Bio (slides) studyguid PDF
Document Details
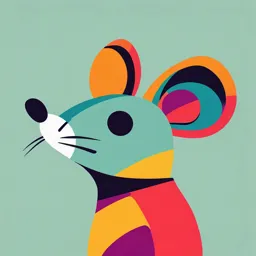
Uploaded by BrightestPalmTree8810
Tags
Summary
This is a study guide on biology. It includes topics on nature of science and life on earth. It contains topics such as cell theory and energy use, with examples, and study tips.
Full Transcript
0.2_Nature of Science Key Concepts: 1. Characteristics of Living Organisms: ○ Cells: All organisms are made up of membrane-bound cells. ○ Replication: All organisms are capable of reproduction. ○ Information: All organisms process hereditary information (genes) and...
0.2_Nature of Science Key Concepts: 1. Characteristics of Living Organisms: ○ Cells: All organisms are made up of membrane-bound cells. ○ Replication: All organisms are capable of reproduction. ○ Information: All organisms process hereditary information (genes) and environmental information. ○ Energy: Organisms acquire and use energy to stay alive. ○ Evolution: Populations of organisms evolve over time. Biology & the Nature of Science: 1. Biology: ○ Aims to understand the living world. ○ Includes various specialties and is linked to other sciences. 2. Science: ○ Systematic study of the physical and natural world through observation, logic, and experimentation. ○ Driven by The Scientific Method: Observations lead to questions. Hypotheses are formulated and tested through experimentation. Data is collected, analyzed, and used to form logical conclusions. Scientific Method: 1. Hypothesis: ○ A testable and falsifiable tentative explanation for an observation. 2. Experiments: ○ Carried out systematically to support or refute a hypothesis. ○ In controlled experiments, most variables remain constant while one variable is changed. 3. Data Analysis: ○ Involves inductive and deductive reasoning: Inductive reasoning: From observations to broad generalizations (hypotheses). Deductive reasoning: From hypotheses or theories to specific predictions that can be tested. Types of Scientific Knowledge: 1. Scientific Facts: Observable and measurable phenomena agreed upon by experts. 2. Scientific Theories: ○ Well-supported explanations of natural phenomena, integrating multiple hypotheses. ○ Examples: Cell Theory, Theory of Evolution by Natural Selection, and Chromosome Theory of Inheritance. 3. Scientific Laws: Descriptions of phenomena that consistently hold true but do not explain why. Hypothesis Testing: 1. Steps in Hypothesis Testing: ○ Clearly state the hypothesis and its predictions. ○ Design observational or experimental studies to test predictions. 2. Example: Why do giraffes have long necks? ○ Food Competition Hypothesis: Giraffes evolved long necks to reach high food sources. ○ Sexual Competition Hypothesis: Giraffes evolved long necks because longer-necked males win more fights and father more offspring. This hypothesis has more supporting data. Study Tips: 1. Focus on the key characteristics of life (cells, reproduction, information, energy, evolution). 2. Understand the scientific method, including how hypotheses are formed and tested. 3. Memorize the difference between scientific facts, theories, and laws. 4. Pay attention to how inductive and deductive reasoning works in scientific inquiry. 0.3_Life on Earth Key Concepts: 1. Characteristics of Living Organisms: ○ Made of Cells: All organisms are composed of one or more cells. ○ Reproduction: Living things replicate. ○ Use Matter and Energy: All organisms acquire and use energy to survive. ○ Process Information: Organisms process genetic information (DNA) and environmental signals. ○ Evolution: Populations of organisms evolve over generations. Cell Theory: 1. All organisms are made of cells. 2. Cells are the basic units of life. 3. All cells arise from preexisting cells through the process of cell division. Historical Context: Robert Hooke and Anton van Leeuwenhoek first observed cells. Louis Pasteur debunked spontaneous generation with his experiment, proving that cells come from other cells. Information Processing: 1. Chromosomal Theory of Inheritance: ○ Genetic information is encoded in DNA located in chromosomes. ○ DNA contains genes, which are units of inheritance. 2. DNA Structure: ○ DNA is a double helix made of four nucleotides: A, T, C, and G. ○ The pairing of A with T and C with G allows DNA to be copied accurately. ○ The central dogma of molecular biology: DNA → RNA → Protein. 3. Mutations: ○ Changes in the DNA sequence can lead to variations in proteins, which may affect the traits of an organism and contribute to evolution. Energy Use: 1. Organisms need: ○ Chemical energy (in the form of ATP). ○ Building blocks to synthesize macromolecules like DNA, RNA, and proteins. 2. Photosynthesis: ○ Plants and some bacteria use sunlight to produce sugar, which is used to make ATP. Evolution: 1. Natural Selection: ○ Evolution is driven by natural selection, where heritable traits that improve reproductive success become more common in a population over time. 2. Darwin’s Theory: ○ Descent with modification: Species are related by common ancestry and evolve over time. ○ Natural selection occurs when: 1. Individuals in a population vary in traits. 2. Some traits provide a reproductive advantage. 3. Fitness: ○ The ability of an individual to produce more offspring relative to others. ○ Adaptation: A trait that increases fitness in a particular environment. Example of Natural Selection: Galápagos Finches: ○ During a period of abundant small, soft seeds, finches with smaller, pointed beaks had a survival advantage, leading to an increase in their population. Study Tips: 1. Focus on the main characteristics of life (cells, energy, reproduction, information processing, and evolution). 2. Understand the structure of DNA and how genetic information flows from DNA to proteins. 3. Review the mechanisms of evolution, particularly natural selection, and the factors that drive it (e.g., variation, adaptation, fitness). 4. Familiarize yourself with Pasteur’s experiment and its importance in establishing cell theory. 0.4_Chem of life Key Concepts 1. Chemistry of Life: ○ Life is based on chemistry, shared by all living organisms. ○ Chemistry focuses on atomic structure, essential elements, and molecular interactions. 2. Chemical Evolution: ○ Explanation for the origin of life, starting with simple molecules forming more complex carbon-based molecules, which could replicate. ○ This led to biological evolution, characterized by metabolism, membranes, and life properties. 3. Matter and Energy in Living Systems: ○ Matter: Used for building structures, cell maintenance, growth, and reproduction. ○ Energy: Required for life functions like metabolism and reproduction. 4. Macromolecules: ○ Living systems involve large carbon-based molecules (macromolecules) and take place in water. ○ The four main types of macromolecules are proteins, lipids, carbohydrates, and nucleic acids. Basic Atomic Structure Atoms: The smallest units that form molecules. Consist of protons (+), neutrons (neutral), and electrons (-). Elements: Pure substances made of one type of atom. Essential elements include carbon (C), hydrogen (H), oxygen (O), and nitrogen (N). Chemical Bonds: 1. Covalent Bonds: ○ Strong bonds formed by sharing electrons between atoms. ○ Types include non-polar covalent (equal sharing) and polar covalent (unequal sharing, e.g., water molecules). 2. Ionic Bonds: ○ Formed when electrons are transferred from one atom to another, creating charged ions (cations and anions). 3. Hydrogen Bonds: ○ Weak bonds that occur between a hydrogen atom and an electronegative atom, essential for the structure of water and DNA. Elements Essential for Life: 96% of living organisms are composed of: ○ Hydrogen (H), Carbon (C), Nitrogen (N), and Oxygen (O). Trace elements like boron are also vital, though required in smaller quantities. Macromolecules in Biological Systems: Structure determines function: The arrangement of atoms in biological molecules (proteins, nucleic acids, etc.) dictates their role in biological processes. Study Tips: 1. Focus on understanding how atomic structure (protons, neutrons, and electrons) relates to chemical properties. 2. Review the different types of chemical bonds (covalent, ionic, hydrogen) and their roles in biology. 3. Memorize key elements essential for life and their functions. 4. Understand how macromolecules are formed and their importance to cellular processes. 0.5_Water is life, Aqueous solutions Key Concepts: 1. Water’s Role in Life Cells are 70-75% water: Essential for maintaining structure, metabolic reactions, and overall cell function. Life originated in water: The structure of water supports life by dissolving substances and participating in chemical reactions. Water's Intracellular Fluid: A complex aqueous solution crucial for cell volume and function. 2. Properties of Water Polar Nature: Water molecules have a bent structure and are highly polar, allowing them to form hydrogen bonds. Solvent Properties: Water is a great solvent for polar and charged molecules, facilitating metabolic reactions by dissolving solutes (like ions). Cohesion and Adhesion: ○ Cohesion: Attraction between water molecules through hydrogen bonds, leading to high surface tension. ○ Adhesion: Water’s attraction to other surfaces, helping it move against gravity in plants (capillary action). Density and Ice: Water is denser as a liquid than as a solid, causing ice to float and insulate water bodies. 3. Temperature Regulation High Specific Heat: Water absorbs and retains heat well due to hydrogen bonding, which helps regulate temperatures in living organisms and environments. Heat of Vaporization: Water requires significant energy to vaporize, which is why sweating is effective for cooling. 4. Aqueous Solutions Solutions: Homogeneous mixtures of solutes dissolved in a solvent (water, in aqueous solutions). Dissociation in Water: Substances like acids and bases dissociate into ions in water, influencing biological processes. Chemical Reactions: Water facilitates chemical reactions by acting as a solvent and sometimes a reactant. 5. Acids, Bases, and pH Acids and Bases: ○ Acids release protons (H+), increasing hydronium ions (H3O+). ○ Bases accept protons, decreasing H3O+ concentration. pH Scale: Measures the acidity or basicity of a solution. ○ Acidic: pH < 7. ○ Neutral: pH = 7 (pure water). ○ Basic: pH > 7. Buffers: Solutions that resist changes in pH, essential for maintaining homeostasis in biological systems (e.g., carbonic acid-bicarbonate buffer in blood). 6. Water's Importance in Acid-Base Reactions Autoionization of Water: Water can dissociate into H+ and OH-, leading to acid-base balance in living systems. Buffers in Cells: Cells use buffers to control pH and protect against fluctuations caused by metabolic reactions. 7. Cellular Sensitivity to pH pH Changes Impact Function: Small pH changes can significantly affect biological molecules and reactions. Enzymatic Sensitivity: Many enzymes require specific pH levels to function properly, which is regulated through pH gradients across cellular compartments. Important Diagrams and Tables: Hydrogen Bonding in Water: Visualize how water molecules interact through hydrogen bonds, which are crucial for many of water’s unique properties. Specific Heats of Liquids: Highlights water’s high specific heat compared to other substances, explaining its role in temperature regulation. 0.6_ Carbon Key Concepts: 1. Life is Based on Carbon Carbon forms the backbone of all biological macromolecules. Versatile nature: Carbon atoms have four valence electrons, allowing them to form up to four covalent bonds with a variety of elements (H, O, N, P, S), enabling diverse molecular shapes and complex functions. 2. Carbon's Importance in Organic Chemistry Hydrocarbon backbones: These form diverse structures like chains, branches, and rings, giving rise to various functional molecules. Chemical Bonds: Carbon-carbon single bonds are strong and flexible, contributing to the stability and diversity of organic molecules. 3. Functional Groups Functional groups determine the chemical behavior of organic molecules and define their reactivity. Important functional groups: ○ Amino Group (-NH₂): Acts as a base, attracting protons. ○ Carboxyl Group (-COOH): Functions as an acid, releasing protons. ○ Carbonyl Group (C=O): Found in aldehydes and ketones, important for linking molecules. ○ Hydroxyl Group (-OH): Acts as a weak acid. ○ Phosphate Group (-PO₄²⁻): Carries two negative charges, key for energy transfer (e.g., ATP). ○ Sulfhydryl Group (-SH): Forms disulfide bonds, important for protein structure. ○ Methyl Group (-CH₃): Involved in methylation reactions, altering molecular behavior. 4. Representation of Molecules Molecular Formulas: Represent the chemical composition of molecules. 3D Structure: Determines the chemical properties, which in turn affect biological functions. Diagrams: Used to represent molecules like DNA, showing how structure dictates function. 5. Macromolecules Polymers: Large molecules made from repeating units called monomers. ○ Polymerization: The process of linking monomers into polymers, typically via condensation reactions (removal of a water molecule). ○ Hydrolysis: The reverse reaction, where water breaks the bond between monomers, increasing entropy and favoring free monomers. Key Macromolecules: Proteins, nucleic acids, carbohydrates—formed through polymerization. 6. Carbon’s Role in Chemical Evolution Early chemical evolution: Small molecules likely polymerized into larger, complex macromolecules under high concentrations of monomers, contributing to the origin of life. Condensation vs. Hydrolysis: ○ Condensation forms polymers, while hydrolysis breaks them apart. ○ Equilibrium favors monomers unless the system has high concentrations of monomers to drive polymerization. Exam Focus Molecular and structural formulas: Be familiar with how different molecules are represented. Properties and reactivity of functional groups: Understand how different functional groups (like amino, carboxyl, and phosphate) affect the behavior of organic molecules. Polymerization and hydrolysis: Know the processes that build up and break down macromolecules. 0.7_ Carbohydrates Key Concepts: 1. Introduction to Carbohydrates Carbohydrates play critical roles in cell structure, cell identity, and energy storage. Types of carbohydrates: ○ Monosaccharides (one sugar unit) – Example: glucose, ribose. ○ Disaccharides (two sugar units) – Example: lactose, sucrose. ○ Polysaccharides (many sugar units) – Example: starch, glycogen, cellulose. 2. Monosaccharides Monosaccharides are simple sugars that cannot be broken down into smaller units. Functions: ○ Provide chemical energy. ○ Serve as building blocks for larger carbohydrates. Structure: ○ Basic formula: (CH2O)n(CH_2O)_n(CH2O)n, where "n" represents the number of carbon atoms. ○ Types of monosaccharides based on the carbonyl group location: Aldose: Carbonyl group at the end of the molecule. Ketose: Carbonyl group in the middle of the molecule. ○ Vary by the number of carbon atoms: 3 (triose), 5 (pentose), 6 (hexose), etc. Form: Monosaccharides exist in both linear and ring forms, with most forming rings in aqueous solutions. 3. Polysaccharides Polysaccharides are long chains of monosaccharide monomers linked by glycosidic bonds. Glycosidic linkage: Forms between two hydroxyl groups of monosaccharides during a condensation reaction (water is released). It can be broken by hydrolysis. Types of linkages: ○ α-1,4-glycosidic linkage: Found in starch and glycogen. ○ β-1,4-glycosidic linkage: Found in cellulose and chitin. Polysaccharides in plants: ○ Starch: Made of α-glucose monomers, stored in plants, and forms a helical structure. Two types: Amylose: Unbranched. Amylopectin: Branched (with α-1,6 linkages). Polysaccharides in animals: ○ Glycogen: Highly branched α-glucose polymer stored in liver and muscle cells, used for quick energy release. 4. Structural Polysaccharides Cellulose: Structural component of the plant cell wall, composed of β-glucose monomers with β-1,4 linkages, forming linear fibers that provide strength. Chitin: Found in the cell walls of fungi and the exoskeletons of insects and crustaceans. Made of N-acetylglucosamine (NAG) monomers linked by β-1,4-glycosidic bonds. Peptidoglycan: Structural polysaccharide in bacterial cell walls, with alternating monosaccharides linked by β-1,4-glycosidic bonds and short peptide chains connecting adjacent strands. 5. Functions of Carbohydrates Structural Support: ○ Carbohydrates such as cellulose, chitin, and peptidoglycan form fibers or sheets that provide strength and elasticity to cells. ○ These structures are resistant to hydrolysis, making them durable. Energy Storage: ○ Carbohydrates store energy, particularly in the form of starch (in plants) and glycogen (in animals). ○ Enzymes like phosphorylase (for glycogen) and amylase (for starch) break down these polysaccharides into glucose, which is used for energy. ○ Glucose is converted into ATP during cellular respiration to power other biological processes. Cell Identity: ○ Carbohydrates on the surface of cells are involved in cell recognition and signaling. ○ Glycoproteins (proteins with carbohydrate groups) and glycolipids (lipids with carbohydrate groups) play a key role in identifying cells as "self" and facilitating communication between cells. 6. Carbohydrates and Energy Storage Photosynthesis: Plants store energy from sunlight in the bonds of carbohydrates during photosynthesis. ○ Equation: Energy content: ○ Carbohydrates contain more energy than carbon dioxide because the electrons in carbon-hydrogen (C-H) and carbon-carbon (C-C) bonds have higher potential energy than those in carbon-oxygen (C=O) bonds. 0.7_ Carbohydrates Introduction to carbohydrates Carbohydrates are organic molecules consisting of carbon, hydrogen, and oxygen; serve as a primary energy source and provide structural support in living organisms. Carbohydrates can be classified as simple sugars (monosaccharides), double sugars (disaccharides), or complex carbohydrates (polysaccharides). Examples of carbohydrates include glucose, fructose, sucrose, cellulose, and starch. Carbohydrates are essential for energy production through cellular respiration. Fiber, a type of carbohydrate, aids in digestion and helps maintain a healthy gut microbiome. Structure and function of monosaccharides Monosaccharides are the simplest form of carbohydrates, with a basic chemical formula of (CH2O)n. They serve as energy sources and structural components in living organisms. Glucose is a common monosaccharide found in many foods and fuels cellular processes. Fructose is another important monosaccharide that is found in fruits and is sweeter-tasting than glucose. Monosaccharides can be classified based on the number of carbon atoms they contain, such as trioses, tetroses, pentoses, and hexoses. They are the building blocks of more complex carbohydrates like disaccharides and polysaccharides. Structure and function of polysaccharides Polysaccharides are complex carbohydrates made of long chains of monosaccharides, providing energy storage, structural support, and cell recognition in organisms. Cellulose is a common polysaccharide found in plant cell walls, providing rigidity and support. Glycogen is a polysaccharide in animals used for energy storage in liver and muscle cells. Chitin is a polysaccharide found in the exoskeletons of arthropods and fungal cell walls, providing structural support. Polysaccharides like hyaluronic acid function in lubricating joints and providing support in connective tissues. Key Terms Aldose Aldose is a type of monosaccharide sugar that consists of a carbon chain with a carbonyl group at one end and hydroxyl groups attached to the remaining carbons. Aldoses serve as building blocks for more complex carbohydrates. Examples of aldoses include glucose, galactose, and ribose. Aldoses can undergo reactions like oxidation and reduction. Linear aldoses can form cyclic structures in aqueous solutions. Cellulose Cellulose is a complex carbohydrate found in the cell walls of plants, providing structural support and rigidity. Cellulose is made up of long chains of glucose molecules linked together. It is the most abundant organic compound on Earth. Cellulose cannot be broken down by most animals, but is digestible by certain bacteria and fungi. Cellulose is often used as a dietary fiber in human nutrition. Chemical energy Chemical energy refers to the potential energy stored in chemical bonds that can be released during a chemical reaction. Chemical energy is a form of potential energy that can be converted into other forms of energy. It is the energy that is released or absorbed during a chemical reaction. Plants convert solar energy into chemical energy through the process of photosynthesis. Chemical energy is essential for the functioning of living organisms as it provides the energy needed for all metabolic activities. Chitin Chitin is a tough, flexible polysaccharide found in the exoskeletons of arthropods and the cell walls of some fungi. It provides structural support and protection in organisms such as insects, crustaceans, and spiders. Chitin is composed of chains of glucose molecules with nitrogen-containing functional groups. It is insoluble in water but can be broken down by enzymes called chitinases. Some medical applications of chitin include wound dressings, drug delivery systems, and tissue engineering scaffolds. Condensation reaction Condensation reaction is a chemical reaction that occurs when two molecules combine to form one larger molecule, producing a molecule of water as a byproduct. Also known as dehydration synthesis. Requires the input of energy. Occurs in biological processes such as protein synthesis. Important for the formation of complex molecules like carbohydrates. Glycogen Glycogen is a polysaccharide consisting of glucose molecules and is the main carbohydrate storage molecule in animals. Glycogen is found in the liver and muscles and serves as a readily accessible energy source. It is synthesized and stored when blood glucose levels are high and broken down to release glucose when needed. Glycogen is more branched than starch, allowing for easy and rapid breakdown. Glycogen storage diseases are inherited conditions that affect the metabolism and storage of glycogen. Glycolipid Glycolipids are molecules composed of a lipid and a carbohydrate group, and are found on the surface of cell membranes. Glycolipids play important roles in cell recognition and communication. They help to stabilize cell membranes. Glycolipids are involved in immune response and cell signaling. Mutations in genes involved in glycolipid synthesis can lead to various diseases. Glycoprotein Glycoproteins are proteins that have sugar molecules attached to them, and they play important roles in cell signaling, immune response, and cell-cell recognition. Glycoproteins are found on the surface of cells and act as receptors for hormones and other signaling molecules. The sugar molecules on glycoproteins can modify the protein's structure and function. Glycoproteins are involved in the immune response by helping to identify foreign invaders such as bacteria or viruses. In certain diseases, such as cancer, abnormal glycoprotein production can occur, leading to changes in cell behavior. Glycosidic linkage Glycosidic linkage is a covalent bond formed between a sugar molecule and another molecule via the hydroxyl group, resulting in the formation of various compounds. Glycosidic linkages are important in carbohydrate metabolism and synthesis. Different types of glycosidic linkages determine the structure and function of carbohydrates. Glycosidic linkages can be broken by hydrolysis, which is important in digestion and energy release. Glycosidic linkages can occur between two sugar molecules or between a sugar and a non-sugar molecule. Hexose Hexoses are simple sugars with six carbon atoms, commonly found in nature and vital for energy production in living organisms. Common hexoses include glucose, fructose, and galactose. They play a crucial role in various metabolic pathways such as glycolysis and the pentose phosphate pathway. Hexoses are a primary energy source for cells, providing fuel for cellular processes. These sugars are often consumed in the diet through sources like fruits, vegetables, and honey. Hydrolysis Hydrolysis is a chemical process where a molecule is split into two parts by the addition of a water molecule. It's a vital process in digestion as it breaks down complex molecules into simpler ones. Hydrolysis often aids in energy production, as with ATP hydrolysis. Enzymes, like proteases and nucleases, catalyze hydrolysis in living organisms. Hydrolysis is instrumental in many biosynthetic pathways and metabolic processes. Ketose Ketose is a type of monosaccharide with a carbonyl group at the second carbon atom, contributing to its unique structure and function. Ketoses are commonly found in fruits and honey. They are important energy sources for living organisms. Examples include fructose and ribose. Ketose molecules form ring structures in aqueous solutions. Monosaccharide A 'Monosaccharide' is the simplest form of carbohydrate consisting of a single sugar molecule, fundamental to cellular energy. Examples include glucose, fructose, and galactose. They can be classified by the number of carbon atoms they contain: triose, pentoses, hexoses etc. Monosaccharides are soluble in water and taste sweet. They serve as building blocks for complex carbohydrates like di- and polysaccharides. Oligosaccharide Oligosaccharides are short carbohydrate chains consisting of 3 to 10 monosaccharide units linked together by glycosidic bonds. They are commonly found in foods such as legumes, beans, and whole grains. Oligosaccharides play a role in cell recognition and communication. This type of carbohydrate is partially digestible by humans and can cause gas due to fermentation in the gut. Some oligosaccharides, like inulin, are considered prebiotics and support the growth of beneficial gut bacteria. Pentose Pentose is a type of sugar that consists of five carbon atoms and is an important component of nucleic acids. Pentose sugars are important for the structure and function of nucleic acids like DNA and RNA. They are also found in other important biomolecules like coenzymes, ATP, and NADP+. Pentoses can be classified into two types: ribose and deoxyribose, depending on the presence or absence of an oxygen atom on the second carbon. Pentoses play a crucial role in energy metabolism and genetic information transfer within cells. Peptidoglycan Peptidoglycan is a complex molecule found in the cell wall of bacteria, providing rigidity and protection to the cell. Peptidoglycan consists of long chains of sugar molecules connected by peptide crosslinks. It plays a crucial role in determining the shape of bacterial cells. Peptidoglycan is the target for many antibiotics, as disrupting its synthesis can weaken or kill bacteria. Some bacteria have a thicker peptidoglycan layer, making them more resistant to antibiotics and immune system attacks. Polysaccharide A Polysaccharide is a complex carbohydrate made up of multiple monosaccharides, or sugar molecules, linked together to form a chain or a branching structure. This structure is responsible for their diverse functions, such as energy storage and structural support. Examples include starch, glycogen, and cellulose, amongst others. Polysaccharides can be broken down into simpler sugars by the process of hydrolysis. The structure and function of polysaccharides significantly depend on the type of monosaccharides they are composed of. Starch Starch is a complex carbohydrate found in plant cells that serves as a main source of energy for both humans and animals. Starch is made up of long chains of glucose molecules. It is stored in plants as granules and can be found in fruits, vegetables, and grains. In humans, starch is broken down into glucose during digestion to provide energy. Starch can be detected with the iodine test, which produces a blue-black color when starch is present. Triose Triose refers to a type of sugar molecule composed of three carbon atoms, commonly found in glycolysis and photosynthesis processes. It plays a crucial role in energy production within cells. Triose phosphates are important intermediates in metabolic pathways. Glyceraldehyde-3-phosphate is a common triose found in glycolysis. Triose sugars can be converted into other molecules to provide building blocks for cell growth. 0.8_ Nucleic Acid Chemical composition of nucleotides Nucleotides consist of a phosphate group, a pentose sugar, and a nitrogenous base, forming the building blocks of nucleic acids. Nitrogenous bases can be purines (adenine, guanine) or pyrimidines (cytosine, thymine, uracil). Phosphate groups link nucleotides together via phosphodiester bonds. DNA structure and function DNA is a double-stranded molecule that carries genetic instructions. It consists of nucleotides, including adenine, thymine, cytosine, and guanine. DNA replicates during cell division to ensure genetic continuity. Genes are segments of DNA that code for specific proteins. The structure of DNA is a double helix, resembling a twisted ladder. Errors in DNA replication can lead to mutations. RNA structure and function RNA is a nucleic acid molecule that plays a key role in protein synthesis, containing a single strand of nucleotides with a ribose sugar and uracil base. RNA can be single-stranded or form complex structures like tRNA or rRNA. RNA can undergo modifications like methylation, affecting its function and stability. The sequence of nucleotides in RNA determines the specific proteins it helps create. RNA functions in processes like transcription, translation, and gene regulation. RNA world hypothesis The 'RNA world hypothesis' suggests that earlier life forms may have relied on RNA both to carry genetic information and to catalyze chemical reactions. RNA is capable of both information storage and catalysis. Predates the evolution of DNA and proteins. Supported by the ribozyme discovery. This theory has insights into the origin of life. Structure and function of nucleic acids Nucleic acids serve as the genetic material of a cell, with DNA encoding genetic information and RNA responsible for protein synthesis. DNA forms a double helix structure, consisting of nucleotide bases adenine, thymine, cytosine, and guanine. RNA is a single strand that contains nucleotide bases adenine, uracil, cytosine, and guanine. Nucleic acids are essential for passing hereditary information from one generation to the next. Mutations in nucleic acids can lead to genetic disorders. Key Terms Activated nucleotides Activated nucleotides are nucleotides that have undergone a chemical reaction to make them reactive and ready to participate in processes like DNA replication and protein synthesis. Adenosine triphosphate (ATP) is a common example of an activated nucleotide used to provide energy for cellular processes. They are used as substrates in enzymatic reactions, serving as the building blocks for nucleic acids. The activation typically involves the addition of phosphates to the nucleotide molecule, increasing its energy content. During transcription and translation, activated nucleotides are crucial for the accurate and efficient synthesis of RNA and proteins. Antiparallel In genetics, antiparallel refers to the opposite orientation of the two strands in a DNA molecule, meaning one strand runs in the 5' to 3' direction while the other runs in the 3' to 5' direction. Antiparallel DNA strands are important for DNA replication and protein synthesis processes. This arrangement allows the DNA strands to act as templates during replication and transcription. The antiparallel structure ensures that DNA molecules can bind together through complementary base pairing. Antiparallel strands are stabilized by hydrogen bonds between the nitrogenous bases in DNA. Complementary Base Pairing Complementary base pairing is a fundamental principle in DNA where adenine pairs with thymine and cytosine pairs with guanine. Complementary base pairing allows DNA replication and transcription to occur accurately. Non-complementary base pairing can lead to errors in DNA replication and mutations. Complementary base pairing is also found in RNA, where adenine pairs with uracil instead of thymine. The stability of the DNA double helix is largely due to the complementary base pairing between the two strands. deoxyribonucleic acid (DNA) DNA is a molecule that contains genetic instructions for the development, functioning, growth, and reproduction of all living organisms. DNA is shaped like a double helix structure, formed by two complementary strands of nucleotides. Nucleotides in DNA consist of a sugar (deoxyribose), a phosphate group, and one of four nitrogenous bases (adenine, thymine, cytosine, guanine). DNA replication ensures genetic information is accurately passed on from one cell generation to the next. Mutations in DNA can result in genetic variation, impacting traits and susceptibility to diseases. Hydrogen bonds Hydrogen bonds are weak attractions between a hydrogen atom in a polar molecule and an electronegative atom in another polar molecule. Hydrogen bonds are important in maintaining the structure of DNA. They are responsible for the unique properties of water, such as its high boiling point and surface tension. Hydrogen bonds are also involved in protein folding and determining the shape of enzymes. They play a crucial role in the stability and function of many biological molecules. Nucleic acid Nucleic acids are complex organic substances in living cells, mainly DNA and RNA, that play critical roles in various biological processes. DNA (deoxyribonucleic acid) stores and transmits genetic information. RNA (ribonucleic acid) synthesizes proteins based on the instructions from DNA. Nucleic acids are comprised of smaller units called nucleotides. Two types of nucleic acids are DNA and RNA; their differences lie in their structure and function. Nucleotide A 'Nucleotide' is a fundamental unit of genetic information. Composed of a sugar, phosphate group, and a nitrogenous base, they form the backbone of DNA and RNA. Nucleotides are formed through a process called 'nucleoside phosphorylation'. Four types exist: Adenine, Guanine, Cytosine, and Thymine (or Uracil in RNA). They also play a key role in cellular energy storage and transfer. They function in cell signaling and metabolism. Phosphodiester Linkage Phosphodiester linkage is a chemical bond that connects nucleotides in nucleic acids, such as DNA and RNA, forming the sugar-phosphate backbone. Occurs between the phosphate group of one nucleotide and the hydroxyl group on the sugar of another nucleotide. Essential for the stability and structure of DNA and RNA. Creates a repeating pattern of sugar-phosphate-sugar in the nucleic acid backbone. The backbone of nucleic acids is negatively charged due to the presence of phosphodiester linkages. Ribonucleic acid (RNA) Ribonucleic acid (RNA) is a nucleic acid molecule essential in protein synthesis, serving as a messenger between DNA and ribosomes. RNA consists of a single strand of nucleotides. There are three types of RNA: messenger RNA (mRNA), transfer RNA (tRNA), and ribosomal RNA (rRNA). RNA is involved in transcription and translation processes. RNA is more versatile than DNA as it can perform various functions beyond genetic information storage. ribozymes Ribozymes are RNA molecules that can catalyze specific chemical reactions, similar to the way enzymes do. Ribozymes are found naturally in cells and can perform various functions, such as self-replication and RNA splicing. They are particularly important in the synthesis and processing of RNA molecules. Ribozymes can be artificially engineered to perform specific reactions, making them potential tools in biotechnology and gene therapy. Studying ribozymes can provide insights into both the origins of life and the development of new therapeutic strategies. 0.9_ Protein Amino acids and their polymerization Amino acids are the building blocks of proteins, polymerizing through peptide bonds to form polypeptide chains essential for various biological functions. Twenty different amino acids exist in proteins, each with unique side chains and properties. Amino acids bond through dehydration synthesis, forming peptide bonds between the carboxyl group of one amino acid and the amino group of another. The sequence of amino acids in a polypeptide chain determines its final structure and function. Protein synthesis involves transcription of DNA into mRNA, translation of mRNA into amino acids, and subsequent folding into functional proteins. Protein function Proteins play crucial roles in the human body, facilitating physiological processes such as structure, communication, transportation, and defense. Enzymes are a type of protein that catalyze biochemical reactions. Proteins help maintain cellular structure and provide scaffolding for tissues. Transport proteins carry molecules across cell membranes. Antibodies are proteins that aid in immune defense by recognizing and neutralizing foreign invaders. Protein structure Protein structure refers to the three-dimensional arrangement of atoms in a protein molecule, which is essential for its function. Proteins are composed of linear chains of amino acids that fold into specific shapes. The primary structure of a protein is its specific sequence of amino acids. The secondary structure refers to local folding patterns, such as alpha helices and beta sheets. The tertiary structure is the overall three-dimensional shape of the protein, determined by interactions between amino acid side chains. Key Terms Denatured Protein Denatured protein refers to the alteration of a protein's structure and loss of function due to external factors such as heat or pH changes. Denaturation can be reversible or irreversible depending on the extent of structural changes. Factors causing denaturation include high temperatures, extreme pH levels, and exposure to certain chemicals. Denatured proteins often lose their normal shape, leading to a loss of function. Protein denaturation can disrupt important biological processes in the body, impacting overall health. Enzymes Enzymes are proteins that act as catalysts in living organisms, expediting chemical reactions without being consumed by these processes. Enzymes work by lowering the activation energy of a reaction. Each enzyme catalyses a specific reaction, often associated with its name. Enzymes can be influenced by factors such as temperature and pH. Enzyme activity can be inhibited, either completely (non-competitive) or temporarily (competitive). Molecular chaperones Molecular chaperones are proteins that assist in proper folding of other proteins, preventing misfolding and aggregation, and aiding in protein transport. They play a crucial role in maintaining cellular homeostasis. Chaperones can also help with the refolding of denatured proteins. Some chaperones are involved in targeting misfolded proteins for degradation. Molecular chaperones are essential for the proper functioning of a cell by ensuring protein quality control. Peptide bond A peptide bond is a chemical linkage formed between two amino acids, connecting the amino group of one with the carboxylic acid group of another. Peptide bonds are responsible for linking amino acids to form proteins. They are created through a dehydration synthesis reaction. Breakdown of peptide bonds occurs during protein digestion. The formation and breaking of peptide bonds are catalyzed by enzymes. Polypeptide A polypeptide is a linear chain of amino acids linked by peptide bonds. It's the fundamental component of proteins. Polypeptides are synthesized from mRNA in a process called translation. The function of a polypeptide depends on its structure and the sequence of its amino acids. Polypeptides can exist as single units or may associate to form larger structures. Enzymes, hormones, and antibodies are examples of proteins, which are composed of polypeptides. Primary structure The 'Primary structure' refers to the specific sequence of amino acids in a protein or polypeptide chain. It's determined by the gene encoding the protein. Each amino acid in the sequence is linked by a peptide bond. Changes in the primary structure can influence a protein's functionality. This structure is critical for understanding protein function and properties. Prions Prions are misfolded proteins that can cause normal proteins to become misfolded as well, leading to various neurodegenerative diseases. Prions are unique because they lack genetic material and can self-replicate. The misfolded proteins aggregate and form plaques in the brain, causing damage to nerve cells. Common prion diseases include Creutzfeldt-Jakob disease, mad cow disease, and fatal familial insomnia. Prions are extremely resistant to common sterilization methods and can survive in the environment for years. Quaternary structure Quaternary structure refers to the complex 3D arrangement involving multiple, separate polypeptide chains (subunits) that interact to form a larger protein molecule. It is the highest level of protein structural organization. Hemoglobin and DNA polymerase are examples of proteins with quaternary structure. This structure is stabilized by non-covalent interactions. A disruption in quaternary structure can affect protein function. Secondary structure Secondary structure refers to the localized, repeatable, three-dimensional shape of proteins and nucleic acids, namely alpha helices and beta sheets in proteins. In proteins, it's stabilized by hydrogen bonds between the backbone amide and carboxyl groups. Two types are present in proteins: alpha helix and beta pleated sheet. In nucleic acids, it describes the hydrogen bonding pattern between base pairs. Ribonucleic acid (RNA) often forms hairpin structures as part of its secondary structure. Tertiary structure The 'Tertiary structure' refers to the three-dimensional shape of a protein, resulting from interactions between amino acid side chains within the molecule. Often stabilized by bonds like disulfide bridges and hydrophobic interactions. Determines the functionality and specificity of proteins. Perturbations can lead to protein malfunction and disease states. Chaperone proteins assist in folding to the correct tertiary structure. 1. Introduction to Energy and Enzymes Energy is essential for cellular activities, with enzymes playing a crucial role in regulating and directing these processes. Metabolic pathways are ordered series of reactions that break down or build molecules. 2. Energy Types and Chemical Reactions Kinetic energy: Energy of motion (e.g., thermal energy from molecule movement). Potential energy: Stored energy in bonds. Chemical reactions involve the transformation of potential energy to kinetic energy, especially through bond changes. 3. Thermodynamics and Energy in Reactions First Law of Thermodynamics: Energy cannot be created or destroyed, only transferred or transformed. Enthalpy (H): Total energy in a molecule, including bond energy and surrounding pressure/volume effects. Exothermic reactions release heat (ΔH\Delta HΔH is negative), while endothermic reactions absorb heat (ΔH\Delta HΔH is positive). Entropy (S): A measure of disorder, increases in less ordered systems (2nd Law of Thermodynamics). 4. Gibbs Free Energy Gibbs free energy (G): Determines whether a reaction is spontaneous. Spontaneous reactions: ΔG 0ΔG>0 (endergonic). 5. Factors Affecting Reaction Rates Temperature and concentration influence reaction rates. Higher temperatures or concentrations lead to more collisions and faster reactions. 6. Coupling Reactions Energetic coupling links exergonic reactions (energy-releasing) with endergonic reactions (energy-requiring), driven by electron or phosphate transfer. 7. Redox Reactions Oxidation: Loss of electrons. Reduction: Gain of electrons. These reactions are always paired, representing a key mechanism of energy transfer. 8. ATP and Phosphate Group Transfer ATP stores high potential energy due to its three phosphate groups. ATP hydrolysis releases energy (7.3 kcal/mol) by breaking a phosphate bond, driving many cellular processes. Phosphorylation: ATP adds phosphate to target molecules, raising their potential energy. 9. Enzymes and Catalysis Enzymes lower the activation energy needed for reactions to proceed by bringing substrates together and stabilizing transition states. The active site of enzymes is where substrates bind, leading to precise reactions. 10. Enzyme Kinetics and Regulation Saturation kinetics: At high substrate concentrations, the rate of enzyme activity plateaus as all active sites are occupied. Enzyme regulation: Enzymes can be regulated by molecules that change their shape or activity, either by competitive inhibition or allosteric regulation. 11. Environmental Effects on Enzymes Temperature and pH affect enzyme shape and function. Each enzyme is optimized for specific conditions. 12. Metabolic Pathways and Feedback Inhibition Metabolic pathways consist of sequential enzyme-catalyzed reactions. Feedback inhibition: Pathways are regulated by the end product, which inhibits an earlier enzyme to prevent overproduction. 1. Introduction to Lipids Lipids are hydrophobic molecules, which means they do not dissolve in water. The primary role of lipids is to form cell membranes, provide energy storage, and act as signaling molecules. Three main types of lipids found in cells: ○ Fats (triglycerides): Made of glycerol and three fatty acids. ○ Steroids: Have a four-ring structure (e.g., cholesterol). ○ Phospholipids: Contain a glycerol, a phosphate group, and two fatty acids. Phospholipids are amphipathic, having both hydrophilic and hydrophobic parts. 2. Structure and Function of Fats Fats are also known as triacylglycerols or triglycerides. ○ Formed by condensation reactions between glycerol and three fatty acids. ○ Fatty acids can be saturated (no double bonds, solid at room temperature) or unsaturated (one or more double bonds, liquid at room temperature). The primary function of fats is energy storage. 3. Steroids and Cholesterol Steroids have a characteristic four-ring structure. Cholesterol is an essential steroid in animal cell membranes, contributing to membrane fluidity. Cholesterol is also a precursor for steroid hormones (e.g., estrogen, testosterone). 4. Phospholipids and Cell Membranes Phospholipids are the major component of cell membranes. ○ They are amphipathic, with a hydrophilic head (phosphate group) and hydrophobic tails (fatty acids). ○ This dual nature allows phospholipids to form bilayers in aqueous environments, creating a barrier that separates the cell interior from the exterior. 5. Membrane Structure and Fluidity The fluid mosaic model describes the structure of cell membranes: ○ Membranes are a fluid bilayer of phospholipids with proteins embedded within it. ○ Membrane fluidity is influenced by: The saturation level of fatty acids (unsaturated fats increase fluidity). The presence of cholesterol (which moderates fluidity). Temperature (higher temperatures increase fluidity). 6. Selective Permeability of Membranes Cell membranes are selectively permeable, allowing some substances to pass through while blocking others. ○ Small, nonpolar molecules (e.g., oxygen, carbon dioxide) can cross easily. ○ Ions and large polar molecules (e.g., glucose) require specific transport mechanisms. 7. Transport Across Membranes Passive Transport: Movement of molecules without energy input, driven by concentration gradients. ○ Diffusion: Movement from high to low concentration. ○ Facilitated diffusion: Uses membrane proteins (e.g., channels or carriers) to help move substances across membranes. Active Transport: Requires energy (usually ATP) to move molecules against their concentration gradient. 8. Types of Membrane Proteins Integral (transmembrane) proteins: Span the entire membrane and are involved in transport or signaling. Peripheral proteins: Attach to one side of the membrane and play roles in signaling or maintaining cell shape. 9. Membrane Dynamics and Transport Mechanisms Osmosis: The diffusion of water across a selectively permeable membrane. ○ Water moves from areas of low solute concentration to areas of high solute concentration. Tonicity: ○ Hypotonic solution: Lower solute concentration outside the cell, leading to water entering the cell (cell may burst). ○ Hypertonic solution: Higher solute concentration outside the cell, leading to water leaving the cell (cell shrinks). ○ Isotonic solution: Equal solute concentrations inside and outside the cell (no net water movement). 10. Bulk Transport Cells use bulk transport mechanisms for large molecules or particles: ○ Exocytosis: Vesicles fuse with the membrane to release substances outside the cell. ○ Endocytosis: The cell membrane engulfs substances to bring them into the cell. Phagocytosis: "Cell eating," bringing large particles into the cell. Pinocytosis: "Cell drinking," bringing fluids and dissolved solutes into the cell. Receptor-mediated endocytosis: Specific molecules are taken in after binding to receptors on the cell surface. 1. Overview of Cell Types Cell Theory: All organisms are made of cells. Basic Cell Components: ○ Proteins: Facilitate cellular functions. ○ Nucleic Acids: Store and process genetic information. ○ Carbohydrates: Provide energy and structural support. ○ Plasma Membrane: Controls the passage of substances in and out of the cell. Cell Classification: ○ Prokaryotic Cells: Lack a nucleus and membrane-bound organelles; DNA is in a nucleoid. ○ Eukaryotic Cells: Contain a nucleus and membrane-bound organelles. 2. Prokaryotic Cells DNA is organized into a circular chromosome and additional small DNA circles (plasmids). Cell Wall: Provides structure and protection (mainly composed of peptidoglycan in bacteria). Flagella and Fimbriae: Used for movement and attachment. Cytoskeleton: Thin protein filaments maintain cell shape and division. 3. Eukaryotic Cell Structures Nucleus: ○ Contains the cell’s DNA. ○ Nucleolus: Synthesizes ribosomal RNA and assembles ribosome subunits. Ribosomes: ○ Synthesize proteins. ○ Found in the cytosol (free) or attached to the rough endoplasmic reticulum (ER). Endomembrane System: ○ Rough ER: Studded with ribosomes, it synthesizes proteins for secretion or membrane insertion. ○ Smooth ER: Synthesizes lipids and detoxifies harmful substances. ○ Golgi Apparatus: Modifies, sorts, and ships proteins and lipids received from the ER. ○ Lysosomes: Found only in animal cells; contain enzymes for breaking down waste. 4. Other Organelles in Eukaryotic Cells Mitochondria: ○ Powerhouse of the cell, produces ATP. ○ Contains its own DNA and can grow and divide independently. Chloroplasts (plant cells only): ○ Site of photosynthesis. ○ Contains stacks of thylakoids (grana) within the stroma where energy conversion occurs. Vacuoles (mainly in plant cells): ○ Store water, ions, nutrients, and waste. ○ In seeds, they contain proteins; in flowers, pigments. Peroxisomes: ○ Centers for redox reactions, including the breakdown of toxic substances like hydrogen peroxide. 5. Cell Wall and Extracellular Matrix (ECM) Cell Wall (plants, fungi, algae): Provides structural support and protection. Extracellular Matrix (animals): Network of proteins and carbohydrates for support and signaling. 6. Cytoskeleton Structure and Movement: ○ Composed of actin filaments, intermediate filaments, and microtubules. ○ Provides cell shape, supports organelle movement, and aids in cell division. Microtubules: Act as tracks for intracellular transport and form structures like cilia and flagella. Biology: Cell Structure I Nucleus Medical Media 1. Overview of the Cytoskeleton The cytoskeleton is a network of protein fibers that gives shape, structural stability, and organization to cells. It plays a crucial role in cell movement, division, and transport of materials within the cell. 2. Major Types of Cytoskeletal Elements 1. Actin Filaments (Microfilaments) ○ Structure: The smallest cytoskeletal elements are made of actin subunits. ○ Functions: Involved in cell movement, with myosin motor proteins facilitating actions like cytokinesis (cell division) and cytoplasmic streaming (flow of cytoplasm). Enables cell crawling by growing and causing membrane bulges, pushing the cell forward(10_Energy, Metabolism …). 2. Intermediate Filaments ○ Structure: Composed of various proteins, including keratins and nuclear lamins. ○ Functions: Provide structural support by resisting tension. Stabilize the nucleus by forming the nuclear lamina, a mesh-like layer that maintains nuclear shape and anchors chromosomes. Common in structures like hair, nails, and the nuclear envelope(10_Energy, Metabolism …). 3. Microtubules ○ Structure: The largest cytoskeletal elements, composed of tubulin dimers (α-tubulin and β-tubulin). ○ Functions: Act as "tracks" for intracellular transport, especially for vesicles and organelles. Essential for chromosome separation during cell division. Form structures like cilia and flagella, enabling cell movement(10_Energy, Metabolism …)(10_Energy, Metabolism …). 3. Functions of the Cytoskeleton Support and Shape: Maintains the cell’s shape and provides support. Intracellular Transport: Motor proteins like kinesin use ATP to "walk" along microtubules, transporting vesicles to various parts of the cell. Cell Division: Microtubules organize chromosomes during mitosis and meiosis, ensuring accurate cell division(10_Energy, Metabolism …)(10_Energy, Metabolism …). 4. Cell Movement and Cytoskeleton Interaction Actin-Myosin Interactions: Actin filaments interact with myosin motor proteins to produce movement, such as in muscle contraction and cell crawling. Cilia and Flagella: Microtubules form the core of cilia and flagella, which propel cells by a whip-like motion in eukaryotic cells (e.g., sperm) or by rotation in prokaryotic cells(10_Energy, Metabolism …)(10_Energy, Metabolism …). 메디컬 애니메이션 | 세포 운동에서 Actin 작용 | The Role of Actin in Cell Motility | Medical… 1. Introduction to the Cell Cycle Cell Division: New cells form by splitting pre-existing cells. Mitosis vs. Meiosis: ○ Mitosis: Produces identical somatic (body) cells. ○ Meiosis: Produces genetically diverse gametes (reproductive cells). Cytokinesis: Accompanies both processes, dividing the cytoplasm to form two daughter cells. 2. Key Concepts of Cellular Replication Basic Steps: 1. Copying DNA. 2. Separating copies. 3. Dividing cytoplasm to complete the cell division. 3. Chromosome Structure Chromosome: A single DNA molecule wrapped around proteins (histones). Gene: DNA region coding for specific RNA/protein. Replication: ○ Chromosomes replicate and condense before mitosis. ○ Sister chromatids: Identical copies attached at the centromere. 4. Phases of the Cell Cycle Interphase (non-dividing phase): ○ G1 Phase: Growth and normal function. ○ S Phase: DNA replication. ○ G2 Phase: Preparation for division. M Phase (mitotic phase): Includes mitosis and cytokinesis. 5. Mitosis: Stages 1. Prophase: ○ Chromosomes condense. ○ Spindle apparatus forms to guide chromosome movement. 2. Prometaphase: ○ Nuclear envelope breaks down. ○ Microtubules attach to chromosomes at kinetochores. 3. Metaphase: ○ Chromosomes align at the metaphase plate. 4. Anaphase: ○ Sister chromatids separate, moving to opposite poles. 5. Telophase: ○ New nuclear envelopes form around each chromosome set. ○ Chromosomes begin to decondense. ○ Cytokinesis: Completes cell division, forming two daughter cells. 6. Control of the Cell Cycle Checkpoints: Regulatory points to ensure proper cell cycle progression. Cancer: Results from uncontrolled cell division due to failed checkpoints. ○ Cancerous cells ignore normal regulatory signals and may invade other tissues. Chapter 13: Meiosis Introduction to Meiosis Meiosis reduces chromosome number by half: ○ Gametes (sperm and egg) contain half the chromosome number of the parent cell. ○ Fertilization restores the full chromosome number. Chromosomes and Genetic Terms Chromosomes: Structures of DNA and proteins carrying hereditary information. Homologous Chromosomes (Homologs): Same genes in the same location but may carry different alleles. Diploid (2n): Two sets of chromosomes (e.g., humans = 46). Haploid (n): One set of chromosomes (e.g., humans = 23 in gametes). Alleles: Different versions of the same gene. Phases of Meiosis I 1. Prophase I: ○ Chromosomes condense, and homologs pair through synapsis to form bivalents. ○ Crossing over occurs at chiasmata, exchanging genetic material. 2. Metaphase I: ○ Homologous pairs align randomly at the metaphase plate (independent assortment). 3. Anaphase I: ○ Homologs separate to opposite poles. 4. Telophase I and Cytokinesis: ○ Cells divide, resulting in two haploid cells with replicated chromosomes. Phases of Meiosis II 1. Prophase II: ○ Spindle apparatus forms; chromosomes prepare for division. 2. Metaphase II: ○ Chromosomes align at the metaphase plate. 3. Anaphase II: ○ Sister chromatids separate and move to opposite poles. 4. Telophase II and Cytokinesis: ○ Four genetically distinct haploid cells form. Key Features of Meiosis Crossing Over: Creates new allele combinations on chromosomes. Independent Assortment: Random alignment of homologs during Metaphase I creates genetic variation. Fertilization: Combines unique gametes to produce a genetically unique zygote. Comparison: Mitosis vs. Meiosis Feature Mitosis Meiosis Number of Divisions 1 2 Chromosome Same as Halved Number parent Genetic Variation None Present (crossing over, independent assortment). Errors in Meiosis Nondisjunction: Homologs or sister chromatids fail to separate properly. ○ Leads to aneuploidy (e.g., trisomy 21 causes Down syndrome). Advantages of Meiosis 1. Genetic Variation: Increases offspring survival in changing environments. 2. Purifying Selection Hypothesis: Reduces accumulation of harmful mutations. Study Guide: Chapter 14 - Mendel and the Gene Key Terms 1. Gene: Hereditary factor influencing a specific trait. 2. Allele: Variant form of a gene. 3. Genotype: Combination of alleles in an individual. 4. Phenotype: Observable traits influenced by genotype and environment. 5. Homozygous: Two identical alleles for a gene. 6. Heterozygous: Two different alleles for a gene. 7. Dominant Allele: Phenotype expressed in heterozygous or homozygous states. 8. Recessive Allele: Phenotype expressed only in homozygous state. Mendel’s Experiments 1. Model Organism: Garden peas, chosen for short generation time, polymorphic traits, and ability to control mating. 2. Traits Studied: Seed shape, seed color, pod shape, pod color, flower color, flower/pod position, stem length. 3. Key Results: ○ Monohybrid Cross: Crossing pure lines led to F1 showing only the dominant phenotype, and F2 showing a 3:1 ratio of dominant to recessive. ○ Particulate Inheritance Hypothesis: Traits are determined by discrete particles (genes) that do not blend. Mendel’s Principles 1. Principle of Segregation: ○ Alleles separate during gamete formation, leading to offspring receiving one allele from each parent. ○ Illustrated by 3:1 phenotype ratio in F2 generation of monohybrid crosses. 2. Principle of Independent Assortment: ○ Alleles of different genes are transmitted independently during gamete formation. ○ Demonstrated by 9:3:3:1 phenotype ratio in dihybrid crosses. Extensions to Mendel’s Rules 1. Incomplete Dominance: Heterozygotes show an intermediate phenotype (e.g., pink flowers in snapdragons). 2. Codominance: Both alleles in a heterozygote are fully expressed (e.g., AB blood type). 3. Multiple Alleles: More than two alleles exist for a gene in a population (e.g., ABO blood types). 4. Pleiotropy: One gene influences multiple traits (e.g., Marfan syndrome). 5. Gene-Gene Interactions: Phenotype depends on interactions between different genes (e.g., epistasis in ABO blood type). 6. Polygenic Traits: Continuous traits influenced by multiple genes (e.g., human height). Environmental Effects 1. Environmental factors can influence phenotypes, leading to variability even among individuals with the same genotype (e.g., PKU diet preventing symptoms). Pedigree Analysis 1. Autosomal Recessive: ○ Trait appears in homozygous individuals. ○ Often skips generations (e.g., sickle cell disease). 2. Autosomal Dominant: ○ Trait appears in homozygous or heterozygous individuals. ○ Every affected individual has at least one affected parent (e.g., Huntington disease). 3. X-Linked Recessive: ○ Males express the trait if they inherit the recessive allele from their mother. ○ Females express the trait only if homozygous recessive. ○ Often skips generations (e.g., red-green color blindness). 4. X-Linked Dominant: ○ Affected males pass the trait to all daughters, none of their sons. ○ Affected females pass the trait to half their offspring (e.g., vitamin D-resistant rickets). Applications of Mendel’s Rules 1. Chromosome Theory of Inheritance: ○ Genes are located on chromosomes. ○ Behavior of chromosomes during meiosis explains Mendel’s principles. 2. Crossing Over: ○ Linked genes can be recombined through crossing over, generating genetic diversity. 3. Quantitative Traits: ○ Continuous traits (e.g., kernel color in wheat) result from additive effects of multiple genes. Evolution by Natural Selection: Comprehensive Study Notes Introduction to Evolutionary Thought Historical Perspectives on Species Origin Typological Thinking (Pre-Darwin Era) ○ Plato: Organisms as perfect, unchanging "types" created by God ○ Aristotle's "Great Chain of Being" Hierarchical organization of species Humans at the top, just below angels and God Species viewed as fixed and unchanging Early Evolution Theories Jean-Baptiste de Lamarck's Theory (1809) ○ Proposed progressive evolution ○ Organisms move "up" the evolutionary chain ○ Suggested acquired characteristics could be inherited ○ Key Limitation: Incorrectly assumed purposeful progression Darwin and Wallace's Revolutionary Concept Breakthrough Insights ○ Replaced linear progression with population-based thinking ○ Emphasized variation among individuals ○ Evolution occurs through differential reproductive success ○ Scientific approach: Testable mechanism for change Evidence for Evolution Fossil Record Evidence Geological Time Scale ○ Radiometric dating techniques ○ Earth approximately 4.6 billion years old ○ Earliest life signs: 3.4-3.8 billion years ago Types of Evolutionary Evidence 1. Extinction Patterns ○ Over 99% of species extinct ○ Continuous species turnover throughout Earth's history 2. Transitional Features ○ Intermediate traits linking ancestral and descendant species ○ Provide direct evidence of evolutionary change 3. Vestigial Traits ○ Reduced/non-functional structures ○ Examples: Whale hip bones Blind cave fish eye sockets Human tailbone 4. Homology ○ Similarities across species indicating common ancestry ○ Three levels of homology: Genetic Developmental Structural Geographical Distribution Darwin's Galapagos Observations ○ Finch species variations ○ Closely related species in same geographic regions ○ Phylogenetic tree relationships Natural Selection Mechanism Darwin's Four Postulates 1. Variation exists in populations 2. Some trait differences are heritable 3. Differential survival and reproduction 4. Non-random survival of individuals Key Biological Definitions Fitness: Ability to produce surviving, fertile offspring Adaptation: Heritable trait increasing reproductive success Selection: Differential reproduction based on heritable variation Case Studies in Evolution Bacterial Antibiotic Resistance Mycobacterium tuberculosis ○ Mutation in rpoB gene ○ Demonstrates natural selection principles ○ Population-level genetic changes Galapagos Finch Evolution Grant's Research ○ Beak size changes during environmental shifts ○ Drought impact on population genetics ○ Documented evolutionary adaptation Common Misconceptions What Natural Selection Is NOT Not Goal-Directed ○ Mutations are random ○ No purposeful trait development Not Perfectionist ○ No "higher" or "lower" organisms ○ Adaptations are compromises Not Individual-Level Change ○ Populations evolve, not individuals ○ Allele frequency changes over generations Evolutionary Constraints Genetic Constraints ○ Limited by existing variation ○ Pleiotropy effects Historical Constraints ○ New traits evolve from pre-existing structures Environmental Constraints ○ Adaptation depends on current environmental conditions Additional Evolutionary Processes Genetic Drift Gene Flow Mutation Key Takeaway Evolution is a complex, multifaceted process driven by variation, inheritance, and environmental interactions.