Cell and Molecular Biology Chapter 10 PDF
Document Details
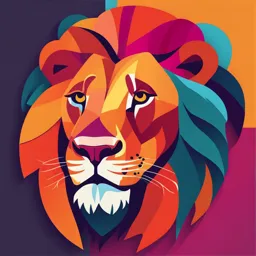
Uploaded by WellManneredBildungsroman
2020
Gerald Karp, Janet Iwasa, Wallace Marshall
Tags
Related
- Chapter 6 How Cells Read the Genome: Molecular Biology of The Cell PDF
- Molecular Biology of The Cell: Chapter 5 - DNA Replication, Repair, and Recombination PDF
- Molecular Biology Lecture 1 PDF
- Molecular Cell Biology and Genetic Module (CBG 1103) PDF
- 2023 Molecular Biology and Diagnostics Main Module PDF
- IB Biology SL Study Guide PDF
Summary
This document is a chapter from the textbook "Cell and Molecular Biology". It covers the concept of a gene as a unit of inheritance, including Mendel's laws and the discovery of chromosomes. The chapter also discusses DNA, its structure, and various related topics.
Full Transcript
Cell and Molecular Biology Ninth Edition Gerald Karp, Janet Iwasa, Wallace Marshall Chapter 10 The Nature of the Gene and the Genome 10.1 | The Concept of a Gene as a Unit of Inheritance (1 of 3) ▪ Genome – the collective body of genetic information that i...
Cell and Molecular Biology Ninth Edition Gerald Karp, Janet Iwasa, Wallace Marshall Chapter 10 The Nature of the Gene and the Genome 10.1 | The Concept of a Gene as a Unit of Inheritance (1 of 3) ▪ Genome – the collective body of genetic information that is present in a species. ▪ 1865 – Mendel - the foundation for the science of genetics. ▪ Mendel crossbred plants through several generations and counted the number of Fig. 10.1 Overview depicting important early individuals having various discoveries on the nature of the gene. characteristics. ▪ He established the laws of inheritance based on his studies of pea plants. Copyright ©2020 John Wiley & Sons, Inc. 10.1 | The Concept of a Gene as a Unit of Inheritance (2 of 3) Trait Dominant allele Recessive allele Height Tall Dwarf Seed color Yellow Green Seed shape Round Angular (wrinkled) Flower color Purple White Flower position Along stem At stem tips Pod color Green Yellow Pod shape Inflated Constricted (See www.mendelweb.org for a discussion of Mendel’s work.) TABLE 10.1 Seven Traits of Mendel’s Pea Plants Copyright ©2020 John Wiley & Sons, Inc. 10.1 | The Concept of a Gene as a Unit of Inheritance (3 of 3) Mendel’s Conclusions: 1. Characteristics of organisms are governed by units of inheritance called genes. o Each trait is controlled by two forms of a gene called alleles. o Alleles could be identical or nonidentical. o When alleles are nonidentical, the dominant allele masks the recessive allele. 2. A reproductive cell (gamete) contains one allele for each trait. o Somatic cells arise by the union of male and female gametes. o Two alleles controlling each trait are inherited; one from each parent. 3. The pairs of alleles are separated (segregated) during gamete formation. 4. Alleles controlling different traits segregate independently of each (independent assortment). Copyright ©2020 John Wiley & Sons, Inc. 10.2 | The Discovery of Chromosomes ▪ Following Mendel’s work a number of biologists were concerned with the other aspect of heredity – its physical basis within the cell. ▪ Cytoplasm split randomly during cell division ▪ Nuclear contents precisely split ▪ During cell division, the material of the nucleus became organized into visible threads which were named chromosomes, meaning Fig. 10.2 Events observed (1888) in the “colored bodies” roundworm Ascaris following fertilization Copyright ©2020 John Wiley & Sons, Inc. 10.3 | Chromosomes as the Carriers of Genetic Information (1 of 5) ▪ Chromosomes are present as pairs of homologous chromosomes. ▪ During meiosis, homologous chromosome pairs form a bivalent; then segregate into different cells. ▪ Genes on the same chromosome do not assort independently and are part of the same linkage group. ▪ Chromosomal behavior correlated with Mendel’s laws of inheritance. Fig. 10.3 Homologous chromosomes Copyright ©2020 John Wiley & Sons, Inc. 10.3 | Chromosomes as the Carriers of Genetic Information (2 of 5) Genetic Analysis in Drosophila ▪ Thomas Hunt Morgan was the first to use Drosophila (fruit flies) in genetic research. ▪ Morgan only had available wild type flies but one he developed his first mutant, it became a primary tool for genetic research. ▪ Mutation was recognized as a mechanism for variation in populations. Fig. 10.4 The fruit fly Drosophila melanogaster, allelic variants Copyright ©2020 John Wiley & Sons, Inc. 10.3 | Chromosomes as the Carriers of Genetic Information (3 of 5) Crossing Over and Recombination ▪ Alleles of two different genes originally on a given chromosome did not always remain together during meiosis ▪ F. A. Janssens: Homologous chromosomes of bivalents wrapped around each in meiosis Proposed this interaction resulted in the breakage and exchange of maternal and paternal chromosomal fragments ▪ Termed cross-over or genetic recombination Fig. 10.6 Visualizing sites of crossing over Copyright ©2020 John Wiley & Sons, Inc. 10.3 | Chromosomes as the Carriers of Genetic Information (4 of 5) Crossing Over and Recombination ▪ Frequency of recombination indicates distance, and increases as distance increases. ▪ The positions of genes along the chromosome (loci) can be mapped from recombination frequencies. Fig. 10.7 Crossing over provides the mechanism for reshuffling between maternal and paternal chromosomes Copyright ©2020 John Wiley & Sons, Inc. 10.3 | Chromosomes as the Carriers of Genetic Information (5 of 5) Mutagenesis and Giant Chromosomes ▪ Exposure to a sub-lethal dose of X-rays increased the rate of spontaneous mutations → inducing mutations became a quicker method to study genes. ▪ Polytene chromosomes of Drosophila provided visual banding patterns correlated to gene Fig. 10.8 Giant polytene chromosomes of larval positions insects Copyright ©2020 John Wiley & Sons, Inc. 10.4 | The Chemical Nature of the Gene (1 of 9) The Structure of DNA ▪ The mystery of DNA structure was investigated by a number of laboratories in both the United States and England in the early 1950s and was solved by James Watson and Francis Crick at Cambridge University in 1953. Fig. 10.9 Model of DNA built by James Watson and Francis Crick at Cambridge University, 1953. Copyright ©2020 John Wiley & Sons, Inc. 10.4 | The Chemical Nature of the Gene (2 of 9) The Structure of DNA ▪ DNA is the genetic material in all organisms. ▪ The nucleotide is the building block of DNA. ▪ It consists of a phosphate, a sugar, and either a pyrimidine or purine nitrogenous base. ▪ Two different pyrimidines: thymine (T) and cytosine (C). ▪ Two different purines: adenine (A) and guanine (G). Fig. 10.10a,b The chemical structure of DNA Copyright ©2020 John Wiley & Sons, Inc. 10.4 | The Chemical Nature of the Gene (3 of 9) The Structure of DNA ▪ Nucleotide features: Directional structure where the ends are called 5' and 3' Linkage into nucleic acid polymers where sugars and phosphates form phosphodiester bonds between a 5' phosphate and 3' sugar hydroxyl group ▪ Chargaff rules of DNA base composition: [A] = [T] [G] = [C] [A] + [T] ≠ [G] + [C] Fig. 10.10c The chemical structure of DNA Copyright ©2020 John Wiley & Sons, Inc. 10.4 | The Chemical Nature of the Gene (4 of 9) The Watson-Crick Proposal 1. DNA is composed of two chains of nucleotides 2. These two chains form a spiral pair of right-hand helices. 3. The two chains are antiparallel, they run in opposite directions. 4. The sugar-phosphate backbone is the exterior of the molecule, and the bases are interior 5. Bases are perpendicular to sugar- phosphate backbone 6. DNA chains are held together by hydrogen bonds between bases Fig. 10.11a The double helix Copyright ©2020 John Wiley & Sons, Inc. 10.4 | The Chemical Nature of the Gene (5 of 9) The Watson-Crick Proposal 7. Double helix width 2nm 8. Pyrimidines are always paired with purines 9. Only A-T and C-G pairs fit within double helix. 10. Molecule has a major groove and a minor groove. 11. Complete turn is 10 base pairs 12. Complementary base sequences on each of the 2 strands Fig. 10.11b–d The double helix Copyright ©2020 John Wiley & Sons, Inc. 10.4 | The Chemical Nature of the Gene (6 of 9) The Importance of the Watson-Crick Proposal DNA was expected to fulfill three primary functions: 1. Storage of genetic information. A DNA segment would correspond to a gene, and the sequence would dictate the sequence of amino acids in a polypeptide. 2. Replication and inheritance capability. During replication, hydrogen bonds of the DNA helix were broken, causing separation of the strands → templates for assembly of a complementary strand. Fig. 10.12 Three functions required of 3. Expression of the genetic message. the genetic material Copyright ©2020 John Wiley & Sons, Inc. 10.4 | The Chemical Nature of the Gene (7 of 9) DNA Supercoiling ▪ DNA that is more compact than its relaxed counterpart is called supercoiled. ▪ Underwound DNA is negatively supercoiled, and overwound DNA is positively supercoiled. ▪ Negative supercoiling plays a role Fig. 10.13 Supercoiled DNA in allowing chromosomes to fit within the cell nucleus. Fig. 10.14 Underwound DNA Copyright ©2020 John Wiley & Sons, Inc. 10.4 | The Chemical Nature of the Gene (8 of 9) DNA Supercoiling ▪ Enzymes called topoisomerases change the level of DNA supercoiling. ▪ Type I – change the supercoiled state by creating a transient break in one strand of the duplex. ▪ Type II – make a transient break in both strands of the DNA duplex. Fig. 10.15a,b DNA topoisomerases Copyright ©2020 John Wiley & Sons, Inc. 10.4 | The Chemical Nature of the Gene (9 of 9) DNA Supercoiling ▪ In addition supercoiling and relaxing DNA, type II topoisomerases can tie a DNA molecule into knots or untie a DNA knot. ▪ They can interlink (catenate) independent DNA circles, or separate interlinked circles into individual components. Fig. 10.15c,d DNA topoisomerases Copyright ©2020 John Wiley & Sons, Inc. 10.5 | The Complexity of the Genome (1 of 8) DNA Denaturation ▪ Denaturation – the ability to separate into its separate components Thermal denaturation (or DNA melting) can be monitored by following the increase in absorbance of UV light by the dissolved DNA. Temperature where the shift in absorbance is half completed is the melting temperature (Tm). The higher the GC content (%G + %C) of the DNA, the higher the Tm. Fig. 10.16 Thermal denaturation of DNA Copyright ©2020 John Wiley & Sons, Inc. 10.5 | The Complexity of the Genome (2 of 8) DNA Renaturation ▪ Renaturation or reannealing is when single-stranded DNA molecules are capable of reassociating. ▪ In nucleic acid hybridization, complementary strands of nucleic acids from different sources can form hybrid molecules; this is important in DNA sequencing, cloning, and amplification. Fig. 10.17 The kinetics of renaturation of viral and bacterial DNAs. Copyright ©2020 John Wiley & Sons, Inc. 10.5 | The Complexity of the Genome (3 of 8) DNA Renaturation ▪ Studying reannealing rates of different genomes has provided insight into various types of sequences. ▪ Three broad classes of DNA sequences (differing in number of times their nucleotide sequence is repeated highly repeated fraction moderately repeated fraction nonrepeated fraction Fig. 10.18 Plot of renaturation kinetics for eukaryotic DNA. Copyright ©2020 John Wiley & Sons, Inc. 10.5 | The Complexity of the Genome (4 of 8) DNA Renaturation: Highly Repeated DNA Sequences ▪ Highly Repeated DNA Sequences – represent about 1–10% of total DNA. ▪ Satellite DNAs – short sequences that tend to evolve very rapidly. ▪ Minisatellite DNAs – unstable and tend to be variable in the population; form the basis of DNA fingerprinting. ▪ Microsatellite DNAs (STRs) – shortest sequences and typically found in small clusters; implicated in genetic disorders. Fig. 10.19 DNA fingerprinting Copyright ©2020 John Wiley & Sons, Inc. 10.5 | The Complexity of the Genome (5 of 8) DNA Renaturation: Highly Repeated DNA Sequences ▪ Fluorescence in situ hybridization (FISH) ▪ Fluorescent probes are generated towards a specific DNA sequence ▪ Allows determination of its location(s) within the genome of an organism. Fig. 10.20 Fluorescence in situ hybridization and the localization of satellite DNA Copyright ©2020 John Wiley & Sons, Inc. 10.5 | The Complexity of the Genome (6 of 8) DNA Renaturation: Highly Repeated DNA Sequences ▪ FISH can be used to visualize repetitive sequences like that found in satellite DNA localized in the centromeric regions of the chromosome, or for determining the position of single copy genes. Fig. 10.21 Chromosomal localization of a nonrepeated DNA sequence Copyright ©2020 John Wiley & Sons, Inc. 10.5 | The Complexity of the Genome (7 of 8) DNA Renaturation: Moderately Repeated DNA Sequences ▪ The moderately repeated fraction of the genomes of plants and animals can vary from 20% to more than 80% of total DNA. ▪ This fraction includes sequences that are repeated within the genome anywhere from a few times to tens of thousands of times. ▪ Some sequences code for abundant gene products, (e.g. rRNAs or histones), but most sequences lack a coding function. ▪ These noncoding elements are scattered (i.e., interspersed) throughout the genome and can be grouped into SINEs (short interspersed elements) or LINEs (long interspersed elements). Copyright ©2020 John Wiley & Sons, Inc. 10.5 | The Complexity of the Genome (8 of 8) DNA Renaturation: Nonrepeated DNA Sequences ▪ The nonrepeated (or single-copy) DNA sequences include genes that exhibit Mendelian patterns of inheritance and localize to a particular site on a particular chromosome. ▪ Included within the nonrepeated fraction are the DNA sequences that code for virtually all proteins other than histones, which comprise less than 1.5% of the human genome. ▪ Even though these sequences are not present in multiple copies, genes that code for polypeptides are usually members of a family of related genes, like the globins, actins, myosins, collagens, tubulins, integrins, and most other proteins in a eukaryotic cell. Copyright ©2020 John Wiley & Sons, Inc. 10.6 | The Stability of the Genome (1 of 7) Whole-Genome Duplication (Polyploidization) ▪ Offspring have four chromosome homologues rather than two. ▪ Common in higher plants ▪ Mechanisms: Two related species can mate to form a hybrid organism with the combined chromosomes from both parents (plants) 1-cell embryo can undergo chromosome duplication and retain the DNA. (animals) Fig. 10.22 A sample of agricultural crops that are polyploid Copyright ©2020 John Wiley & Sons, Inc. 10.6 | The Stability of the Genome (2 of 7) Duplication and Modification of DNA Sequences ▪ Gene duplication occurs within a portion of a single chromosome. ▪ Duplication may occur by unequal crossing over between misaligned homologous chromosomes. ▪ Duplication has played a major role in the evolution of multigene families. Fig. 10.23 Unequal crossing over between duplicated genes provides a mechanism for generating changes in gene number Copyright ©2020 John Wiley & Sons, Inc. 10.6 | The Stability of the Genome (3 of 7) Evolution of Globin Genes ▪ The globin gene family includes hemoglobin, myoglobin, and plant leghemoglobin. ▪ Ancestral forms have given rise to recent forms by duplication, gene fusion, and divergence. ▪ Some sequences, called pseudogenes, resemble globin genes but are nonfunctional. Fig. 10.24 A pathway for the evolution of globin genes Copyright ©2020 John Wiley & Sons, Inc. 10.6 | The Stability of the Genome (4 of 7) The Dynamic Nature of the Genome: “Jumping Genes” ▪ Barbara McClintock suggested that genetic elements were capable of moving around the genome. ▪ She called this genetic rearrangement transposition, and the mobile genetic elements transposable elements. Fig. 10.25 Visible manifestations of transposition in maize. Copyright ©2020 John Wiley & Sons, Inc. 10.6 | The Stability of the Genome (5 of 7) The Dynamic Nature of the Genome: “Jumping Genes” – Transposons ▪ Only certain sequences can act as transposons, but these insert into target sites randomly. ▪ It requires the enzyme transposase to facilitate insertion of transposons into target site. Fig. 10.26 Transposition of a bacterial transposon by a “cut-and paste” mechanism Copyright ©2020 John Wiley & Sons, Inc. 10.6 | The Stability of the Genome (6 of 7) The Dynamic Nature of the Genome: “Jumping Genes” – Transposons ▪ Integration of the element creates a small duplication in target DNA, which serves as a “footprint” to identify sites occupied by transposable elements. ▪ Retrotransposons use an RNA intermediate which produces a complementary DNA via reverse transcriptase. Fig. 10.27 Schematic pathways in the movement of transposable elements Copyright ©2020 John Wiley & Sons, Inc. 10.6 | The Stability of the Genome (7 of 7) The Dynamic Nature of the Genome: “Jumping Genes” Roles of Mobile Genetic Elements in Adaptive Genome Evolution ▪ Transposable elements can carry adjacent parts of the host genome with them as they move from one site to another. ▪ DNA sequences originally derived from transposable elements are found as parts of eukaryotic genes and DNA segments that regulate gene expression. ▪ Transposable elements themselves appear to have given rise to genes. ▪ A number of recent studies have found evidence that mammalian brain cells have a greatly elevated level of L1 retrotransposition compared to that of other tissues. Copyright ©2020 John Wiley & Sons, Inc. 10.7 | Sequencing Genomes: The Footprints of Biological Evolution (1 of 3) ▪ The genomes of hundreds of organisms have been sequenced. ▪ In 2004 the “finished” version of the human genome was reported, revealing that it contains about 20,000 genes. Fig. 10.28 Genome comparisons Copyright ©2020 John Wiley & Sons, Inc. 10.7 | Sequencing Genomes: The Footprints of Biological Evolution (2 of 3) Number of Protein-Coding Genes in the Human Genome ▪ Alternative splicing is when a single gene can encode a number of related proteins ▪ MicroRNAs are noncoding RNAs that can have gene regulatory functions. ▪ Proteins work together as complex networks rather than individual actors. Modest increase in protein numbers can generate significant network complexities. Copyright ©2020 John Wiley & Sons, Inc. 10.7 | Sequencing Genomes: The Footprints of Biological Evolution (3 of 3) Comparative Genomics: “If It’s Conserved, It Must Be Important” ▪ Genome regions that encode protein sequences or contain regulatory sequences that control gene expression are subject to natural selection, which tends to eliminate individuals whose genome contains mutations. ▪ If these sequences tend to be conserved, the best way to identify functional sequences is to compare the genomes of different types of organisms. ▪ Recent studies have shown a significant proportion of functional DNA sequences are constantly evolving and are not highly conserved. Copyright ©2020 John Wiley & Sons, Inc. 10.8 | Engineering Linkage: Engineering Genomes ▪ CRISPR-Cas9 and other DNA editing technologies have now made it possible to introduce specific changes in the genomes of diverse organisms. ▪ These edits tend to by small and targeted – the addition or deletion of a short sequence at a specific site in the genome. ▪ There have been a few projects on a much larger scale led by Craig Venter: Synthesis of the first synthetic bacterial cell capable of self-replication. Creation of a minimal bacterial genome that would support life (half the size of the original genome. Copyright ©2020 John Wiley & Sons, Inc. 10.9 | The Genetic Basis of “Being Human” (1 of 5) What Genes Are Unique to the Human Lineage? ▪ FOXP2 in human differs very little from that in chimps, and is called the “speech gene”. ▪ HAR1, which also differs little between humans and chimps and its function is unknown. ▪ AMY1 encodes the enzyme amylase and its frequency is remarkably different between humans and chimps. Fig. 10.29 Duplication of the amylase gene during human evolution Copyright ©2020 John Wiley & Sons, Inc. 10.9 | The Genetic Basis of “Being Human” (2 of 5) Genetic Variation within the Human Species Population ▪ Homo sapiens vs. Neanderthal and Denisovan ▪ Since the completion of sequencing from the Human Genome Project sequence, a great deal of attention has been focused on how DNA sequence varies within the human population. ▪ Genetic polymorphisms are sites in the genome that vary among different individuals, and usually refers to a genetic variant that occurs in at least 1 percent of a species population. Copyright ©2020 John Wiley & Sons, Inc. 10.9 | The Genetic Basis of “Being Human” (3 of 5) Genetic Variation within the Human Species Population DNA: Sequence Variation ▪ The most common type of genetic variability in humans occurs at sites where single nucleotide differences are found in a population. ▪ When present in at least 1 percent of the population, these sites are called single nucleotide polymorphisms (SNPs) and occur as two alternate alleles, such as A or G. ▪ On average, two randomly selected human genomes have about 3 million single nucleotide differences between them, or one every thousand base pairs. ▪ Current estimates suggest that each person harbors over 100 rare single nucleotide variants in his or her exome (the portion of the genome that codes for proteins). Copyright ©2020 John Wiley & Sons, Inc. 10.9 | The Genetic Basis of “Being Human” (4 of 5) Genetic Variation within the Human Species Population: Structural Variation ▪ Segments of the genome can change as the result of duplications, deletions, insertions, and inversions. ▪ Large changes range from hundreds to millions of base pairs in length, and are called structural variants. ▪ A typical human genome carries approximately 1000 structural variants, ranging in length from about 500 bases to 1.3 million bases (Mb). Fig. 10.30 Structural variants Copyright ©2020 John Wiley & Sons, Inc. 10.9 | The Genetic Basis of “Being Human” (5 of 5) Genetic Variation within the Human Species Population: Copy Number Variation ▪ The lengths of minisatellite sequences depend on the number of copies of the sequence that are present at particular sites in the chromosomes, an example of a copy number variation (or CNV). ▪ Larger-sized CNVs (