With lactose in the cell, lactose in a form of allolactose binds to the repressor. This causes a structural change in the repressor and it loses its affinity for the operator. RNA polymerase can then bind to the promoter and transcribe the structural genes. In this system lactose acts as an effector molecule. Lactose is not the preferred carbohydrate source for E. coli. If lactose and glucose are present, the cell will use all of the glucose before the lac operon is turned on. This type of control is termed catabolite repression. To prevent lactose metabolism, a second level of control of gene expression exists. The promoter of the lac operon has two binding sites. One site is the location where RNA polymerase binds. The second location is the binding site for a complex between the catabolite activator protein (CAP) and cyclic AMP (cAMP). Negative control The binding of the CAP-cAMP complex to the promoter site is required for transcription of the lac operon. The presence of this complex is closely associated with the presence of glucose in the cell. As the concentration of glucose increases the amount of cAMP decreases. As the cAMP decreases, the amount of complexity decreases. Positive control This decrease in the complex inactivates the promoter, and the lac operon is turned off. Because the CAP-cAMP complex is needed for transcription, the complex exerts a positive control over the expression of the lac operon. Due to usage, concentration of glucose decreases causing the amount of cAMP to start to increase. As the cAMP increases, the amount of complex increases and the lac operon turns on. trp operon The trp operon produces products that are used to manufacture an amino acid, tryptophan. The pathway controlled by the trp operon is an example of an anabolic pathway. The lac operon controls a catabolic pathway, because it breaks down complex molecules to release energy for biological work. The lac operon is controlled both by the abundance of its substrate, lactose, and by the abundance of glucose, an alternative to glucose. The trp operon is controlled by the amino acid itself This operon contains five structural genes: trp E, trp D, trp C, trp B, and trp A, which encode tryptophan synthetase. It also contains a promoter where RNA polymerase binds to a repressor gene (trp R) which synthesizes a specific protein. The protein that is synthesized by trp R then binds to the operator which then causes the transcription to be blocked. In the lac operon, allolactose binds to the repressor protein, allowing gene transcription, while in the trp operon, tryptophan binds to the repressor protein effectively blocking gene transcription. In both situations, repression is that of RNA polymerase transcribing the genes in the operon. Also unlike the lac operon, the trp operon contains a leader peptide and an attenuator sequence which allows for graded regulation. Within the operon's regulatory sequence, the operator is blocked by the repressor protein in the presence of tryptophan (thereby preventing transcription) and is released in tryptophan's absence (thereby allowing transcription). It is an example of repressible negative regulation of gene expression. Attenuation is a second mechanism of negative feedback in the trp operon. The repression system targets the intracellular trp concentration whereas the attenuation responds to the concentration of charged tRNAtrp. The trpR repressor decreases gene expression by altering the initiation of transcription, while attenuation does so by altering the process of transcription that's already in progress. At the beginning of the transcribed genes of the trp operon is a sequence of at least 130 nucleotides termed the leader transcript (trpL). The attenuation efficiency is correlated with the stability of a secondary structure embedded in trpL, this transcript includes four short sequences designated 1-4, each of which is partially complementary to the next one. Thus, three distinct secondary structures (hairpins) can form: 1-2, 2-3 or 3-4. The hybridization of sequences 1 and 2 to form the 1-2 structure is rare because the RNA Polymerase waits for a ribosome to attach before continuing transcription past sequence 1. The formation of a hairpin loop between sequences 2-3 prevents the formation of hairpin loops between both 1-2 and 3-4. The 3-4 structure is a transcription termination sequence (abundant in G/C and immediately followed by several uracil residues), once it forms RNA polymerase will disassociate from the DNA and transcription of the structural genes of the operon can not occur Attenuation is made possible by the fact that in prokaryotes (which have no nucleus), the ribosomes begin translating the mRNA while RNA polymerase is still transcribing the DNA sequence. This allows the process of translation to affect transcription of the operon directly. Ara operon The ara operon codes for three enzymes that are required to catalyze the metabolism of arabinose. The three structural genes are arranged in an operon that is regulated by the araC gene product. The operators are araO1 and araO2 The operators lie between the araC and inducer site. araI lies between the structural genes and the operator. The araI1 and araI2 are DNA-binding sites that, when occupied by AraC, induce expression. Regulation of the arabinose operon is much more complex than the lactose operon. It is an operon that encodes enzymes needed for the catabolism of arabinose in Escherichia coli. When arabinose is absent, there is no need to express the structural genes. AraC does this by having its repressor protein binding simultaneously to araI and araO2 As a result the intervening DNA is looped. These two events block access to the PBAD promoter which is a very weak promoter. A comparison between two possible DNA conformations in response to specific binding proteins or transcriptional regulators. Panel (a): Looping Out In this scenario, a DNA loop forms, facilitated by proteins (represented as yellow and blue ovals) binding to specific sequences (red regions) on the DNA. The looping mechanism occurs when two distant sites on the DNA come into proximity, often mediated by protein-protein interactions or DNA bending proteins. Purpose: This looping out can enable interactions between regulatory sequences (e.g., enhancers or silencers) and the transcription machinery at the promoter, influencing gene expression positively (activation) or negatively (repression). Panel (b): No Looping Out In this scenario, DNA looping is prevented. The diagram suggests that some structural or mechanistic constraint (indicated by the label "this twist cannot occur") inhibits the DNA from forming a loop. Reason: This could occur due to: ○ The positioning of binding proteins or sequences that make the required twist physically impossible. ○ Incompatibility of DNA bending due to protein obstruction or lack of flexibility in the DNA sequence. ○ Changes in the topology of the DNA (e.g., supercoiling) that prevent loop formation. Effect: Without looping, regulatory regions may not interact with the