Bio Topics PDF
Document Details
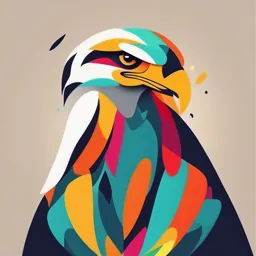
Uploaded by GenuineGyrolite917
Tags
Summary
This document provides an overview of biological topics, including different types of reactions, various biological molecules such as carbohydrates, lipids, and proteins, and their functions. It also discusses the structure and function of various biological molecules and their roles in living organisms.
Full Transcript
CHAPTER 1: Different types of reactions: 1. Dehydration reactions: larger molecules join by the removal of water 2. Hydrolysis reactions: water is used to split a large molecule into 2 smaller molecules (reverse of dehydration) 3. Neutralization reaction: occurs between acids an...
CHAPTER 1: Different types of reactions: 1. Dehydration reactions: larger molecules join by the removal of water 2. Hydrolysis reactions: water is used to split a large molecule into 2 smaller molecules (reverse of dehydration) 3. Neutralization reaction: occurs between acids and bases to produce salts 4. Redox reactions: electrons are lost from one atom and gained by another Oxidation: the loss of electrons/the transfer of an entire hydrogen atom Oxidizing agent: the molecule that gains the electrons Reduction: the gain of electrons Reducing agent: the molecule that loses the electrons Hydrogen bonds: strong attraction between water molecules - a force between molecules, not a chemical bond Buffers: a chemical that compensates for pH changes in a solution by accepting or donating H+ ions Makes Up Most Cells: Carbon, hydrogen, oxygen and nitrogen make up 99% of the mass of most cells. Bonds: 1. Glycosidic bonds: bring together carbohydrates 2. Ester linkages: bring together lipids 3. Peptide bonds: bring together proteins Organic compounds: Carbon compounds Organized into chains/rings Contain carbon-hydrogen bonds (good source of energy) Usually found in and produced by living organisms (carbohydrates & proteins) Living systems Inorganic compounds: Pure carbon and carbon compounds No hydrogen No carbon-hydrogen bonds Not organized into chains/rings Living systems Carbon: Has 4 valence electrons Can bond with 4 other atoms in covalent molecules Usually bonds with hydrogen, oxygen, nitrogen, phosphorus, sulfur or carbon Can form straight & branched chains and rings Functional groups: Attached groups of bonded atoms to complex molecules. Compounds can have more than one attached. Hydrophilic Polar except phosphate groups Increase the organic molecules’ solubility in water Can change the chemical properties of the organic molecule it’s bonded to Each has a specific role in cell metabolism The 6 Types of Groups: 1. Hydroxyl 2. Carbonyl 3. Carboxyl 4. Amino 5. Sulfhydryl 6. Phosphate Hydroxyl: alcohols with names ending in -ol EX: Ethanol Carbonyl: 1. Aldehydes: the carbonyl group is at the end of the carbon skeleton 2. Ketones: the carbonyl group is within a carbon skeleton Carboxyl: carboxylic acids or organic acids EX: Acetic acid in vinegar Amino: amino acids EX: Glycine Sulfhydryl: Sulfur containing amino acids Phosphate: organic phosphates EX: Glycerol phosphate Macromolecules: 1. Carbohydrates - energy course for cellular respiration 2. Lipids - energy storage, insulation, and membrane structure 3. Proteins - muscle development, enzymatic reactions 4. Nucleic acids - genetic information and protein synthesis ★ Molecular structure and function are inseparable Carbohydrates functions: 1. Energy 2. Structures (form plants cell walls) Types: 1. Monosaccharides Aldoses Ketoses 2. Disaccharides 3. Polysaccharides Monosaccharides: the primary energy molecule of life Contain only carbon, hydrogen and oxygen (Glucose) Classified by: 1. The location of the carbonyl group (as aldose or ketose) 2. The number of carbons in the carbon skeleton Aldehyde: double bonded oxygen at the end (Glucose) Ketone: double bonded oxygen not at the end (usually on the second carbon - fructose) Disaccharides: made of two monosaccharides covalently bonded by dehydration synthesis Weak bond (glycosidic/ester linkage) Dehydration synthesis: Water is formed and released as subunits combine Polysaccharides: composed of hundreds of glucose monomers Functional & structural roles determined by its sugar monomers and the positions of glycosidic linkages Storage 4 types 1. Starch/amylose: energy storage (made by plants) 2. Glycogen: animal starch (stored in muscle & liver) 3. Cellulose: produced by plants for cell wall structure (humans can’t digest) 4. Chitin: animal cellulose (used to make exoskeletons & fungi cell wall) Molecular isomers: organic compounds with the same molecular formula but different structures Lipids: Do not form polymers Hydrophobic Fats, phospholipids, steroids Digested to glycerol (used in glycolysis) and fatty acids (used in generating acetyl-CoA) Fats: Constructed from glycerol and fatty acids 3 fatty acids are joined by an ester linkage to create a triglyceride Major function = energy storage (provide more energy than carbohydrates) Saturated fatty acids: have the maximum number of hydrogen atoms possible and no double bonds. Saturated fats are solid at room temperature Unsaturated fatty acids: have 1+ double bonds. Unsaturated fats are liquid at room temperature Hydrogenation: converting unsaturated fats to saturated fats by adding hydrogen Phospholipids: 2 fatty acids and a phosphate group are attached to glycerol Fatty acid tails are hydrophobic Phosphate group forms hydrophilic head Major component of cell membranes Steroids: Have carbon skeletons consisting of 4 fused rings Cholesterol Waxes: Large with long fatty acid chains linked to alcohols or carbon rings Hydrophobic Non polar Ideal for waterproof coatings Helps conserve water in plants & prevent infections Proteins: account for more than 50% of the dry mass of most cells. Consists of 1+ polypeptides Must be digested to amino acids; amino groups can feed glycolysis or the citric acid cycle Polypeptides: polymers built from the same set of 20 amino acids. Each has a unique linear sequence of amino acids that determines its function Modified to form functional proteins (protein synthesis) Amino acids: organic molecules with carboxyl and amino groups, differ in functions due to R groups (side chains). Amino acid polymers are linked by peptide bonds. Protein functions: Structural support Storage Transport Cellular communications Movement Defense Enzymes/catalysts (speed up chemical reactions) Functional proteins: consists of 1+ polypeptides twisted, folded and coiled into a unique shape 4 levels of protein structure: 1. Primary structure: unique sequence of amino acids (coils and folds in polypeptide chain) 2. Secondary structure: linear amino acid sequence begins to coil, creating a spiral shape as a result of hydrogen bonds. Folds over with the help of chaperones. 3. Tertiary structure: determined by R group interactions attached by peptide bonds 4. Quaternary structure: the backbone of the protein made up of polypeptide chains comes together to form one large macromolecule Sickle-cell disease: an inherited blood disorder that changes the primary structure, affecting its function Protein structure determinants: Alterations in pH Salt concentration Temperature Can cause unravelling and denaturation if the protein’s native structure is lost. Denatured protein = biologically inactive Nucleic acids: Polynucleotides made of nucleotides containing a nitrogenous base, a pentose sugar and a phosphate group 1. DNA Provides directions for its own replication Directs the synthesis of mRNA (controls protein synthesis occurring in ribosomes) 2. RNA Passes through nuclear pores Nitrogenous bases: adenine, guanine, cytosine & thymine/uracil (2 types) A&T, G&C 1. Pyrimidines: cytosine, thymine, uracil (single six-membered ring) 2. Purines: adenine & guanine (six-membered ring fused to a five-membered ring) Chemical reactions: need initial input of activation energy (transition state) ★ increased temperature = faster reaction (molecules move faster) Enzymes can increase reaction rate without increasing temperature by lowering the activation energy (they create a new reaction pathway) Caloric content: Carbs: 4.1 kcal/gram Proteins: 4.3 kcal/gram Fats: 9.1 kcal/gram Enzymes: Proteins with 3D globular structure Have an active site (depression on the enzyme) & a substrate (reactants that are activated by the enzyme) Cofactor: a non-protein group that binds to an enzyme and is essential for catalytic activity Coenzyme: an organic molecule that acts as a cofactor of an enzyme ★ Enzymes are specific to their substrates, determined by the active site Induced fit hypothesis: proteins change shape when substrate combines with the active site so it is moulded perfectly to fit (makes the chemical environment suitable for the reaction). Makes the reaction easier by lowering the activation energy. 1. Enzymes adjust their shape to accommodate the substrate 2. The combination of the enzyme and substrate forms a compound called a enzyme-substrate complex 3. The enzyme-substrate complex is held together by hydrogen bonds and weak ionic bonds 4. These attractions lock the substrate molecule into the active site 5. Once in the active site, is subject to necessary collisions, bonds breaks, and bond formations that must take place to form the product molecule (transition state) 6. Once the product molecule is formed, it is released from the enzyme-substrate complex 7. The enzyme is now able to accept another substrate and begin the process again Factors affecting enzymes (increasing or decreasing enzyme rate): 1. Substrate (increases)/enzyme concentration (proportional): amount that can be reacted 2. pH (decreases): extreme levels will cause denaturation 3. Temperature (increases): the rate of the reaction doubles or triples with every 10℃ rise in temperature (Q10 = temperature coefficient). High temperatures can cause denaturation (usually 70℃). Optimum temperature is usually 30℃. 4. Inhibitors: chemicals that reduce the rate of enzymatic reactions (block the enzyme) Effects of enzyme inhibition: 1. Irreversible inhibitors: combine with the functional groups of the amino acids in the active site, irreversibly 2. Reversible inhibitors: can be washed out of the solution of enzyme by dialysis Competitive: compete with the substrate molecules for the active site (resembles the substrate) Non competitive: binds irreversibly to the enzyme but not at the active site Allosteric: other site that fits the inhibitor molecule instead of the substrate like the active site CHAPTER 2: Eukaryotic cells contain: 1. DNA with a membrane-bound nucleus 2. Cell membrane with a phospholipid bilayer with embedded proteins and lumen between the layers 3. Cytoplasm filling the interior and consisting of organelles, cytosol and molecules Nucleus: Filled with nucleoplasm Surrounded by the nuclear membrane Controls cellular activities by regulating gene expression Nucleolus contains: 1. RNA 2. Protein 3. Chromatin - Site of ribosome production Endoplasmic reticulum (ER): connects to the nucleus and has 2 parts 1. Rough ER: membrane-bound sacs associated with ribosomes which synthesize proteins (enzymes and insulin) intended for export from the cell or for membrane formation 2. Smooth ER: synthesizes lipids and does not have ribosomes associated with it The endomembrane system: the nuclear envelope, the endoplasmic reticulum, the golgi apparatus, and vesicles act as a transportation and product-processing component. This is where proteins are synthesized and modified. Lysosomes also break down macromolecules and are produced by the golgi apparatus. Golgi apparatus: sorts, packages and distributes lipids and manufactures macromolecules - modifies proteins / lipids (adding carbs to form glycoproteins) Chloroplast: contains chlorophyll - helps with photosynthesis Mitochondria: break down energy-rich molecules to convert stored energy into useable energy - produces ATP with cellular respiration Flagella: 1 or 2 long tails that whip on the outside of some eukaryotic cells Cilia: Many short appendages on the outside of some eukaryotic cells that move the cell with wave-like motions The fluid mosaic model: explains the cell membrane as a semi fluid phospholipid bilayer with a mosaic of proteins. The two leaflets can slide across each other and individual phospholipids exchange places frequently - membrane proteins can function as receptors, enzymes, or channels Degree of fluidity (of the phospholipid bilayer): crucial to the proper functioning of the cell. Affected by: 1. Temperature: too high = too porous, too low = barrier 2. Presence of double bonds in the fatty acid tails: more fluid because less tightly packed 3. Length of the fatty acid tails: longer = shorter intermolecular attraction 4. Presence of cholesterol: reduces fluidity at room temperature, at cold temperatures keeps the bilayer from solidifying Types of transport: 1. Diffusion: the net movement of ions/molecules from an area of high concentration to an area of low concentration. Rate is affected by: Molecule size (bigger = slower) Molecule or ion charge Molecule polarity (polar = slower) Temperature & pressure (increase in both = faster) 2. Passive transport: some substances do not require energy to move across the semipermeable cell membrane because they move down their concentration gradient By diffusion By osmosis: the movement of water molecules across a semipermeable membrane. Water moves along its concentration gradient until the concentrations on each side of the membrane are equal By facilitated diffusion: when molecules that cannot pass through the cell membrane unassisted are helped through by channel or carrier proteins. Moves along the concentration gradient - EX) oxygen and carbon dioxide diffusion Channel proteins: highly specific hollow cylinders. Shape and size of the structure determine the particles that can pass through Carrier proteins: bind to specific molecules and carry them across the membrane then release them. Can transport large molecules but are slower than channel proteins 3. Active transport: the transport of a solute across a membrane against its concentration gradient requiring ATP energy Primary: carrier proteins acting as ion pumps using ATP energy to pump ions from one side of the membrane to another Secondary: when an ion pump actively moves charged ions against the concentration gradient and builds up an electrochemical gradient on one side of the membrane to transport molecules or ions (hydrogen-sucrose pump) - EX) sodium-potassium pump 4. Membrane assisted transport: a cell forms a vesicle to surround incoming/outgoing material, requiring energy, to transport large macromolecules Endocytosis: moving materials into the cell 1. Phagocytosis: a cell engulfs a large particle along with some of the liquid surrounding it 2. Pinocytosis: a cell engulfs a liquid and the small particles dissolved/suspended in it 3. Receptor-mediated endocytosis: receptor proteins in the cell membrane bind to specific molecules outside the cell - EX) uptake of nutrients or pathogens like white blood cells Exocytosis: moving materials out of the cell. Large molecules/waste leave a cell by way of vesicles, which then become part of the cell membrane - EX) secretion of neurotransmitters or hormones Types of energy: 1. Kinetic energy: the energy of motion EX: falling water 2. Potential energy: stored energy EX: a molecule of glucose has stored energy in its bonds 3. Chemical energy: the potential energy stored in a molecule’s bonds EX: the breakdown of glucose or ATP molecules releases chemical energy which can be used to fuel chemical activities in cells Thermodynamics: the study of the flow of energy to study metabolic processes and the energy transformations that take place within living systems Types of systems: 1. Open system: a system where matter/energy can be exchanged with the surroundings 2. Closed system: a system isolated from the environment Laws of thermodynamics: how energy flows 1. The law of conservation of energy: energy can neither be created nor destroyed, but can be transformed from one form to another 2. The second law of thermodynamics: energy can not be transformed from one form to another without a loss of useful energy. (energy is lost and escapes as waste thermal energy) Predictions with Laws of Thermodynamics: The laws of thermodynamics allow us to predict whether a given chemical or physical change can occur without the continuous input of additional energy. If the △G value is negative, the reaction has released free energy and can proceed without an outside source of energy Spontaneous change: a change that will continue to happen on its own without a continuous input of energy EX: burning of a match Depends on: Energy change Entropy Temperature ★ A positive △G value = non spontaneous Gibbs free energy: energy that can do useful work - the energy that is not lost during an energy transformation - Negative △G: spontaneous reactions (exergonic) - Positive △G: non-spontaneous reactions (endergonic) Types of Reactions: Exergonic reaction: releases free energy (negative △G value) Endergonic reaction: absorbs free energy (positive △G value) Transition state: a temporary condition during a chemical reaction in which the bonds in the reaction are breaking and the bonds of the product are forming Exothermic reaction: net release of energy (reactants chemical potential energy > products) Endothermic reaction: net absorption of energy requiring an initial input of energy to proceed (products chemical potential energy > reactants) CHAPTER 3: Metabolism: the collective name for all the reactions that occur inside the cell Coupled reaction: the energy released by an exergonic reaction (greater amount) can be used to fuel an endergonic one (lesser amount) EX: ATP hydrolysis provides energy for muscle contractions or the Na/K pump Two Pathways: Catabolic pathway: complex molecules break down into simpler compounds, releasing energy (negative △G value) EX: cellular respiration Anabolic pathway: consumes free energy to build complicated molecules from simpler molecules (positive △G value) EX: Biosynthesis (not all you eat gets used for cellular respiration) or photosynthesis Uses for ATP in cells: 1. Chemical work: energy to synthesize macromolecules 2. Mechanical work: energy needed to permit muscles to contract, cilia and flagella to beat, chromosomes to move etc. 3. Transport work: supplies energy to pump substances across the cell membrane Advantages of ATP: 1. Used in many different cellular chemical reactions 2. When it is broken down to ADP + P some energy is used in reactions to make more energy 3. ATP breakdown is coupled with endothermic reactions to minimize energy loss. Some energy is released and used in endothermic reactions. Energy coupling: ATP is moved into close proximity of an endergonic reaction Phosphorylation: 1. The terminal phosphate will transfer to the reactant molecule, providing free energy 2. An enzyme is required to bring the ATP to the reactant 3. The enzyme binds to ATP and the reactant in order to bring them to one another 4. In the end, a phosphate group attaches to another organic molecule and an organic molecule gains free energy and becomes more reactive - Substrate-level phosphorylation: ATP generated by transferring a phosphate group directly to ADP from a phosphorylated substrate. - Oxidative phosphorylation: ATP synthesized by ATP synthase, driven by the proton gradient in mitochondria. ATP synthesis: ATP needs to be regenerated so we can continue using it to function normally Occurs at the mitochondria’s cristae and matrix Reverse of ATP hydrolysis (ATP hydrolysis products are ADP, water and free energy) Endergonic reaction that requires energy The energy comes from breaking down the complex molecules from the food we eat or light energy EX) oxidative phosphorylation (ETC) and substrate-level phosphorylation (glycolysis) Enzymes: specialized proteins that speed up chemical reactions by lowering the amount of activation energy The catalysts of metabolic reactions Without enzymes, metabolic reactions would proceed too slow to maintain normal cellular functions - EX) Amylase: Breaks down starch into maltose. - EX) Protease (trypsin): Breaks down proteins into amino acids. Catalysis: the acceleration of a chemical reaction by some substance (the substance does not undergo permanent chemical change) Occurs at the active site (where the substrate fits) Activation energy is required for the initial bonds to break in the reactants Substrate: a reactant in any given enzymatic reaction for that specific enzyme How enzymes reduce the activation energy needed to break the bonds in a substrate: 1. Bringing the reactants in close proximity to one another and in the proper orientation to allow for collisions 2. Expose the reactant molecules to altered charged environments to place stress on the initial bonds 3. Change the shape of the substrate to create a strain that will weaken the chemical bonds Three overall goals: 1. To break bonds between 6 carbon atoms of glucose, resulting in 6 CO2 molecules 2. To move hydrogen atom electrons from glucose to oxygen, forming 6 water molecules 3. To trap as much of the free energy released in the process as possible in the form of ATP Photoautotrophs: green plants and microorganisms - photosynthesis: light energy converted to glucose Chemoautotrophs: extract energy from inorganic sources and found in extreme conditions (ie: volcanoes) - chemosynthesis: energy from inorganic compounds Heterotrophs: rely on autotrophs for energy and eat other living things ★ Total daily caloric need & calculating macros CHAPTER 4: Energy transfer is accomplished by substrate level phosphorylation Substrate level phosphorylation: a catalyzed reaction that forms ATP directly in an enzyme Oxidative phosphorylation: the potential energy by the oxidation of NADH is used to pump protons into the intermembrane space. Forms ATP indirectly through a series of enzyme-catalyzed redox reactions (O2 is used) - Aerobic respiration is more efficient than glycolysis Catabolic processes: processes used by obligate and facultative anaerobes that produce less free energy than aerobic cellular respiration 1. Anaerobic respiration: a process that uses a final inorganic oxidizing agent other than oxygen to produce energy Uses a series of electron-transferring steps 2. Fermentation: a process that uses a final organic component as the final oxidizing agent to produce energy Does not use a series of electron-transferring steps 1. Alcohol fermentation - used by yeast to produce ethanol and CO₂ 2. Lactic acid fermentation: pyruvate is reduced by NADH, forming lactate (no release of CO2) - occurs in human muscle cells under oxygen limited conditions Aerobic vs. Anaerobic Respiration: Aerobic: requires oxygen, yields 36-38 ATP per glucose, produces CO₂ and H₂O Anaerobic: does not require oxygen, yields 2 ATP per glucose, produces lactic acid or ethanol Aerobic cellular respiration: 1. Glycolysis (I & II): occurs in cytosol - anaerobic process that breaks down one glucose molecule - Energy investment phase: consumes 2 ATP - Energy payoff phase: produces 4 ATP and 2 NADH 2. Pyruvate oxidation: pyruvate molecules from glycolysis are oxidized further to form acetyl-CoA and transferred through the inner and outer mitochondrial membranes - Produces 1 CO₂, 1 NADH per pyruvate molecule. 3. Citric acid/krebs cycle: occurs in the mitochondrial matrix and 1 ATP, 3 NADH, 1 FADH₂, 2 CO₂ 4. Electron transport chain: occurs in the inner membrane of the mitochondria where NADH and FADH2 donate electrons to produce 32-34 molecules of ATP Glycolysis: Location: Cytoplasm Type: Anaerobic (does not require oxygen) Purpose: Breaks down glucose (6-carbon molecule) into two molecules of pyruvate (3-carbons each). Glycolysis Step-by-Step: Phase I: Energy Investment Phase (Preparatory Stage) Phosphorylation of Glucose: - Glucose is phosphorylated using 2 ATP molecules to form fructose-1,6-bisphosphate. - Purpose: Destabilizes the glucose molecule, making it more reactive. Cleavage: - Fructose-1,6-bisphosphate is split into two 3-carbon molecules: G3P (glyceraldehyde-3-phosphate). Phase II: Energy Payoff Phase 3. Oxidation of G3P: - Each G3P is oxidized, transferring electrons to NAD+, forming NADH. - High-energy phosphate bonds are formed. 4. ATP Formation: - Substrate-level phosphorylation generates 4 ATP molecules (2 per G3P). Net Yield of Glycolysis: ATP: 4 produced - 2 invested = 2 ATP NADH: 2 NADH Pyruvate: 2 Pyruvate Pyruvate Oxidation Location: Mitochondrial Matrix Purpose: Prepares pyruvate for entry into the citric acid cycle by converting it into acetyl-CoA. Steps: Transport into the Mitochondria: - Pyruvate enters the mitochondrial matrix via a transport protein. Decarboxylation: - One carbon is removed from pyruvate, forming CO2. Oxidation: - The remaining 2-carbon fragment is oxidized, and electrons are transferred to NAD+, forming NADH. Formation of Acetyl-CoA: - The 2-carbon molecule binds to coenzyme A, forming acetyl-CoA. Net Yield of Pyruvate Oxidation (per pyruvate): NADH: 1 NADH Acetyl-CoA: 1 Acetyl-CoA CO2: 1 CO2 (Since glycolysis produces 2 pyruvate, double these amounts per glucose.) Citric Acid Cycle (Krebs Cycle) Location: Mitochondrial Matrix Purpose: Completes the oxidation of glucose by fully breaking down acetyl-CoA. Steps: Citrate Formation: - Acetyl-CoA (2-carbons) combines with oxaloacetate (4-carbons) to form citrate (6-carbons). Isomerization: - Citrate is converted to its isomer, isocitrate. Decarboxylation Reactions: - Two carbons are removed as CO2, reducing NAD+ to NADH. ATP Production: - Substrate-level phosphorylation generates 1 ATP (or GTP) per cycle. Regeneration of Oxaloacetate: - The remaining 4-carbon molecule undergoes several steps, reducing FAD to FADH2 and NAD+ to NADH. - Oxaloacetate is regenerated to restart the cycle. Net Yield of Citric Acid Cycle (per acetyl-CoA): ATP: 1 ATP NADH: 3 NADH FADH2: 1 FADH2 CO2: 2 CO2 (Since each glucose generates 2 acetyl-CoA, double these amounts per glucose.) Electron Transport Chain (ETC) Location: Inner Mitochondrial Membrane Purpose: Uses high-energy electrons from NADH and FADH2 to produce ATP through oxidative phosphorylation. Steps: Electron Transfer: - NADH and FADH2 donate electrons to the ETC, starting at complex I (NADH) or complex II (FADH2). - Electrons are passed through protein complexes (I, II, III, IV), releasing energy. Proton Gradient Formation: - Energy from electrons pumps H+ ions into the intermembrane space, creating an electrochemical gradient. Oxygen as Final Electron Acceptor: - At complex IV, electrons combine with H+ and oxygen to form water (H2O). ATP Production: - Protons flow back into the matrix via ATP synthase, driving the phosphorylation of ADP to ATP. Net Yield of ETC (per glucose): ATP: 32-34 ATP (via oxidative phosphorylation). By-products: H2O. Uncoupling electron transport: If electron transport and ATP synthesis is uncoupled, the energy that is being released will become thermal energy instead of being converted to ATP energy by… 1. Regulating the expression of a number of uncoupled proteins Found in the inner mitochondrial membrane and allow for protons to re-enter the matrix through a specialized pathway 2. Chemical compounds form channels across the membrane of protons to leak through Components: Mitochondria: the main source of ATP molecules in cells, composed of an inner membrane and an outer membrane, separated by an inner membrane space The inner membrane folds as shelf-like cristae (increases surface area for ETC enzymes and ATP production) and contains the matrix (an enzyme-rich fluid - site for krebs cycle enzymes) Outer membrane: contains porin (a transport protein) that makes it permeable to small molecules. Also contains enzymes that help convert fatty acids to molecules that can pass into the interior of the mitochondrion to be broken down further Inner membrane: contains the multienzyme complexes and the electron carriers of the ETC and a high proportion of cardiolipin (a phospholipid that makes the membrane impermeable to ions). The impermeability is important in the production of ATP during chemiosmosis Intermembrane space: a fluid-filled area containing enzymes that use ATP. Used as a hydrogen ion (H+) reservoir that stores the hydrogen ions that will be used for ATP synthesis Transition reaction (start of pyruvate oxidation): occurs in the matrix of the mitochondria as long as oxygen is available If no oxygen is available, the pyruvate in the cytosol can be oxidized through fermentation Depolarization: the loss of the concentration gradient Early step towards cell death Slows down and stops the ETC and its production of ATP - loss of proton gradient → inability to produce ATP → energy crisis in the cell → apoptosis or necrosis CHAPTER 6: Chromosomes unravel after cell division takes place and become functional and is spread throughout the nucleus Gene: the coding region of DNA which contains instructions for building the proteins that are responsible for each inherited trait The gene that is responsible for coding the protein becomes activated when a protein needs to be produced Genome: The complete set of an organism’s hereditary information Gene vs. Genome - gene: functional unit of heredity coding for a protein or RNA - genome: includes all genetic material (coding and non-coding regions). The transformation principle: 1. One bacteria takes up DNA from a dead cell of the same species or related species 2. There is a decrease in the population 3. There is an increase in the genetic variety Horizontal gene transfer: the smooth strain (virulent) DNA is transferred to the rough strain (non-virulent). Heat can kill the infection in the smooth strain, but when that is mixed with the rough strain, it stays virulent and the mouse dies. DNA structure: made up of two strands of repeating DNA nucleotides (a phosphate group, a deoxyribonucleic acid, and a nitrogen base) that run in anti-parallel directions - Antiparallel: strands are antiparallel (leading strand read 5’ to 3’ orientation; opposite to the lagging strand read 3’ to 5’ direction) The sugar and phosphates alternate to make up the rails of the ladder Hydrophobic nitrogen bases connect at the center creating the rungs of the ladder (space between = 0.34nm) Strands are complementary Base-Pairings: adenine pairs with thymine (A-T) via two hydrogen bonds, and cytosine pairs with guanine (C-G) via three hydrogen bonds Molecular stability: 3 forces that stabilize the DNA molecule 1. The phosphate bridges link the sugar-phosphate handrails 2. Hydrogen bonds between base pairs keep the two strands together in the helix 3. Hydrophobic and hydrophilic reactions cause the bases to remain on the inside of the molecule and the handrails to face out into the watery nucleus of the cell DNA vs. RNA: 1. RNA sugar = ribose, DNA sugar = deoxyribose 2. RNA = uracil (U), DNA = thymine (T) 3. RNA = single stranded, DNA = double stranded DNA replication and repair: 1. During cell division in eukaryotic cells, replicated genetic material in nucleus divided equally between two daughter nuclei via mitosis 2. Followed by cytokinesis in which the cell is split into two new cells Essential for growth, regeneration, and repair of tissues 3. Hydrogen bonds between complementary bases can break, allowing the DNA helix to unzip 4. Each strand then acts as a template to build the complementary strand 5. Results in two identical DNA molecules (one for each daughter cell) Semiconservative replication: Each of the two strands of parent DNA is incorporated into a new double-stranded DNA molecule DNA replication: 1. DNA cannot just be pulled apart by hydrogen bonds, two parent DNA strands must be unravelled and kept separate 2. Enzymes work together to expose the DNA template strands 3. Topoisomerse relieves tension caused by the unwinding of DNA 4. DNA helicase breaks hydrogen bonds between complementary base pairs (unwinding) 5. Two individual strands are kept apart by single-stranded binding proteins (SSBs) 6. SSBs bind to exposed DNA single strands and block hydrogen bonding 7. Replication begins in two directions from origins as a region of DNA is unwound 8. Complementary strands are built as soon as an area of DNA has been unwound 9. DNA replication proceeds toward direction of replication fork on one strand and away from the fork on the other strand 10.The leading strand writes in the 5’ -> 3’ prime direction 11.It is read in the 3’ -> 5’ direction Components: Replication fork: the place where the two disrupted DNA strands are still joined Leading strand is copied in the direction toward the replication fork Lagging strand is copied in the direction away from the replication fork Replication bubble: forms when two replication forks are near each other RNA primer: marks initiation sequence/acts as a starting point DNA ligase: catalyzes the formation of a phosphodiester bond between two DNA strands and Okazaki fragments Okazaki Fragments: Highlight that these are short DNA fragments formed on the lagging strand during replication. Primase: synthesizes RNA primers to initiate DNA strand elongation. DNA polymerase I, II, III: the three enzymes known to function in replication and repair and proofread new strands DNA polymerase I fills in gaps in the lagging strand between Okazaki fragments DNA polymerase III builds new DNA strands from nucleotides and functions only under certain conditions - adding nucleotides in the 5’ to 3’ direction during replication and proofreading for errors to maintain proper formation Requires an initial starting 3’ end to start elongation DNA’s primary and secondary structure in relation to DNA function: 1. How DNA is organized in the cell 2. How its genome is arranged into distinct functional regions of DNA (genes and non-coding regions) DNA of prokaryotic cells: Bacterial chromosomes consist of a circular, double-stranded DNA molecule Some do not have a nuclear membrane Most are haploid (one set of chromosomes/one copy of each gene) Packing DNA: DNA must be tightly packed so it can fit in the nucleoid. This is done by coiling, compacting and supercoiling The circular chromosomal DNA molecule can be compacted through the formation of looped structures The looped DNA can be further compacted by DNA by supercoiling Supercoiling: the formation of additional coils in the structure of DNA by twisting forces - Controlled by the enzymes topoisomerase I and II Plasmids: small circular or linear DNA molecules that carry non-essential genes (not part of the nucleoid) DNA of eukaryotic cells: Double stranded & linear Tightly compacted Greater amount than prokaryotic (greater compacting) Histones: a family of proteins that associate with DNA Telomeres: the caps at the end of each strand of DNA that protect our chromosomes and vital information in our DNA and affect how our cells age (get shorter as we age, smoke, eat poorly) CHAPTER 7: The central dogma explains the process of how the linear arrangement of nucleotides containing the information in the DNA could be transformed into three-dimensional proteins made of polypeptides. The central dogma: the two step process by which genes are expressed and proteins are built, going from a gene in the DNA to a strand of RNA, then into a polypeptide chain for a specific protein to be formed while reserving as much energy as possible A strand of DNA serves as the template for the construction of a complementary strand of RNA This strand of RNA then moves from the nucleus to the cytoplasm where it guides the synthesis of a polypeptide chain Transcription: the synthesis of a RNA molecule from a strand of DNA. The nucleotide sequence of the DNA strand is copied into the nucleotide sequence of the synthesized RNA molecule Translation: The language preserved in the RNA must be converted to the amino acid sequence of a polypeptide in order for a protein to be synthesized Advantages of two steps for gene expression: 1. Saves cell energy by not transporting the whole chromosomes from the nucleus and back again 2. Protects DNA material from risk associated with moving DNA around the cell 3. A single stretch of DNA can also be copied many times over to produce a large number of RNA molecules (multiple copies help speed up protein synthesis) 4. Gives opportunities to regulate gene expression Types of RNA: involved in protein synthesis 1. Messenger RNA (mRNA): acts as the intermediary between DNA and the ribosomes (RNA version of the gene encoded by DNA) 2. Transfer RNA (tRNA): transfers the appropriate amino acid to the ribosome to build a protein 3. Ribosomal RNA (rRNA): structural component of a ribosome tha bonds the correct amino acid to the polypeptide chain Genetic code characteristics: 1. Continuity: the letter codons have no spaces and never overlap. Knowing exactly where transcription and translation start is essential 2. Grouping of codons: if reading frame on an RNA molecule is shifted (insertion/deletion) there is no mechanism in the cell that can reset/correct the translation 3. Redundancy: there are only 3 RNA codons that do not code for any amino acids and instead stop protein synthesis Universality: RNA codons correspond to the same amino acids in almost all organisms that have genetic material (exception for mitochondria, chloroplasts, and unicellular eukaryotes) Provides evidence that all these organisms share a common ancestor A gene that is taken from one kind of organism and inserted into another can still express the same polypeptide Transcription: only proceeds in one direction - assists RNA polymerase in binding to the promoter region 1. Initiation: locates the correct spot on the original DNA template where transcription is to begin 2. Elongation: copies the correct number of nucleotides from the DNA template onto messenger RNA 3. Termination: signals the right place to stop the copying process to make sure the mRNA molecule contains the complete set of instructions from the gene 4. Post-transcriptional modifications: the mRNA transcript undergoes some additional processing/final changes before it is transported from the nucleus to the cytoplasm of a eukaryotic cell - enhance mRNA stability and support Promoter sequence/TATA box: a particular nucleotide sequence on the DNA molecule that provides a binding site for RNA polymerase Usually rich in T and A nucleotides Must be in correct locations RNA polymerase: the main enzyme that catalyzes the synthesis of mRNA Will only bind in one direction Processing of mRNA transcript: in eukaryotes, the mRNA molecule that is released when transcription ends is called precursor mRNA/pre-RNA Pre-mRNA cap and tail: each end of the pre-mRNA molecule undergoes a change Functions: Help protect the finished mRNA molecule from enzymes Help bind the molecules that synthesize proteins Plays a role in transporting the finished mRNA molecule from the nucleus to the cytoplasm mRNA splicing: the RNA polymerase does not distinguish between introns and exons as it transcribes a gene Intron: non-coding sequence of DNA/RNA Exon: a sequence of DNA/RNA that codes for part of a gene Splicing: the initial mRNA transcript contains long stretches of nucleotides that must be removed before the transcript is used to construct a polypeptide (splicing act) 1. The spliceosome cleaves the pre-mRNA at the ends of each intron and then splices the remaining exons 2. The removal of introns does not always follow the same pattern in any given gene 3. A stretch of nucleotides that is retained as an exon in one pre-mRNA transcript may be removed from another 4. As a result, a single eukaryotic gene can code for a variety of different protein products 5. The finished mRNA molecule is transported from the nucleus to the cytoplasm, where it begins the process of translation SnRNPs: proteins that are joined to nucleic acids of a type of RNA Anticodon: contains a nucleotide triplet with a sequence that is complementary to the codon of the mRNA molecule Found at the end of one lobe of tRNA Across from this lobe is an amino acid attachment site that binds the particular amino acid specified by the mRNA codon Activating enzymes: the genetic code-breakers/chemical translators of the cell Responsible for attaching the correct amino acid to the tRNA molecule with the correct anticodon Have 2 binding sites; one recognizes the anticodon, one recognizes the amino acid The enzyme catalyzes a reaction that attaches the amino acid to the hydroxyl group at the 3’ end of the tRNA molecule The aa-tRNA molecule is released Ribosomes: a complex that contains a cluster of different kinds of proteins together with rRNA Has two subunits (one big one small) that fit together to build an active ribosome Has a binding site for the mRNA transcript and 3 binding sites for tRNA molecules (P, A, E) Bring together the mRNA strand, the aa-tRNAs, and the enzymes involved in the building of polypeptides The site for protein synthesis within the cytoplasm P site: holds one aa-tRNA and the growing chain of amino acids A site: holds the tRNA bringing the next amino acid to be added to the chain E site: releases the tRNA molecules back into the cytoplasm Translation: a cell must translate mRNA codons into amino acid sequences 1. Initiation 2. Elongation 3. Termination Condons: - Start codon: AUG - Stop codon: UAA, UAG, UGA Prokaryotes vs. Eukaryotes: Prokaryotic cells have an extra advantage in accelerating the rate of protein synthesis Prokaryotic cells mRNA transcript extends directly into the cytoplasm of the cell as it is being formed (ribosomes can bind to the mRNA even before transcription is complete) In eukaryotic cells, transcription and translation are physically separated by the nucleus Polypeptide to protein: The polypeptide is not functional until it is folded into the correct shape Enzymes manipulate the polypeptide into its functional shape Some proteins require multiple polypeptide subunits in order to become functional Gene expression: regulated by the concentration of lactose and tryptophan Lac operon: a cluster of 3 genes that code for the proteins involved in lactose metabolism Consists of: 1. A promoter: where transcription begins 2. An operator: the sequence of bases that controls transcription 3. Coding regions: areas that code for the various enzymes that metabolize lactose 4. Repressor protein: a protein that binds to the operator to repress gene transcription (regulates the production of lac proteins) Lactose present: the lactose molecule will bind to the lac repressor and cause it to become inactive Lactose not present: binds to the operator and stops lactose-metabolizing enzymes from being synthesized Control points in protein synthesis: 1. Transcriptional control: the cell can speed up/slow down the transcription of mRNA from the gene that codes for the polypeptide 2. Post-transcriptional control: the cell may transcribe the mRNA but break it down before translation. May change the length of the tail or control the time that the mRNA is stable/active for 3. Post-translational regulation: after synthesizing the polypeptide, the cell may modify it chemically or change the rate at which it becomes a functional protein Operon: a stretch of DNA that contains a set of one or more genes involved in a particular metabolic pathway, along with an operator Operator: a DNA sequence located within the promoter sequences (control element) Repressor: makes it impossible for RNA polymerase to bind to the promoter Negative gene regulation: a protein molecule interacts directly with the genome to turn off gene expression Mutations: Point mutation: Substitution/insertion/deletion of 1+ nucleotide (EX: sickle cell anemia) Missense mutation: beneficial or harmful Spontaneous mutations: result from molecular interactions Induced mutations: caused by agents outside the cell Mutagens: increases the rate of mutation in an organism 1. Physical mutagen: outside force that tears through a DNA strand 2. Chemical mutagen: molecules that can enter the cell nucleus and induce mutations Transcription and translation occur if a virus’s genome is composed of DNA (different manner if it’s RNA) Retrovirus: a virus that uses reverse transcriptase for replication Reverse transcriptase: a viral enzyme that uses RNA as a template strand to synthesize complementary DNA Vectors: a virus that is used to transfer the DNA from a donor to a host (transduction) Central Dogma Process: Follows two main steps: transcription and translation, in order to go from a gene to a protein Transcription - first step, occuring in the nucleus, and follows four sub-steps in order to go from a DNA strand into an RNA strand Translation - occurs in the cytoplasm, where it takes the RNA strand and creates a polypeptide chain in three sub-steps to form a protein Transcription: Initiation: RNA polymerase binds to the necessary DNA strand, forming a bubble shape in order to split the double helix into two single strands at specific point, known as the promoter sequence process of copying down the information is similar to DNA synthesis, having the RNA polymerase binding to the promoter sequence, known as the TATA box, to start at a proper sequence order Elongation: follows a second set of unwinding, using the RNA polymerase to follow down the sense strand of the DNA, since it can only copy the nucleotides in one direction of 5' to 3' since it only needs one strand, there is no need for okazaki fragments like there would be in DNA synthesis process of copying down the necessary nucleotide bases continues until the RNA polymerase reaches a terminator sequence Termination: once the signal is given to stop elongation, the RNA polymerase cleaves from the DNA strand and allows it to reform its double helix, while the RNA strand also cleaves from the DNA strand as mRNA before it continues in this process, the mRNA is known as pre-mRNA, which is the most vulnerable state of the genetic information throughout the whole process Post-Transcriptional Modifications: uses caps and tails to protect the ends of the RNA strand On the 5' end of the RNA, the 5' cap, which is a strand of 7 G nucleotide bases, is connected while the poly-A-tail, a strand of A nucleotide bases, connects onto the 3' end - caps and tails protect the ends of the RNA strand that are susceptible to being broken down by an enzyme or protein, and prevent the code from being returned to their original base forms. - the poly-A-tail aids in directing the mRNA strand towards the cytoplasm Further into this step, spliceosomes are used to cleave out the non-coding sequences, known as the introns, while keeping the exon sequences, which contain all the genetic coding information. - IMPORTANCE: transcription process doesn't have a proofreading step, which causes unnecessary information to be contained in the strand. With the method of splicing the introns, it saves the cells energy by ensuring only the vital information is present in the RNA strand. Translation: Initiation: commenced as soon as the mRNA strand reaches the cytoplasm Once outside the nucleus, it binds to a small ribosomal subunit in order to begin further formation On ribosomal sub-unit, the mRNA strand is now binded to the ribosome, beginning the process of transferring its information into the proper form of polypeptides for protein synthesis Also, the met-tRNA binds to a site on the same ribosomal sub-unit, using the methionine code to find the starting codon sequence on the mRNA to show which nucleotide the strand of RNA needs to start being processed at in order to code for the proper amino acids. Elongation: repeats four steps in a continuous and simultaneous manner: 1. The met-tRNA is transferred to the P site of the ribosomal sub-unit. 2. Then, a second tRNA, which contains the proper amino acid and the anticodon, is binded to the A site 3. Once the tRNA is met on the A site, the methionine from the first tRNA strand is cleaved off, then added onto the A site with the second tRNA strand, the amino acid, and the anticodon. 4. Once the first tRNA loses its methionine, it is then moved from the P site to the E site, where it can be cleaved and begin the process again as it finds a new methionine to bond with. - This whole process of the four steps occurs in less than 0.1 seconds in your cell. Once the second tRNA is with the amino acid, the anticodon, and the methionine, it can begin replication as the mRNA strand that originally bound to the sub-unit is read by the ribosome. The ribosome slides along the mRNA strand and copies the written code of nucleotides to create the amino acid necessary for the polypeptide chain to be formed and will continue until the anticodon on the strand is met Termination: process is stopped, the polypeptide chain separates from the ribosomal subunit, now being a fully coded polypeptide strand, but not yet functional. enzymes and other proteins work together to fold and shape the polypeptide chain into its necessary 3D shape, allowing its properties to become functional. Once it is fully formed, the process of going from gene to protein is fully completed, and the process can continuously occur in your cell. CHAPTER 8: Homeostasis: at all times of the day, during all forms of activity, the body tries to maintain a constant temperature of 37℃, 100mg/mL of glucose and pH off 7.4 (dynamic - not static because it varies) - Regulate body temp through sweating or shivering - Blood glucose changes based on eating and physical activity Negative feedback: mechanism in homeostasis whereby the output reverses the variable bringing it back to a normal state of balance EX) body temp increases, the body sweats to cool down (reverses the increase) OR body temp decreases, body shivers to warm up (reverses the decrease) Positive feedback: mechanism in homeostasis whereby the output strengthens and increases the change in the variable EX) child birth - release of oxytocin stimulates contractions, causes more oxytocin to release and continues the cycle until delivery Main components of feedback systems: 1. Sensor: when the internal environment within the body changes, it is deleted by the sensor 2. Control center: the message is relayed to the correct area within the brain 3. Effector: the brain relays the signal to the appropriate effector (organ) Cortisol: stress hormone that increases sugar/glucose in the bloodstream Can trigger the body to release extra insulin, leading the body to store extra abdominal fat (or stress eating) Pancreas: organ involved in both digestion and maintaining sugar levels Releases insulin and glucose Insulin: produced in the beta cells - Released when sugar is high - Lowers blood sugar by helping cells absorb glucose Glycogen: produced in alpha cells - Released when sugar is low - Raises blood sugar by signaling the liver to release glucose - Stored as glucose in liver and muscles Blood sugar: controlled by a negative feedback loop High sugar: insulin will target the liver, muscles and adipose tissue Low sugar: glycogen targets liver and adipose tissue to break down fat or glycogen Diabetes: Type 1: (juvenile) - people do not produce enough insulin because the immune system attaches the beta cells which makes them unable to produce it - Usually diagnosed earlier in life and requires insulin injections for life Type 2: (adult onset due to diet) - the beta cells in people’s bodies no longer respond to the hormone insulin, leading to elevated blood glucose - Increases chances of obesity Hyperglycemia: high blood sugar causes the body to revert to fat and protein metabolism for energy (glucose cannot be absorbed and is excreted in urine) Radiation in Biotechnology: Cancer Treatment (Radiotherapy): Benefits: Radiation helps treat cancer by killing cancer cells. Risks: It can also harm healthy cells, causing side effects. Genetic Mutation in Plants and Animals: Benefits: Radiation can create beneficial traits in plants and animals, like pest resistance. Risks: Mutations may cause unintended changes, which can be harmful. Chernobyl: Benefits: Studying Radiation: Scientists have researched the long-term effects of radiation on humans, animals, and the environment. Bioremediation: Some biotechnologies now focus on using plants and bacteria to clean up radioactive areas. Genetic Research: The mutations caused by radiation have helped scientists better understand how radiation affects DNA. Risks: Consequences: It caused deaths, long-term health problems, and forced people to move from the area. The area remains unsafe today. Costs: It led to huge cleanup costs, health expenses, and long-term environmental damage. Essay Topics: 1. Biology is the study of living organisms, elaborate on how important biological molecules are in sustaining life. MAIN IDEAS: biological molecules (proteins, lipids, carbs, nucleic acids) and how they are the building blocks of life EX) Biological molecules, including proteins, carbohydrates, lipids, and nucleic acids, are essential for sustaining life. Proteins catalyze reactions and perform structural functions, carbohydrates provide energy and structure, lipids form cell membranes and store energy, and nucleic acids store and transmit genetic information. These molecules enable processes like metabolism, energy production, and cell growth, all of which are crucial for life. 2. Using class material as examples, describe three different biological processes that maintain life. MAIN IDEAS: biological processes and explain their roles - homeostasis, cellular respiration, and protein synthesis EX) Three key biological processes that maintain life are homeostasis, cellular respiration, and protein synthesis. Homeostasis regulates the body’s internal environment, such as temperature and blood sugar, with the help of the pancreas and body regulations. Cellular respiration produces energy (ATP) by converting glucose and oxygen, vital for cellular function, through glycolysis, krebs cycle, and the ETC. Protein synthesis ensures that cells produce the necessary proteins, which are essential for structure and function, through transcription and translation steps. These processes are crucial for survival, growth, and adaptation. 3. Discuss the idea in biology that structure influences function. MAIN IDEAS: structures of biological molecules determine their functions (enzyme, cell membrane, hemoglobin) EX) In biology, the structure of molecules and systems determines their function. For example, the shape of enzymes allows them to bind to specific substrates and catalyze reactions, 3d shape to bind to substrates like the lock and key model in relation to the active and allosteric sites. The phospholipid bilayer of the cell membrane determines selective permeability, regulating what enters and exits the cell, mainly with the hydrophobic interior and hydrophilic exterior layers determine what substances can pass into or out of the cell, which is vital for maintaining homeostasis. Hemoglobin’s structure allows it to carry and release oxygen efficiently, the quaternary structure allows it to bind to oxygen in the lungs and release it in tissues where oxygen is needed. The structure-function relationship is fundamental to biology at every level, from molecules to organisms.