Bio 13.1 Part 2 PDF
Document Details
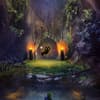
Uploaded by iiScholar
Arizona State University
Tags
Summary
This document details the structure, function and regulation of the human heart. It covers heart chambers, valves, cardiac muscle, and the cardiac conduction system. The document also briefly describes the role of the heart in thermoregulation.
Full Transcript
13.1.06 The Heart The heart is a muscular structure that contains four chambers: two thin-walled **atria** and two thick-walled **ventricles**. The walls of the chambers are composed primarily of cardiac muscle. The left side and right side of the heart each consist of an atrium and a ventricle, an...
13.1.06 The Heart The heart is a muscular structure that contains four chambers: two thin-walled **atria** and two thick-walled **ventricles**. The walls of the chambers are composed primarily of cardiac muscle. The left side and right side of the heart each consist of an atrium and a ventricle, and the two sides function as separate pumps that contract simultaneously to move blood throughout the body. The atria function to transfer blood into the adjoining ventricles; therefore, the atria are not as thick-walled (muscular) as the ventricles, which must propel blood over significantly greater distances. The right ventricle pumps blood to the lungs, which are located near the heart, while the left ventricle pumps blood throughout the rest of the body. The left ventricle is the heart\'s most muscular chamber, an adaptation that allows the left ventricle to contract more forcefully than the other heart chambers, generating sufficiently high pressure to efficiently deliver blood to body regions located relatively far from the heart. Specialized valves provide for unidirectional blood flow through the heart. **Atrioventricular (AV) valves** permit blood to flow from the atria into the ventricles but prevent blood in the ventricles from moving directly back into the atria. **Semilunar valves** allow blood to flow from the ventricles into the blood vessels (ie, arteries) that lead away from the ventricles but stop blood in these arteries from flowing back into the ventricles. Figure 13.11 shows the pathway that blood follows through the heart and the locations of the AV and semilunar valves that prevent backflow. **Figure 13.11** Atrioventricular (AV) and semilunar valves in the heart. A diagram of a heart Description automatically generated Chapter 13: Circulation 463 Cardiac muscle cells making up the walls of the heart are connected end to end by structures called **intercalated discs**, which contain gap junctions and desmosomes. Gap junctions permit ions to pass directly from cell to cell, allowing [action potentials](javascript:void(0)) (ie, series of membrane voltage changes responsible for triggering muscle cell contraction) to spread rapidly across the heart. Desmosomes prevent cardiac muscle cells from pulling away from each other during contraction. The rapid transmission of action potentials occurring throughout the heart muscle via gap junctions and the strong connections that exist between cardiac muscle cells due to the presence of desmosomes allow the heart to act as a coordinated unit. The coordination of activity among cells is the basis for the heart\'s ability to function as an efficient pump. More information about cardiac muscle cell structure and function is found in Concept 17.2.02. Cardiac muscle is **myogenic**, that is, the electrical signal that stimulates contraction of the heart comes from self-depolarizing cells within the heart muscle itself rather than from an outside source, such as the nervous system. The heart also contains specialized cells that form a **cardiac conduction system** (Figure 13.12). This system allows the contraction signal, which consists of a wave of [depolarization](javascript:void(0)), to be rapidly transmitted to particular regions of the heart in a specific sequence. In this way, the timing of contraction of different parts of the heart is controlled to ensure that the heart pumps blood effectively. **Figure 13.12** Pathway followed by electrical signals passing through the cardiac conduction system.  Chapter 13: Circulation 464 Events leading to coordinated heart contraction include the following: 1.The electrical signal stimulating heart contraction typically originates in a region called the **sinoatrial (SA) node** in the right atrium. This signal spreads from the SA node across both atria via gap junctions, triggering simultaneous contraction of the atria, and travels through cells of the conduction system to a region called the **AV node**. 2.The AV node is located in the inferior portion of the wall that separates the two atria (ie, interatrial septum). Cells making up the AV node possess structural adaptations that delay passage of the signal to the ventricles by approximately 0.1 seconds, which, as discussed later in this concept, contributes to the heart\'s coordinated pumping action. 3.After passing through the AV node, the contraction signal is carried through a portion of the conduction system called the **AV bundle** (bundle of His), which is the only structure that electrically links the atria to the ventricles. 4.The AV bundle divides into two **bundle branches**, which are conduction system pathways that carry the signal within the wall separating the ventricles (ie, interventricular septum) toward the heart\'s pointed inferior end (ie, apex). 5.The bundle branches divide to form **Purkinje fibers**, which carry the signal superiorly within the ventricular walls. The signal triggers ventricular contraction, which begins at the heart\'s apex and progresses superiorly toward the atria. Operation of the heart\'s conduction system can be monitored using electrodes attached to the body\'s surface at specific locations. Data collected by these electrodes can be used to generate a graph with characteristic wave patterns. This graph, called an **electrocardiogram (ECG)**, provides a record of [electrical activity](javascript:void(0)) within the heart as the heart beats. Figure 13.13 shows how specific components of an ECG correspond to depolarization or repolarization of specific regions of the heart. Chapter 13: Circulation 465 **Figure 13.13** Electrocardiogram record of the heart\'s electrical activity. The series of events that the heart undergoes to pump blood is called the **cardiac cycle** (see Figure 13.14). These events cause pressure changes within the heart that allow the heart to fill with blood and then eject the blood. The cardiac cycle involves two primary phases: diastole and systole. During **diastole**, cardiac muscle relaxes, which lowers pressure within the heart and allows blood to enter the heart. Conversely, during **systole**, cardiac muscle contracts (first in the atria and then in the ventricles), which increases pressure within the heart and causes blood to be pumped out of the heart into the attached arteries. A diagram of heart anatomy Description automatically generated Chapter 13: Circulation 466 **Figure 13.14** Events occurring during the cardiac cycle. The pattern of activity in the cardiac conduction system underlies the pattern of muscle contraction that characterizes the cardiac cycle and results in efficient pumping of blood. For example, the delay in signal transmission mediated by the AV node allows the atria to finish contracting before the ventricles begin contracting, facilitating blood transfer from atria to ventricles. Likewise, transfer of blood out of the ventricles into arteries is efficient because ventricular contraction begins at the heart\'s apex and proceeds superiorly, thereby squeezing blood toward the heart\'s base (ie, broad superior end), where the arteries are attached.  Chapter 13: Circulation 467 Pressure changes within the heart cause opening and closing of heart valves during the [cardiac cycle](javascript:void(0)) (Figure 13.14). Closing of these valves produces heart sounds (\"lub-dup\") characteristic of a beating heart. The first sound (\"lub\") occurs at the beginning of ventricular systole, when pressure in the contracting ventricles becomes greater than pressure in the relaxed atria, causing the AV valves to forcefully close. The second sound (\"dup\") occurs when the semilunar valves close at the beginning of ventricular diastole, when pressure in the ventricles drops below pressure in the arteries leading from the heart. The purpose of the heart is to propel blood to all parts of the body. The heart\'s effectiveness at this task can be assessed by measuring **cardiac output (CO)**, which is defined as the amount of blood pumped from one ventricle (either left or right) in one minute. CO is affected by both **heart rate (HR)** and **stroke volume (SV)**. HR (ie, pulse) is the number of times the heart completes the cardiac cycle per minute (ie, number of heart beats per minute), and SV is the volume of blood pumped from the ventricle during a single contraction (ie, during ventricular systole). CO can be calculated as follows: CO = HR × SV **Concept Check 13.1** A person\'s cardiac output (CO) is determined to be 5,100 mL/min. Every 0.8 seconds, the person\'s sinoatrial node generates a signal that passes through the cardiac conduction system. Assuming the person\'s heart is functioning as expected, what is this person\'s stroke volume? [**Solution**](javascript:void(0)) 13.1.07 Pattern of Blood Circulation The left and right sides of the heart (each of which is composed of an atrium and a ventricle) function as independent pumps that propel blood through two separate circuits of blood vessels. The **pulmonary circuit** carries blood under lower pressure from the right side of the heart to the sites of gas exchange in the lungs and back to the left side of the heart. The **systemic circuit** transports blood pumped under higher pressure from the heart\'s left side to all parts of the body not served by the pulmonary circuit. Upon return to the heart, blood from the systemic circuit enters the heart\'s right side (ie, starting point of the pulmonary circuit). In a healthy heart, blood passes through an atrium before entering a ventricle; consequently, blood is received by the heart into the atria and blood exits the heart through the ventricles. Specifically, oxygen-rich blood returning from the lungs via **pulmonary veins** enters the left atrium, which transfers this blood to the left ventricle to be pumped into the systemic circuit via the **aorta**. Likewise, oxygen-poor blood returning from the systemic circuit via the **venae cavae** enters the right atrium, where the blood is transferred to the right ventricle to be pumped back to the lungs via **pulmonary arteries** in the pulmonary circuit (see Figure 13.15). A blue check mark in a square Description automatically generated Chapter 13: Circulation 468 **Figure 13.15** Pathway of blood through the pulmonary and systemic circuits. **Concept Check 13.2** Identify and arrange in order the structures in the following list that blood in a pulmonary artery would pass through to reach the superior vena cava. aorta, aortic semilunar valve, bicuspid valve, left atrium, left ventricle, pulmonary semilunar valve, pulmonary vein, right atrium, right ventricle, superior vena cava, tricuspid valve [**Solution**](javascript:void(0)) 13.1.08 Blood Vessels Blood is transported through the body within an extensive system of blood vessels, collectively referred to as the vasculature. Different types of blood vessels, primarily **arteries**, **veins**, and **capillaries**, comprise  A blue check mark in a square Description automatically generated Chapter 13: Circulation 469 the vasculature. Small branches that diverge from arteries are called **arterioles**, and small vessels that converge to form veins are called **venules**. Figure 13.16 shows the general arrangement of the different blood vessel types within the vasculature and the order in which blood flows through these vessels. **Figure 13.16** Sequential arrangement of different types of blood vessels in the vasculature. All blood vessels have an internal lining called the **endothelium**, which is composed of a single layer of specialized [epithelial cells](javascript:void(0)) (ie, endothelial cells). The endothelium provides a slick surface that reduces friction between the vessel wall and the blood, facilitating smooth blood flow through the vessels. Endothelial cells also secrete a variety of chemical signals (eg, endothelin, nitric oxide) involved in regulating various aspects of cardiovascular system function, including control of blood vessel diameter, blood clotting, blood vessel permeability, formation of new blood vessels, and inflammation. Blood vessels other than capillaries typically have walls that, in addition to possessing an inner endothelial layer, have a middle layer containing smooth muscle cells and elastic fibers and an outer layer consisting of connective tissue, primarily collagen fibers. The relative thicknesses of these layers, which vary in different types of blood vessels (Figure 13.17), reflect the vessels\' functional differences.  Chapter 13: Circulation 470 **Figure 13.17** Blood vessel structure. Arteries, which function to carry blood away from the heart, have relatively thick walls that contain abundant smooth muscle cells, elastic fibers, and collagen fibers. This wall structure enables arteries to withstand the pressure exerted on blood during contraction (ie, systole) of the heart\'s ventricles. Similarly, the wall structure allows arteries to stretch, which stores energy to maintain blood\'s forward motion during periods in which the heart is relaxed (ie, diastole). Arteries typically carry oxygen-rich blood; however, arteries of the pulmonary circuit (ie, pulmonary arteries) carry oxygen-poor blood to the lungs so the blood can pick up oxygen. Veins carry blood toward the heart and have walls with relatively thin layers of smooth muscle and elastic tissue. The blood in veins is typically under low pressure; therefore, to prevent backflow of blood, many veins contain **valves** (see Figure 13.17). Furthermore, most veins transport oxygen-poor blood, an exception being veins that carry blood in the pulmonary circuit (ie, pulmonary veins). Because blood gains oxygen in the lungs, pulmonary veins carry oxygen-rich blood directly back to the heart from the lungs. Capillaries are the smallest type of blood vessel. The walls of capillaries are extremely thin and consist of a single layer of endothelial cells attached to a basement membrane. The structure of capillary walls facilitates exchange of materials between blood and interstitial fluid in body tissues. This movement of materials enables blood to supply oxygen and nutrients to body tissues and to remove cellular waste products from the tissues. 13.1.09 Blood Flow Regulation The circulatory system can efficiently distribute materials as needed to specific parts of the body (eg, organs) by regulating blood flow. By adjusting the amount of blood that flows to a particular body region, the body can vary the delivery of needed substances (eg, oxygen, nutrients, immune cells) to meet the changing demands for these substances in different parts of the body at different times. A diagram of a blood vessel Description automatically generated Chapter 13: Circulation 471 Blood flows in the circulatory system due to differences in pressure, that is, blood (like all fluids) moves down a pressure gradient (Δ*P*) from areas of higher pressure to areas of lower pressure. During systole of the cardiac cycle, pressure in the circulatory system is greatest within the heart, which causes blood to exit the heart and enter the vasculature, where pressure is lower. As blood moves through the vasculature, friction among blood cells and between the blood and blood vessel walls causes a continuous loss of pressure. Therefore, in the systemic circuit, blood pressure is highest in the aorta (ie, artery leading from the left ventricle) and lowest in the venae cavae (ie, veins leading into the right atrium), as depicted in Figure 13.18. **Figure 13.18** Blood pressure within different blood vessels of the systemic circuit. **Blood flow (*Q*)** is defined as the volume of blood that moves through a structure (eg, organ) in a given amount of time, and friction within the circulatory system opposes blood flow. The total amount of friction encountered by blood as it passes through the circulatory system is referred to as the **total peripheral resistance (TPR)**. TPR is influenced by the viscosity (ie, thickness) of blood as well as by the length and internal (ie, lumen) diameter of blood vessels. TPR increases when blood viscosity or blood vessel length increases, or when blood vessel diameter decreases. Because movement of blood through the circulatory system is driven by a pressure gradient (Δ*P*) and is opposed by resistance resulting from friction (ie, TPR), *Q* can be analyzed using the following equation (which is derived from [Ohm\'s law](javascript:void(0))): 𝑄 = ∆𝑃 TPR This equation shows that *Q* is directly proportional to Δ*P* (ie, *Q* increases as Δ*P* increases) and inversely proportional to TPR (ie, *Q* decreases as TPR increases). Although blood viscosity and blood vessel length may change over long time frames (eg, lengthening of blood vessels as an infant grows to adulthood), these variables typically remain constant over short periods of time (ie, hours). Therefore, TPR (and therefore, *Q*) is regulated primarily via changes to blood vessel diameter (ie, [vasoconstriction and vasodilation](javascript:void(0))). Figure 13.19 shows a hypothetical example of how blood flow can be adjusted to meet the needs of particular body regions by changes in blood vessel diameter.  Chapter 13: Circulation 472 **Figure 13.19** Regulation of blood flow via changes to blood vessel diameter. As blood passes through the circulatory system, the greatest drop in blood pressure occurs as blood passes through arterioles (see Figure 13.18), which indicates that arterioles account for the majority of TPR. Consequently, arterioles are the primary sites at which TPR is regulated via vasoconstriction and vasodilation. 13.1.10 Capillary Beds Capillaries are organized into networks called **capillary beds**. Each capillary bed typically receives blood from an arteriole and passes the blood to a venule, with blood flow (ie, microcirculation) through the capillary bed determined by the diameter of the supplying arteriole. In addition, entrances to some capillaries are encircled by smooth muscle cells that form structures called **precapillary sphincters**. Precapillary sphincters can constrict, causing blood to bypass the capillary bed (see Figure 13.20). In this way, blood flow through capillary beds can be closely matched to the metabolic activity level of the surrounding tissues. A diagram of a condition Description automatically generated Chapter 13: Circulation 473 **Figure 13.20** Regulation of blood flow through capillary beds. After being pumped from the heart, blood generally passes through only one capillary bed before returning to the heart. However, in a few locations within the body (eg, intestines, [pituitary gland](javascript:void(0)), [kidney](javascript:void(0))), blood received from one set of capillaries is carried by one or more larger vessels to a second set of capillaries, through which this blood passes before returning to the heart via the systemic circuit. Such pathways that involve two capillary beds arranged in series are called **portal systems** and allow substances to be transported from one body region to another without being diluted or widely distributed. Blood in capillaries moves at a slower velocity than blood in other types of vessels. This slow movement of blood increases the efficiency with which materials are exchanged across capillary walls between blood and interstitial fluid because the amount of time during which diffusion can occur is increased. Blood velocity is slowest in capillaries because the total (ie, combined) cross-sectional area of all capillaries is greater than the total cross-sectional areas of other blood vessel types (see Figure 13.21).  Chapter 13: Circulation 474 **Figure 13.21** Blood flow velocity and total cross-sectional area of different types of blood vessels. Exchange of materials between capillary blood and interstitial fluid occurs by [diffusion](javascript:void(0)), [endocytosis](javascript:void(0)), [exocytosis](javascript:void(0)), and bulk flow (ie, mass fluid transfer). Bulk flow is caused by **hydrostatic pressure (HP)** and **oncotic pressure (OP)** inside and outside capillaries. HP is the force a fluid exerts on a vessel wall (eg, blood pressure). OP (also known as colloid osmotic pressure) results from the presence of large molecules (eg, proteins) that do not freely cross the capillary wall, causing water to move from areas of lower OP to areas of higher OP by [osmosis](javascript:void(0)). As shown in Figure 13.22, the OP and HP of the interstitial fluid, as well as the OP of the blood, remain essentially unchanged as blood passes through a capillary. However, due to friction, the HP of blood *decreases* from the arterial end to the venous end of a capillary. Consequently, differences in net pressure at opposite ends of a capillary bed cause fluid to move from the blood to the interstitial fluid (ie, filtration) at the arterial end of the capillary bed and cause fluid to move into the blood from the interstitial fluid (ie, absorption) at the venous end of the capillary bed (Figure 13.22). A diagram of blood vessels Description automatically generated Chapter 13: Circulation 475 **Figure 13.22** Fluid transfer via bulk flow. Bulk flow of fluid out of and into capillaries plays an important role in determining the volume of fluid present in blood (ie, plasma volume) and in the interstitial space (ie, interstitial fluid volume). More fluid generally exits the blood via filtration into the interstitial space than is taken up by the capillaries via absorption. However, the **lymphatic system** functions to return excess interstitial fluid to the blood (as discussed in Concept 13.2.02); therefore, a higher filtration rate relative to absorption rate in capillary beds typically does not result in inappropriate accumulation of interstitial fluid, a condition known as **edema**. 13.1.11 Blood Pressure As discussed in Concept 13.1.09, blood flow through the circulatory system occurs due to a pressure gradient resulting from the heart\'s activity. Specifically, blood flow is driven by contraction (ie, systole) of the heart\'s ventricles, and blood pressure develops as a result of the resistance to blood flow that exists within the system due to friction among blood cells and between blood vessel walls and the blood. Arterial blood pressure resulting from ventricular systole, referred to as **systolic pressure**, causes elastic arteries near the heart to stretch, allowing these arteries to store energy. During ventricular relaxation (ie, diastole), the arteries that were stretched during systole recoil, releasing their stored energy and  Chapter 13: Circulation 476 generating pressure by pushing on the blood. This **diastolic pressure**, although lower than systolic pressure, maintains forward blood movement in the vasculature because backward blood movement is prevented by the semilunar valves in the heart (ie, valves between the ventricles and the pulmonary trunk and aorta). Systemic blood pressure in humans is typically [measured](javascript:void(0)) using a **sphygmomanometer**, which consists of an inflatable cuff and a pressure gauge. Blood pressure readings are reported as a ratio of systolic to diastolic pressures (see Figure 13.23), typically in units of millimeters of mercury (mm Hg). Normal blood pressure for healthy adults at rest is generally defined as less than 120/80 mm Hg. Blood pressure that is higher than normal is known as **hypertension**, while blood pressure that is lower than normal is known as **hypotension**. **Figure 13.23** Systolic versus diastolic blood pressure. As shown in Figure 13.24, blood pressure within arteries rises and falls in a regular pattern, which is due to alternating systole and diastole of the heart. These pressure fluctuations result in a detectable **pulse** (ie, rhythmic stretching and recoiling of arteries). **Pulse pressure** represents the difference between diastolic and systolic pressures in an artery, and **mean arterial pressure (MAP)** represents the average pressure in an artery during one cardiac cycle. MAP is not exactly midway between diastolic and systolic pressures because diastole typically lasts longer than systole; therefore, the following equation is often used to calculate MAP: MAP= Diastolic pressure+Pulse pressure A diagram of a heart and blood pressure Description automatically generated Chapter 13: Circulation 477 **Figure 13.24** Parameters of blood pressure. Arterial blood pressure varies based on blood flow into and out of arteries. If more blood enters than leaves the arteries in a given amount of time, blood volume in the arteries increases, causing blood pressure within the arteries to increase. Conversely, if more blood leaves than enters the arteries in a given amount of time, blood volume and blood pressure in the arteries decrease. Consequently, increased cardiac output (which increases blood flow into the arteries) or increased peripheral resistance due to arteriole vasoconstriction (which decreases blood flow out of the arteries) tends to raise arterial blood pressure, as does an increase in total blood volume in the circulatory system. Figure 13.25 summarizes factors that affect arterial blood pressure.  Chapter 13: Circulation 478 **Figure 13.25** Factors that produce changes in blood pressure. 13.1.12 Circulation and Thermoregulation Blood is composed mostly of water, which has a high [specific heat capacity](javascript:void(0)). Consequently, blood can carry a significant amount of heat. Distribution of heat within the body can be controlled via regulation of blood flow to specific body regions, allowing for **thermoregulation** (ie, maintenance of a physiologically appropriate internal body temperature). When internal body temperature rises, the cardiovascular system facilitates thermoregulation by increasing blood flow through capillary beds near the surface of the skin via vasodilation, allowing heat to be dissipated to the environment. Conversely, when internal body temperature falls, decreased blood flow to capillary beds at the body\'s surface via vasoconstriction allows heat to be retained in the body\'s core. Figure 13.26 shows how blood flow to surface capillary beds is regulated via vasodilation and vasoconstriction of arterioles supplying blood to these capillaries. A diagram of blood pressure Description automatically generated Chapter 13: Circulation 479 **Figure 13.26** Role of cardiovascular system in thermoregulation. 13.1.13 Endocrine and Nervous Control of Circulation The cardiovascular system is regulated by **extrinsic controls**, which are signals that originate in the nervous and endocrine systems and affect heart and blood vessel activity. In addition, local conditions (eg, extent of smooth muscle stretching, CO2 concentration) within an organ or tissue can influence local blood flow by affecting the diameter of arterioles supplying blood to capillary beds in the region, a mechanism referred to as **autoregulation**. As discussed in Concept 13.1.06, heart contraction is *not* initiated by signals outside the heart; however, the contraction *rate* and contraction *force* (ie, heart contractility) are regulated by extrinsic signals. Activity of the [autonomic nervous system (ANS)](javascript:void(0)) plays an important role in regulating heart function. Specifically, activity of the **sympathetic division** of the ANS increases heart rate and contractility via release of **norepinephrine** from neurons. Conversely, activity of the **parasympathetic division** of the ANS decreases heart rate (but has little effect on contractility) via release of **acetylcholine**. Hormones released by glands in the endocrine system also affect the function of the heart (see Lesson 11.2). **Epinephrine**, which is released by the [adrenal medulla](javascript:void(0)) in response to sympathetic nervous system (SNS) activity, stimulates the heart to beat faster and more forcefully. Likewise, the hormone **triiodothyronine**, which is released from the [thyroid gland](javascript:void(0)), increases heart rate by acting on the heart muscle directly and by making the heart more responsive to epinephrine and norepinephrine. Blood vessel vasodilation and vasoconstriction are also subject to neural and hormonal control. This regulation of blood vessel diameter is complex and involves an interplay of multiple factors, some of which produce opposite effects (ie, vasodilation versus vasoconstriction) depending on the context in which signals are received. Furthermore, blood vessels respond to a variety of chemical and physical stimuli via autoregulation. For example, **nitric oxide** released by endothelial cells in arteries and arterioles triggers vasodilation of these vessels in response to increased frictional force (ie, shear stress) experienced by endothelial cells during periods of increased blood flow. In addition, cell stretching caused by increased blood pressure in arteries and arterioles can trigger contraction of smooth muscle in the walls of these vessels, resulting in vasoconstriction 