Neuronal Signaling and Nervous System Structure (BIO 215 Spring 2025)
Document Details
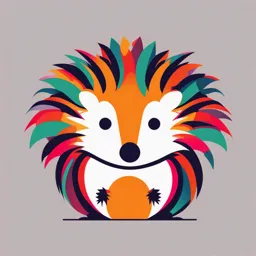
Uploaded by LikableHedgehog
2025
Dr. Colette Kabrita-Bou Serhal, PhD
Tags
Related
Summary
These lecture notes for BIO 215 cover the structure and function of the nervous system, including neuronal signaling. They detail the components of the central and peripheral nervous systems, the roles of neurons and glial cells, and the mechanisms of action potentials.
Full Transcript
Neuronal Signaling and Structure of the Nervous System Chapter 6 BIO 215 – spring 2025 Dr. Colette Kabrita-Bou Serhal, PhD SECTION A: NEURAL TISSUE I. The central and peripheral nervous systems II. Anatomy of a ne...
Neuronal Signaling and Structure of the Nervous System Chapter 6 BIO 215 – spring 2025 Dr. Colette Kabrita-Bou Serhal, PhD SECTION A: NEURAL TISSUE I. The central and peripheral nervous systems II. Anatomy of a neuron 1. Information flow in neurons 2. Myelin 3. Axon transport III. Functional classes of neurons IV. Roles of glial cells V. Neural growth and regeneration Components of the NS 1. Central nervous system (CNS): Brain + Spinal cord terminologies: tracts / pathways / commissures nuclei 2. Peripheral nervous system (PNS): Nerves and nerve fibers (afferent & efferent) terminologies: nerves ganglia The Central & Peripheral Nervous System Divisions of PNS 1. SNS: controls skeletal muscle 2. ANS: controls visceral organs a. sympathetic: (emergency) b. parasympathetic: (rest & digest) Cells of the CNS 1. Neurons: - Basic units - count 10% of the total # of cells; 50% of the volume of the CNS - generate electrical signals (basis of intercellular communication) - integrators (i.e. output reflects balance of several inputs) 2. Glia (neuroglia) - account for 90% of the number of CNS cells - physically & metabolically support neurons: Ä form myelin Ä regulate ECF composition in CNS Ä + formation of tight junctions of capillary wall (BBB) Ä provide fuel (glucose) to neurons and remove metabolic wastes (ammonia) Ä potential role in info signaling Ä immune defense in CNS Ä regulate the production & flow of CSF Ä support embryonic cells: guidance cues, source of growth factors Types of Glia CNS: – Oligodendrocytes (myelin-forming cells) – Astroglia (support neurons metabolically) – Microglia (serve immune function) PNS: Schwann cells (myelin-forming) Silverthorn: Human Physiology- An Integrated Approach Dendrites: receive information, typically neurotransmitters, then undergo graded potentials. Figure 6-1 Axons: undergo action potentials to deliver information, typically neurotransmitters, from the axon terminals. Figure 6-2 Among all types of neurons, myelinated neurons conduct action potentials most rapidly. The “myelin sheath” is actually a collection of cells called Schwann cells and oligodendrocytes that are closely associated with the neuron. Axonal Transport Important for the maintenance of axonal structure & function; a way by which some harmful agents & pathogens invade the CNS Involves transport of material between soma and axon terminal Carried out by microtubules and motor proteins (kinesins & dyneins) Two types: 1. Anterograde (mainly powered by kinesin) 2. Retrograde (mainly powered by dynein) Functional Classes of Neurons 1. Afferent neurons: info from tissues/organs ® CNS 2. Efferent neurons: info from CNS ® effector cells 3. Interneurons: majority of neuronal types info within CNS For each AN, there are 10 EN and 200,000 IN CNS PNS Figure 6-3 PNS = afferent neurons (their activity CNS = brain “affects” what will happen + next) into the CNS spinal cord; + all parts of efferent neurons (“effecting” change: interneurons movement, secretion, etc.) are in the CNS. projecting out of the CNS. Sensory receptors at ends of AN: a specialized portion of PM or specialized cell types associated with the neuron. Three Functional Types of Neurons Ref.: Human Physiology: An Integrated Approach 1998. Silverthorn. Prentice Hall. NEURAL COMMUNICATION Presynaptic membranes “deliver information” in the form of neurotransmitters. Postsynaptic membranes “receive information” because they have receptors for neurotransmitters. Figure 6-4 Transmission of Information in NS Neural pathways: - long axons between brain and SC / or different brain regions - v. few synapses ® little info processing Multineural (multisynaptic) pathways: Many synapses ®significant alteration in transmitted info (bec. of multiple integration sites). Neuronal Growth and Regeneration Neural development: 1. Generation: from neural tube (for CNS): stem cells ® postmitotic neuroblasts ® migration to target tissue guided by growth cone. 2. Axonal guidance: glial guidance (role of CAMs. NTs). 3. Synapse formation: synapse activity and competition for growth (neurotrophic) factors). 4. Fine-tuning of synapses: loss /degeneration of many synapsis through apoptosis. Division of neuron precursor stem cells is largely complete before birth. Generation of new neurons & modification of synaptic contacts can occur, though at a slow pace, after birth and throughout life as part of normal growth. Axonal Regeneration: Can occur if axonal damage is outside the CNS and does not affect the neuron’s body. Restoration of neuronal function is possible (with a delay though). B. Membrane Potentials ØBasic principles of electricity ØResting membrane potential ØMembrane potentials in excitable cells Measurement of the plasma membrane potential difference (or potential) Charges that separate across the membrane are only an extremely small fraction of the total number of ions inside & outside the cell The resting membrane potential exists because of tiny excess of –ve ions inside the cell and an excess +ve ions outside. Factors that Determine the Resting Membrane Potential 1. Passive forces (electrochemical gradient): Differences in specific ion concentrations (mainly Na+ & K+) in the intra- & extracellular fluids. Differences in membrane permeabilities to different ions (the membrane is much more permeable to K+ than to Na+ at rest) ® greater contribution of K+ to the transmembrane potential. 2. Active forces (membrane pumps): The electrogenic activity of the Na+/K+-pump. Forces acting on K+ when the Forces acting on Na+ when the membrane of a neuron is at (a) the membrane of a neuron is at (a) the resting potential (-70mV), & (b) the resting potential (-70mV), & (b) the K+ equilibrium potential (-90mV) Na+ equilibrium potential (+60mV) Leak channels Movements of Na+ & K+ across the plasma membrane of a resting neuron in the steady state. The passive movements (black arrows) are exactly balanced by the active transport (red arrows) of the ions in the opposite direction. Distribution of Gated Channels Along a Neuron Transmission of Information in the NS Generation of electrical signals (graded / action potentials) Graded potentials: signaling over short distances Action potentials: signaling over long distances Involve transient changes in the transmembrane potential from its resting value. Figure 6-13 Overshoot refers to the development of a charge reversal. A cell is “polarized” Repolarization is movement back because its interior toward the resting potential. is more negative than its exterior. Depolarization occurs Hyperpolarization is when ion the development of movement even more negative reduces the charge inside the cell. charge imbalance. (a) Membrane potential is closer to the resting potential with increasing distance from the depolarization site. (b) Local current surrounding the depolarized region produces depolarization of adjacent regions. Graded potentials & generation of local currents Figure 6-14 Graded potentials decay over distance. The size of a graded potential (here, graded depolarizations) is proportionate to the intensity of the stimulus. Graded potentials (a) Can be depolarizing or hyperpolarizing (b) Can vary in size (c) Are conducted decrementally (d) Can be summed Action Potentials Large, very rapid alterations in membrane potential Property of excitable membranes (e.g. nerve & muscle cells; some endocrine, immune & reproductive cells) Important for long-distance communication Mediated by voltage-gated channels (mainly Na+ & K+) An action potential is an “all-or-none” sequence of changes in membrane potential. The rapid opening of voltage-gated Na+ channels allows rapid entry of Na+, Action potentials result moving membrane potential from an all-or-none closer to the sodium sequence of changes equilibrium potential (+60 mv) in ion permeability due to the operation The slower opening of of voltage-gated voltage-gated K+ channels Na+ and K + channels. allows K+ exit, moving membrane potential closer to the potassium Figure 6-17 equilibrium potential (-90 mv) Positive- feedback relation between membrane depolarization & increased Na+ permeability, which leads to the rapid depolarizing phase of the action potential. Three subthreshold graded depolarizations, each proportional to the stimuli, are shown here. Four action potentials, each the result of a stimulus strong enough to cause a supra-threshold graded deloplarization, are shown in the right half of the figure. Figure 6-19 Threshold & the All-or-None Response of Action Potentials The threshold of most excitable membranes is about 15mV less negative than the resting membrane potential. Action potentials either occur maximally or do not occur at all. Information about the strength of the stimulus depends upon the frequency of action potentials transmitted per unit of time. Factors that Prevent the Generation of APs Work by blocking the voltage-gated Na channels ® no peripherally-generated signals can reach the brain. Include: - Local anesthetics: e.g. procaine (Novocaine) & lidocaine (Xylocaine) - Some toxins: e.g. tetrodotoxin Refractory Periods of Action Potential Absolute refractory period: – lasts for the duration of an AP. – No 2nd AP can be generated no matter how strong the stimulus is. Relative refractory period: – lasts 10-15 ms in neurons & coincides roughly with the period of afterhyperpolarization. – A 2nd AP can be generated if the stimulus is stronger that usual. Refractory Periods of Action Potential Refractory periods limit the # of APs that can be generated by an excitable membrane in a given period of time. Most neurons respond at frequencies of up to 100 AP/ sec They determine the direction of AP propagation. The only direction of AP propagation is away from a region of membrane that has recently been active. Propagation of an Action Potential Along a Plasma Membrane Factors that Determine the Velocity of Action Potential Propagation Fiber diameter the larger the diameter, the faster a.p. propagation. Whether or not the fiber is myelinated Propagation in myelinated fibers (via saltatory conduction) is faster than propagation in nonmyelinated fibers of the same diameter. Conduction velocities range from 0.5 m/s for small- diameter, unmyelinated fibers to about 100 m/s for large- diameter, myelinated fibers. Current during an action potential in (a) myelinated & (b) an unmyelinated axon. Action Potentials Resulting from Pacemaker Potentials Spontaneously generated APs. C. Synapses Synapses Anatomically specialized junctions (1014 in CNS) Can or ¯ the likelihood that the postsynaptic neuron will fire action potentials by producing a brief, graded potential. Excitatory synapse The membrane potential of a postsynaptic neuron is brought closer to threshold. Inhibitory synapse The membrane potential of a postsynaptic neuron is driven farther from threshold or stabilized at its present level. Four primary neurons One primary neuron communicate to one communicates to four secondary neuron. secondary neurons. Figure 6-22 Factors that Determine the Level of Postsynaptic Cell Excitability 1. The number of active synapses 2. The type of active synapses (i.e. number of excitatory or inhibitory synapses). Types of Synapses Electrical synapse – Signals are transmitted from presynaptic to postsynaptic neuron via gap junctions. – Flow of local currents in either direction between adjacent neurons. – Rapid mode of communication. – Rare in the mammalian nervous system. Chemical synapse Signals are transmitted from presynaptic to postsynaptic neuron by means of chemical messengers (Neurotransmitters). Diagram of a Synapse -Synaptic cleft: 10-20 nm -More than 1 NT could be released simultaneously from the axon terminal (co- transmitter). -Synaptic delay of 0.2 sec bet. the arrival of an a.p. at a presynaptic terminal & the membrane-potential changes in the postsynaptic cell. 1. Action potential, moving along axon’s voltage-gated Na+ AXON 1 and K + channels, arrives at terminal. Ca++ 2 2. Action potential opens voltage-gated 3 Ca++ channels. 3. Ca++ influx triggers 4 neurotransmitter release into the DENDRITE synaptic cleft. 4. The binding of neurotransmitters to receptor proteins in the postsynaptic membrane is linked to an alteration in its ion permeability. Figure 6-25 Diversity in synaptic form allows the nervous system to achieve diversity and flexibility in meeting its needs for communication and coordination of reflexes. Figure 6-24 Removal of the NT from the Synaptic Cleft Terminates the Response of the Postsynaptic Cell. HOW can it be removed? - NT can be actively transported back into the axon terminal, or into nearby glial cells. - NT can diffuse away from the synaptic cleft. - NT can be transformed by enzymes into ineffective substances, some of which can be transported back into the axon terminal for reuse. An excitatory postsynaptic potential (EPSP) is a graded depolarization that moves the membrane potential closer to the threshold for firing an action potential (excitement). Figure 6-26 An inhibitory postsynaptic potential (IPSP) is a graded hyperpolarization that moves the membrane potential further from the threshold for firing an action potential (inhibition). Figure 6-27 Threshold refers to the minimum graded depolarization that initiates the cyclic activity of the voltage-gated Na+ and K+ channels, resulting in the initiation of an action potential. The membrane potential of a real neuron typically undergoes many EPSPs (A) and IPSPs (B), since it constantly receives excitatory and inhibitory input from the axons terminals that reach it. Figure 6-28 Interaction of EPSPs & IPSPs at the Postsynaptic Neuron Real neurons receive as many as 200,000 terminals. A single EPSP is not enough to depolarize to threshold in the postsynaptic neuron (0.5 mV). Changes of about 15 mV are needed. Comparison of Excitatory & Inhibitory Synapses, Showing Effect on the Initial Segment Graded depolarizations are typically the result of the inward movement of Na+ (but can result from Ca++ influx and/or decreased K+ efflux). Graded hyperpolarizations are typically the result of the outward movement of K+ (but can result from decreased Na+ influx). Figure 6-30 Axo-axonal communication (here, between A & B) can modify classical synaptic communication (here, between B & C); this can result in presynaptic inhibition or presynaptic facilitation. Note: the Terminal B must have receptors Figure 6-31 for the signal released from A. Possible drug effects on synaptic effectiveness: A. release and degradation of the neurotransmitter inside the axon terminal. B. increased neurotransmitter release into the synapse. C. prevention of neurotransmitter release into the synapse. D. inhibition of synthesis of the neurotransmitter. E. reduced reuptake of the neurotransmitter from the synapse. F. reduced degradation of the neurotransmitter in the synapse. G. agonists (evoke same response as neurotransmitter) or antagonists (block response to neurotransmitter) can occupy the receptors. H. reduced biochemical response inside the dendrite. Figure 6-32 Acetylcholine (ACh) Major NT in the PNS & some areas in the brain. Synthesized from Choline + Acetyl-CoA, in the cytoplasm of presynaptic terminals. [ACh] at the synapse is reduced by: – The action of Acetylcholinesterase, located on both pre- & postsynaptic membranes, which breaks down ACh. Choline is reused by the presynaptic cell in the synthesis of new ACh. – ACh may diffuse away from the synaptic cleft, & is broken down by enzymes in the blood. 2 major types of ACh receptors: nicotinic (a ligand-gated ion channel, permeable to both Na+ & K+) & muscarinic (couple with G proteins). Nicotinic receptors in the brain (part of brain’s reward pathways) are important in cognitive functions & behavior. Catecholamine Biosynthetic Pathway Tyrosine hydroxylase Dopamine & NE: more common CNS catecholamine NT Adrenergic fibers: neurons that release E or NE Noradrenergic fibers: neurons that release NE Catecholamines Made from tyrosine; rate-limiting is tyrosine hydroxylase. [Catecholamine] at the synapse is reduced by: – The action of enzymes (such as monoamine oxidase) which occur both in the extracellular fluid & the axon terminal. – Active reuptake by the axon terminal. 2 major types of receptors for NE & E: – a-adrenergic receptors (a-adrenoceptors) – b-adrenergic receptors (b-adrenoceptors) Receptor types & subtypes can be distinguished by the effect of various drugs & their 2nd-messenger systems (all are metabotropic). Play essential roles in states of consciousness, mood, motivation, directed attention, movement, blood-pressure regulation & hormone release. Serotonin (5-hydroxytryptamine, 5- HT) Made from tryptophan (essential a.a.). Funtions as a neuromodulator (its effects have a slow onset). Many receptor types have been identified. Serotonergic pathways are involved in control/regulation of muscles, sleep/wakefulness, food intake, reproductive behavior, & emotional states (such as mood & anxiety). Circuits in the central region of the brain in which neurons that communicate with a particular NT are in high concentration. Goodenough, Wallace, McGuire: Human Biology Amino Acid NT Excitatory a.a. (glutamate & aspartate) – Major excitatory NT in the CNS, & most CNS neurons respond to them. – Two types of receptors: ionotropic (AMPA & NMDA, together involved in LTP) & metabotropic. – Function in learning, memory, & neural development. – Implicated in several neurodegenerative diseases. Inhibitory a.a. (GABA & glycine) – Major inhibitory NT in the CNS, & most CNS neurons respond to GABA. Neuropeptides NT Neurons that release 1 or more NP are called peptidergic. Many NP have been identified, the physiological functions of some are not known. Of particular interest are the endogenous opioids (endorphins & enkephalins): their receptors are the sites of action of opiate drugs (such as morphine & codeine), which are powerful analgesics. – Endogenous opioids also play a role in eating & drinking behavior, in regulation of the cardiovascular system, & in mood & emotions. Gases- Nitric Oxide (NO) Serves as a NT between some neurons, & between neurons & effector cells. Produced from arginine by the action of NOS NO activates guanylyl cyclase in target cells Þ cGMP Plays role in learning, development, drug tolerance, & sensory & motor modulation. Also implicated in neural damage that results from stoppage of blood flow to the brain. NO is also released by nonneural cells, & plays an important paracrine role in the cardiovascular & immune systems. Structure of the Nervous System Nerve- A group of many nerve fibers (axons) traveling to the same general location in the PNS. Pathway or tract- A group of many nerve fibers (axons) traveling to the same general location in the CNS. Ganglion- A group of neuron cell bodies clustered together in the PNS. Nucleus- A group of neuron cell bodies clustered together in the CNS. CNS= Spinal Cord + Brain PNS= Spinal Nerves (31 pairs) + Cranial Nerves (12 pairs) The spinal cord & divisions of the brain The four interconnected ventricles of the brain (a) Side-to-side section of the brain. (b) The dashed line indicates the location of the x-section in (a). A lateral view of the brain. The outer layer of the forebrain (the cortex) is divided into four lobes. Figure 6-35 Examination of the laminated organization of neurons and other cells in the cerebral cortex reveals six layers involved in the integration of afferent and efferent signal. Functions of the limbic system: learning emotion appetite (visceral function) sex Figure 6-36 endocrine integration afferent neurons efferent neurons Anterior view of one vertebra and the nearby section of the spinal cord. Figure 6-37 C = cervical Dorsal view T = thoracic of the spinal cord. L = lumbar S = sacral Figure 6-38 Ref.: Human Physiology: An Integrated Approach 1998. Silverthorn. Prentice Hall. Divisions of the Peripheral Nervous System I. Afferent division II. Efferent division A. Somatic nervous system B. Autonomic nervous system 1. Sympathetic division 2. Parasympathetic system 3. Enteric system PNS: Somatic & Autonomic Divisions Somatic 1. Consist of a single neuron bet. CNS & skeletal muscle cells 2. Innervates skeletal muscle 3. Can lead only to excitation Autonomic 1. Has 2 neuron chain bet. CNS & effector organ 2. Innervates smooth & cardiac muscle, glands, & GI neurons 3. Can be either excitatory or inhibitory Divisions of the PNS Relationship between a sympathetic trunk & spinal cord (1-5) with the various courses that preganglionic fibers (solid lines) take through the sympathetic trunk. Dashed lines represent postganglionic fibers. A mirror image of this exists on the opposite side of the spinal cord. Skeletal Voluntary muscle command: Motor neuron contraction Move! Heart, smooth Involuntary muscle, command: glands, Rest & digest. many “involuntary” targets” Involuntary Heart, command: smooth Emergency! muscle, glands, many “involuntary” targets” Figure 6-42 Classes of Receptors for Acetylcholine (ACh), Norepinephrine (NE), & Epinephrine (Epi) Receptors for ACh a. Nicotinic receptors - On postganglionic neurons in the autonomic ganglia - At neuromuscular junctions of skeletal muscle - On some CNS neurons b. Muscarinic receptors - On smooth & cardiac muscle, gland cells - On some CNS neurons - On some neurons of autonomic ganglia Receptors for NE & Epi - On smooth & cardiac muscle, gland cells - On some CNS neurons The ventricular system of the brain & the distribution of the cerebrospinal fluid (shown in blue). The cerebrospinal fluid (CSF) is a filtrate of the blood. It flows through the four ventricles shown here and also along the length of the spinal cord before being reabsorbed into the blood. Figure 6-43 Brain Blood Supply & the Blood-Brain Barrier Under normal conditions, glucose is the only substrate metabolized by the brain to provide energy. – The brain is absolutely dependent on a continuous blood supply of glucose & O2. – If blood supply to a region of the brain is ¯ or stopped (a condition called stroke), neurons cease to function & die. The blood-brain barrier, formed by tightly sealed endothelial cells of brain capillaries, plays key role in: – Regulating the chemical composition of the EC fluid of the brain – Minimizing the ability of harmful substances to reach the brain. The Blood-Brain Barrier Silverthorn: Human Physiology- An Integrated Approach