Bio 108 Final Study Stuff PDF
Document Details
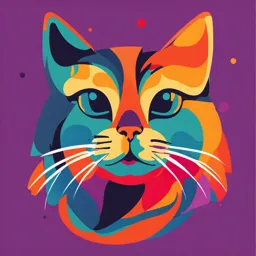
Uploaded by SincereSelkie3538
MacEwan University
Tags
Summary
This document contains study materials for a biology course, potentially for a final exam.
Full Transcript
B) Understanding the Following Concepts 1. Reproductive Isolation Reproductive isolation prevents gene flow between populations, allowing them to evolve independently and form distinct species. It is a key driver of speciation. 2. Speciation Speciation is the process by which one...
B) Understanding the Following Concepts 1. Reproductive Isolation Reproductive isolation prevents gene flow between populations, allowing them to evolve independently and form distinct species. It is a key driver of speciation. 2. Speciation Speciation is the process by which one population splits into two or more distinct species due to genetic divergence and reproductive isolation. 3. Divergence Divergence refers to the accumulation of genetic differences between populations, often driven by environmental pressures, genetic drift, or mutation. This can eventually lead to speciation. C) Definitions of Terms 1. Prezygotic Isolating Mechanisms Barriers that prevent fertilization from occurring. Examples include: ○ Temporal isolation (mating at different times) ○ Behavioral isolation (differences in mating behaviors) ○ Mechanical isolation (incompatibility of reproductive organs) ○ Gametic isolation (incompatibility of sperm and egg) 2. Postzygotic Isolating Mechanisms Barriers that occur after fertilization, preventing viable or fertile offspring. Examples include: ○ Hybrid inviability (offspring fail to develop properly) ○ Hybrid sterility (offspring are sterile, e.g., mule) ○ Hybrid breakdown (offspring of hybrids are weak or sterile) 3. Allopatry A condition where populations are geographically separated, preventing gene flow. This often leads to allopatric speciation. 4. Sympatry A condition where populations coexist in the same geographic area but are reproductively isolated due to mechanisms such as behavioral differences or polyploidy. 5. Macroevolution Evolutionary changes that occur above the species level, such as the formation of new taxa (genera, families). It involves large-scale changes that can span millions of years. 6. Polyploid A condition where an organism has more than two complete sets of chromosomes. Polyploidy can drive speciation, particularly in plants, where it creates reproductive isolation from the parent species. D) Descriptions 1. The Process of Allopatric Speciation ○ Allopatric speciation occurs when a population is geographically isolated by physical barriers (e.g., mountains, rivers, or distance). ○ Over time, genetic drift, mutation, and natural selection act on the isolated populations, leading to genetic divergence. ○ If the populations remain isolated for long enough, reproductive isolation evolves, and they become distinct species. 2. How Geographic Isolation Depends on Organismal Characteristics ○ Characteristics such as size, mobility, and dispersal ability determine how easily a population can become isolated. Example: A small, flightless bird may be isolated by a small river, whereas a large bird capable of flying long distances may not be. ○ Species with limited mobility or specific habitat requirements are more likely to undergo allopatric speciation. 3. The Link Between Microevolution and Macroevolution ○ Microevolution refers to small-scale changes within a population (e.g., allele frequency changes due to selection or drift). ○ Over time, microevolutionary changes can accumulate and result in significant differences between populations, leading to macroevolution (e.g., speciation or the evolution of new taxa). ○ The processes that drive microevolution—mutation, natural selection, drift—are the same forces that contribute to macroevolution, but macroevolution happens over longer timescales. 4. Autopolyploid Speciation ○ Autopolyploid speciation occurs when an individual within a single species undergoes genome duplication, creating a polyploid organism. ○ The polyploid individual is reproductively isolated from the parent population because it cannot produce viable offspring with diploid individuals. ○ This is common in plants. For example, a tetraploid plant (4n) cannot breed with a diploid plant (2n) but can self-fertilize or mate with other tetraploids, leading to a new species. 5. Hybridization Hybridization occurs when individuals from two distinct species or populations interbreed and produce offspring. The outcomes of hybridization depend on genetic compatibility and environmental conditions and can lead to new species, reinforcement, or fusion. 6. Reinforcement Reinforcement is the strengthening of prezygotic isolating mechanisms when hybrids have reduced fitness. This process occurs to prevent hybridization, ensuring that distinct species maintain their integrity. 7. Fusion Fusion occurs when reproductive barriers between two species are weak, allowing them to merge back into a single species through extensive gene flow. 8. Macroevolution Macroevolution refers to large-scale evolutionary changes that result in the emergence of new species, genera, families, or higher taxa. It often involves patterns like adaptive radiation or extinction events over long timescales. 9. Abiogenesis Abiogenesis is the hypothesis that life originated from non-living chemical compounds through natural processes, without requiring pre-existing life forms. It explores how simple molecules formed complex organic compounds, leading to the first living cells. 10. Panspermia Panspermia is the hypothesis that life originated elsewhere in the universe and was transported to Earth via meteoroids, asteroids, comets, or interstellar dust. This concept suggests life may be widespread across the cosmos. 11. The Origin of Heavy Elements Heavy elements (like carbon, oxygen, nitrogen) were formed through nuclear fusion in stars and supernova explosions. These elements are essential for life and were incorporated into the materials that formed Earth. C) Definitions of Terms 1. Sympatry Sympatry refers to populations or species that live in the same geographic area but remain reproductively isolated due to ecological, behavioral, or genetic factors. 2. Hybrid A hybrid is an offspring resulting from the interbreeding of two different species or genetically distinct populations. Hybrids may be fertile (capable of reproduction) or sterile (unable to reproduce, e.g., mules). 3. Polyploid Polyploid organisms have more than two sets of chromosomes. This condition often leads to reproductive isolation and speciation, particularly in plants. 4. Hybrid Zone A hybrid zone is a region where individuals from two distinct species or populations meet, interbreed, and produce hybrid offspring. The stability and outcomes of hybrid zones depend on hybrid fitness and gene flow. 5. Ribozyme A ribozyme is an RNA molecule capable of acting as an enzyme to catalyze chemical reactions. Ribozymes are crucial to the RNA world hypothesis, which suggests RNA played a central role in the origin of life. 6. Vesicle A vesicle is a small, membrane-bound sac composed of lipid bilayers. Vesicles are thought to have been critical in early life, serving as primitive cell membranes that enclosed and protected genetic material and biochemical reactions. D) Descriptions 1. The Relationship Between Natural Selection and Different Outcomes for Hybrids in Hybrid Zones ○ Reinforcement: If hybrids are less fit than their parent species, natural selection favors individuals with strong prezygotic barriers, reducing hybridization. ○ Fusion: If hybrids are equally fit, gene flow between species can erase distinctions, causing species to merge. ○ Stability: If hybrids are viable and occupy a unique niche, the hybrid zone may persist, maintaining genetic diversity. 2. Conditions on Earth as It First Formed and Cooled ○ Early Earth (~4.6 billion years ago) was molten and inhospitable, with intense volcanism, asteroid impacts, and a lack of oxygen. ○ As Earth cooled, water vapor condensed to form oceans. The atmosphere contained gases like methane, ammonia, hydrogen, and water vapor, creating conditions favorable for chemical evolution. 3. The Scientific Hypotheses for How Life on Earth First Formed ○ Abiogenesis: Life arose from simple organic molecules formed through chemical reactions in early Earth’s environment. Key steps include: Formation of organic molecules (e.g., amino acids, nucleotides) possibly facilitated by lightning or hydrothermal vents (Miller-Urey experiment). Assembly of complex molecules like proteins and nucleic acids. Formation of protocells—membrane-bound vesicles containing self-replicating molecules. ○ Panspermia: Life’s building blocks or even microorganisms arrived on Earth from space via meteorites or comets. 4. Why Scientists Propose RNA as the First Molecule of Inheritance ○ RNA can store genetic information like DNA and catalyze reactions like proteins. ○ Ribozymes demonstrate that RNA can self-replicate and drive biochemical reactions, supporting the idea of an “RNA world.” ○ RNA likely predated DNA and proteins, serving dual roles in early life before specialization into the modern DNA-RNA-protein system. B) Understanding the Following Concepts 1. Macroevolution ○ Macroevolution refers to large-scale evolutionary changes above the species level. ○ It includes processes such as speciation, adaptive radiation, and extinction, as well as trends seen over geological time scales, such as the emergence of major taxonomic groups. ○ Evidence for macroevolution comes from comparative anatomy, molecular biology, biogeography, and the fossil record. 2. Bias in the Fossil Record ○ The fossil record is incomplete and biased due to several factors: Organismal Bias: Hard-bodied organisms (e.g., those with shells, bones) fossilize more readily than soft-bodied ones. Habitat Bias: Organisms in environments conducive to fossilization (e.g., sedimentary basins) are more likely to be preserved. Temporal Bias: Older fossils are less likely to be preserved due to geological processes like erosion and plate tectonics. Discovery Bias: The likelihood of finding fossils depends on where paleontologists search and how accessible a site is. 3. Relative vs. Absolute Age ○ Relative Age: Determines the sequential order of events or fossils without assigning specific dates. This is done using stratigraphy (studying rock layers) and principles like superposition (older layers are beneath younger layers). ○ Absolute Age: Determines the actual age of a fossil or rock using radiometric dating, which measures the decay of radioactive isotopes (e.g., carbon-14 or uranium-238). C) Definitions of Terms 1. Fossil ○ A fossil is the preserved remains, impression, or trace of an organism that lived in the past, often embedded in sedimentary rock. ○ Fossils provide critical evidence of ancient life and evolutionary processes. 2. Sedimentary Rock ○ Sedimentary rocks are formed from the accumulation and compression of mineral and organic particles. ○ They are the primary rock type where fossils are found because the layers can preserve organisms and their traces. D) Descriptions 1. Radiometric Dating of Fossils ○ Radiometric dating determines the absolute age of fossils by measuring the decay of radioactive isotopes. ○ Key steps: 1. Isotopes like carbon-14 (for recent fossils) or uranium-238 (for older rocks) decay at known rates, called half-lives. 2. The ratio of parent isotope to daughter product is measured to calculate the fossil's age. 3. Example: If half of the carbon-14 in a sample has decayed, the fossil is approximately 5,730 years old (one half-life of carbon-14). ○ Limitations: Carbon dating is only effective for fossils up to ~50,000 years old; older fossils require isotopes with longer half-lives, like potassium-40 or uranium-238. 2. The Sequence of Geologic Eons, Eras, and Periods (Bonus) ○ Eons (Largest Timescale): 1. Hadean (4.6–4.0 billion years ago): Formation of Earth; no life. 2. Archean (4.0–2.5 billion years ago): First life (prokaryotes), formation of stromatolites. 3. Proterozoic (2.5 billion–541 million years ago): Oxygen accumulation (Great Oxygenation Event), first eukaryotes. 4. Phanerozoic (541 million years ago–present): Abundant fossil evidence; includes the current eon. ○ Eras within the Phanerozoic: 1. Paleozoic (541–252 million years ago): Cambrian Explosion, origin of vertebrates, early plants. Ends with the Permian Extinction. 2. Mesozoic (252–66 million years ago): Age of dinosaurs, first mammals, flowering plants. Ends with the Cretaceous Extinction. 3. Cenozoic (66 million years ago–present): Age of mammals and birds; evolution of humans. ○ Periods within the Paleozoic, Mesozoic, and Cenozoic Eras: 1. Examples: Cambrian, Jurassic, Quaternary (too numerous to fully list here, let me know if you'd like a more detailed table!). 3. Major Causes of Mass Extinctions ○ There have been five major mass extinctions in Earth's history, each wiping out a significant portion of species: 1. Ordovician-Silurian Extinction (~443 million years ago): Likely caused by a sudden glaciation event and a drop in sea levels. 2. Late Devonian Extinction (~372–359 million years ago): Possibly due to global anoxia (oxygen depletion in oceans), climate change, or asteroid impacts. 3. Permian-Triassic Extinction (~252 million years ago): Known as "The Great Dying," it was likely caused by massive volcanic eruptions (Siberian Traps) leading to global warming, ocean acidification, and anoxia. 4. Triassic-Jurassic Extinction (~201 million years ago): Likely caused by volcanic activity, climate change, and rising CO2 levels. 5. Cretaceous-Paleogene Extinction (~66 million years ago): Caused by an asteroid impact (Chicxulub crater) and massive volcanic activity, leading to the extinction of non-avian dinosaurs. ○ Mass extinctions create evolutionary opportunities, allowing surviving species to diversify (e.g., mammals after the Cretaceous extinction). B) Understanding the Following Concepts 1. Systematics ○ The scientific study of the diversity of organisms and their evolutionary relationships. ○ Systematics combines taxonomy (classification) and phylogenetics (study of evolutionary relationships) to create a comprehensive picture of life’s history. 2. Monophyly ○ A monophyletic group (or clade) consists of an ancestor and all its descendants. ○ Monophyly is the ideal classification in systematics because it reflects true evolutionary relationships. 3. Cladistics ○ A method of systematics that classifies organisms based on shared derived characters (synapomorphies). ○ It focuses on reconstructing phylogenies using branching diagrams (cladograms) to depict evolutionary relationships. 4. Maximum Parsimony ○ A principle used in phylogenetics to find the simplest evolutionary explanation for observed traits. ○ It assumes the tree with the fewest evolutionary changes (mutations, trait gains/losses) is the most likely. 5. Phylogenies as Hypotheses ○ Phylogenetic trees are hypotheses about evolutionary relationships based on available data (e.g., morphology, DNA). ○ They are tested and refined as new evidence emerges, making them dynamic rather than definitive. 6. Sister Taxa ○ Sister taxa are two groups that share an immediate common ancestor and are each other’s closest relatives. ○ For example, in a tree showing primates, humans and chimpanzees are sister taxa. 7. Plate Tectonics ○ The movement of Earth's lithospheric plates, which shapes continents and oceans over time. ○ Plate tectonics has driven events like continental drift, the formation of mountain ranges, and the isolation or merging of populations, influencing evolution and biogeography. C) Definitions of Terms 1. Anagenesis ○ Evolutionary change within a single lineage over time without branching into new species. ○ Example: Gradual transformation of one species into another. 2. Cladogenesis ○ Evolutionary branching, where one lineage splits into two or more distinct species. ○ Cladogenesis is responsible for biodiversity and is depicted in phylogenetic trees. 3. Taxon (plural: Taxa) ○ A taxonomic group of organisms at any level of classification (e.g., species, genus, family). ○ Example: Homo sapiens is a taxon at the species level. 4. Phylogenetics ○ The study of evolutionary relationships among species or groups using data from morphology, genetics, or molecular biology. 5. Clade ○ A monophyletic group that includes an ancestor and all its descendants. ○ Example: Mammals are a clade within vertebrates. 6. Branch Point/Node ○ Represents a common ancestor where a lineage splits into two or more lineages on a phylogenetic tree. 7. Polytomy ○ A branch point on a phylogenetic tree where more than two lineages emerge. ○ Polytomies indicate unresolved relationships due to insufficient or conflicting data. 8. Ingroup ○ The group of species being studied in a phylogenetic analysis, typically compared to an outgroup to identify derived traits. 9. Continental Drift ○ The gradual movement of continents over geological time due to plate tectonics. ○ Continental drift has driven species isolation (allopatry), extinctions, and adaptive radiations. D) Descriptions 1. Major Causes of Mass Extinctions ○ Catastrophic Events: Volcanic eruptions (e.g., Siberian Traps during the Permian-Triassic extinction). Asteroid impacts (e.g., Chicxulub impact during the Cretaceous-Paleogene extinction). ○ Environmental Changes: Climate change (e.g., ice ages, global warming). Ocean anoxia (oxygen depletion). ○ Biological Factors: Emergence of new predators or competition. 2. How Mass Extinction Can Affect the Evolution of Organisms ○ Mass extinctions eliminate large numbers of species, opening ecological niches. ○ Surviving species often undergo adaptive radiation, diversifying to exploit these empty niches. ○ Example: Mammals flourished and diversified after the extinction of non-avian dinosaurs. 3. The Function of the Outgroup in Cladistic Analysis ○ An outgroup is a species or group known to be less closely related to the ingroup than the ingroup species are to each other. ○ It helps identify shared derived traits (synapomorphies) within the ingroup and distinguishes them from ancestral traits. 4. Differences Between Mono-, Para-, and Polyphyletic Clades ○ Monophyletic: Includes a common ancestor and all its descendants (e.g., birds as a clade within reptiles). ○ Paraphyletic: Includes a common ancestor and some, but not all, of its descendants (e.g., reptiles excluding birds). ○ Polyphyletic: Includes groups with different ancestors, often based on convergent traits (e.g., grouping bats and birds due to flight). 5. How to Make and Test Predictions Derived from Phylogenetic Hypotheses ○ Making Predictions: Use phylogenetic trees to hypothesize trait distributions or evolutionary events. Example: If two species are closely related, they might share similar traits or genetic sequences. ○ Testing Predictions: Collect new data, such as fossil evidence or molecular sequences, to confirm or refine the tree. If a tree predicts a trait in a common ancestor, the fossil record or genetic evidence should reflect it. 6. Difference Between Shared Derived and Shared Ancestral Characters ○ Shared Derived Characters (Synapomorphies): Traits that are unique to a clade and not present in distant ancestors. Example: Hair in mammals. ○ Shared Ancestral Characters (Plesiomorphies): Traits present in a common ancestor and shared by multiple groups, including those outside the clade. Example: Vertebrae in mammals and other vertebrates. Understanding the Following Concepts 1. Horizontal Gene Transfer (HGT) ○ The movement of genetic material between organisms without reproduction. ○ Common in prokaryotes and occurs through mechanisms like transformation, transduction, and conjugation. ○ It plays a major role in the evolution of antibiotic resistance and the exchange of beneficial traits. 2. Antibiotic Resistance ○ The ability of bacteria to survive and reproduce despite the presence of antibiotics. ○ Often acquired through horizontal gene transfer or mutations. ○ Overuse and misuse of antibiotics in medicine and agriculture accelerate resistance. 3. Monophyly ○ A group consisting of an ancestor and all its descendants (e.g., mammals). ○ Monophyletic groups accurately represent evolutionary relationships. 4. Paraphyly ○ A group that includes a common ancestor but not all its descendants (e.g., reptiles excluding birds). ○ Paraphyletic classifications can obscure evolutionary relationships. 5. Endosymbiosis ○ A theory explaining how eukaryotic cells evolved. ○ Suggests mitochondria and chloroplasts originated as free-living prokaryotes that were engulfed by a host cell. ○ Evidence includes: Double membranes around mitochondria and chloroplasts. Their own circular DNA and ribosomes. Reproduction similar to prokaryotic binary fission. 6. The Evolution of Multicellularity ○ Multicellularity evolved independently in multiple lineages (e.g., plants, animals, fungi). ○ It allowed specialization of cells into tissues and organs. ○ Early multicellular organisms were likely colonial (e.g., Volvox). 7. The Biological Carbon Pump ○ A process in the ocean where photosynthetic plankton fix CO2 into organic carbon, which sinks to the deep ocean when organisms die. ○ It regulates atmospheric CO2 levels and plays a critical role in Earth’s carbon cycle. C) Definitions of Terms 1. Peptidoglycan ○ A polymer that makes up the cell walls of most bacteria, providing structural support. ○ It is a target for antibiotics like penicillin. 2. Transformation ○ A form of horizontal gene transfer where prokaryotes take up free DNA from their environment. 3. Transduction ○ Horizontal gene transfer mediated by viruses (bacteriophages), which transfer DNA between bacteria. 4. Conjugation ○ A process where prokaryotes transfer DNA directly between cells through a pilus. 5. Primary Productivity ○ The rate at which autotrophs (e.g., plants, algae) convert light or chemical energy into organic compounds, forming the base of the food web. 6. Unicellularity ○ The state of being composed of a single cell, characteristic of prokaryotes and many protists. 7. Coloniality ○ A form of organization where individual cells live in a group but remain largely independent (e.g., Volvox). 8. Multicellularity ○ The state where cells are specialized and interdependent, characteristic of plants, animals, and fungi. 9. Mixotrophy ○ A nutritional strategy combining autotrophy (e.g., photosynthesis) and heterotrophy (e.g., consuming organic matter). ○ Example: Euglena. 10. Secondary Endosymbiosis Occurs when a eukaryotic cell engulfs another eukaryotic cell that has already undergone primary endosymbiosis (e.g., some protists like dinoflagellates). D) Descriptions 1. The Differences and Similarities Among the Three Domains of Life ○ Bacteria: Prokaryotic, have peptidoglycan in their cell walls, diverse metabolic pathways. ○ Archaea: Prokaryotic, no peptidoglycan, unique membrane lipids, thrive in extreme environments. ○ Eukarya: Eukaryotic, have membrane-bound organelles, larger and more complex cells. ○ Similarities: All domains share a common genetic code, ribosomes, and basic metabolic pathways. 2. Why Prokaryotes Are the Most Successful Organisms on Earth ○ High reproductive rates and adaptability to diverse environments. ○ Ability to metabolize a wide range of substances. ○ Capacity for horizontal gene transfer enhances genetic diversity. 3. How Prokaryotes Have Changed the Earth’s Atmosphere ○ Cyanobacteria produced oxygen through photosynthesis, leading to the Great Oxygenation Event (~2.5 billion years ago). ○ Prokaryotes continue to influence the nitrogen, carbon, and sulfur cycles. 4. The Full Range of Nutritional Modes ○ Autotrophs: Use inorganic sources of carbon (e.g., CO2). Photoautotrophs: Use light energy (e.g., cyanobacteria). Chemoautotrophs: Use chemical energy (e.g., sulfur bacteria). ○ Heterotrophs: Use organic carbon sources. Photoheterotrophs: Use light energy but require organic carbon (e.g., purple non-sulfur bacteria). Chemoheterotrophs: Use organic molecules for both energy and carbon (e.g., most animals). ○ Mixotrophs: Combine autotrophy and heterotrophy (e.g., Euglena). 5. The Ecological Roles of Prokaryotes ○ Decomposers recycle nutrients in ecosystems. ○ Nitrogen-fixing bacteria (e.g., Rhizobium) make atmospheric nitrogen available to plants. ○ Symbiotic relationships with hosts (e.g., gut microbiota in humans). 6. The Theory of Endosymbiosis and Supporting Evidence ○ Mitochondria and chloroplasts originated from engulfed prokaryotes. ○ Evidence: Organelles have their own DNA and ribosomes. Organelles divide independently of the host cell. Their membranes and metabolic pathways resemble those of prokaryotes. 7. The Ecological Roles of Protists ○ Primary Producers: Algae form the base of aquatic food webs. ○ Decomposers: Some protists break down organic matter. ○ Symbionts: Protists like zooxanthellae live in mutualistic relationships with coral. ○ Pathogens: Some cause diseases (e.g., Plasmodium causes malaria). 8. How Diffusion Is Related to the Evolution of Multicellularity ○ Diffusion limits the size of unicellular organisms because it becomes inefficient over large distances. ○ Multicellularity allows specialization, with certain cells handling nutrient acquisition and distribution. ○ Larger, multicellular organisms developed circulatory systems to overcome diffusion constraints. Understanding the Following Concepts 1. The Biological Carbon Pump ○ A critical process in the ocean where phytoplankton fix atmospheric CO₂ during photosynthesis. ○ When these organisms die or are consumed, their organic carbon sinks to deeper ocean layers, sequestering carbon for long periods and reducing atmospheric CO₂. ○ Plays a role in regulating Earth’s climate. 2. Alternation of Generations ○ A life cycle characteristic of plants and some algae where organisms alternate between two multicellular stages: Sporophyte (2n): Produces haploid spores by meiosis. Gametophyte (n): Produces gametes by mitosis. ○ These stages alternate, ensuring genetic diversity and adaptation. 3. Dominant Generation ○ Refers to the stage in the alternation of generations that is larger and longer-lived. ○ Example: In nonvascular plants (e.g., mosses), the gametophyte is dominant. In vascular plants (e.g., ferns, gymnosperms, angiosperms), the sporophyte is dominant. 4. Pollination ○ The transfer of pollen from the male structure (anther) to the female structure (stigma) of a plant. ○ Enables fertilization in seed plants. 5. Pollination Syndrome ○ The set of traits in flowers (e.g., shape, color, scent) that attract specific pollinators (e.g., bees, birds, bats). ○ Reflects co-evolution between plants and pollinators. 6. Modular Body Plan ○ Plants and fungi grow as a collection of repeating units (modules), allowing for indeterminate growth. ○ Example: Roots, shoots, or fungal hyphae extend as needed based on environmental conditions. 7. Absorptive Heterotrophy ○ A mode of nutrition where organisms (e.g., fungi) secrete enzymes into their environment to break down organic material and absorb the nutrients. 8. Facultative Asexuality ○ The ability of some organisms to reproduce asexually under certain conditions and sexually under others. ○ Common in fungi and plants, this strategy allows flexibility in reproduction depending on environmental factors. C) Definitions of Terms 1. Primary Productivity ○ The rate at which autotrophs (e.g., plants, algae) convert light or chemical energy into organic compounds, forming the base of the food chain. 2. Myxotrophy ○ A nutritional strategy combining autotrophy (e.g., photosynthesis) and heterotrophy (e.g., consuming organic material). ○ Example: Protists like Euglena. 3. Secondary Endosymbiosis ○ When a eukaryotic cell engulfs another eukaryote that has already undergone primary endosymbiosis (e.g., dinoflagellates acquiring chloroplasts from red algae). 4. Apical Meristem ○ Regions of actively dividing cells at the tips of roots and shoots in plants. ○ Responsible for primary growth (lengthening of the plant). 5. Cuticle ○ A waxy, waterproof layer covering the epidermis of plants, reducing water loss and protecting against desiccation. 6. Mycelium ○ The mass of hyphae that forms the body of a fungus. ○ It grows through and absorbs nutrients from the substrate. 7. Hypha ○ A single filament of fungal mycelium. ○ Hyphae are the main mode of fungal growth and nutrient absorption. 8. Karyogamy ○ The fusion of two haploid nuclei to form a diploid nucleus during sexual reproduction in fungi. 9. Plasmogamy ○ The fusion of cytoplasm from two fungal cells during sexual reproduction, resulting in a heterokaryotic stage. 10. Heterokaryotic ○ A fungal cell or mycelium containing two or more genetically distinct nuclei within the same cytoplasm. ○ Occurs after plasmogamy and before karyogamy in fungal reproduction. D) Descriptions 1. The Costs and Benefits of Living on Land for Photoautotrophs ○ Benefits: Greater access to sunlight for photosynthesis. Higher concentrations of CO₂ in the air than in water. Fewer herbivores and pathogens (initially). ○ Costs: Risk of desiccation (water loss). Need for structural support (gravity). Challenges in dispersing gametes and offspring without water. 2. The Importance of Coastal Areas for the Evolution of Terrestriality in Land Plants ○ Coastal environments offered fluctuating conditions (e.g., tides, drying) that selected for adaptations to land (e.g., cuticles, stomata). ○ Provided access to both aquatic and terrestrial habitats, facilitating the transition. 3. Why the Evolution of Plants Can Be More Accurately Called Adaptation to Life “in Air” Rather Than “on Land” ○ Key challenges for plants (e.g., desiccation, gas exchange, UV radiation) are related to air, not soil. ○ Adaptations like cuticles, stomata, and vascular tissues address atmospheric rather than terrestrial constraints. 4. The Constraints on the Fungus Body Plan Imposed by Their Mode of Nutrition ○ Fungi secrete enzymes to digest food externally, requiring large surface areas for absorption. ○ This necessitates the filamentous hyphal structure, which maximizes contact with the substrate. ○ Limits fungi to environments where nutrients are accessible and moist. 5. A Generalized Fungal Life Cycle ○ Asexual Phase: Fungi produce haploid spores via mitosis, which germinate into new mycelia. ○ Sexual Phase: Plasmogamy: Fusion of cytoplasm from two mating types. Heterokaryotic Stage: Mycelium contains nuclei from both parents. Karyogamy: Fusion of nuclei forms a diploid zygote. Meiosis: Produces haploid spores that germinate into mycelia. 6. The Ecological Roles of Fungi ○ Decomposers: Break down organic material, recycling nutrients. ○ Mutualists: Form symbiotic relationships (e.g., mycorrhizae with plant roots, lichens with algae). ○ Pathogens: Cause diseases in plants and animals (e.g., rusts, athlete’s foot). 7. The Importance of Fungi to Humans ○ Food and Beverages: Fungi are used in bread, beer, wine, and cheese production. ○ Medicine: Source of antibiotics (e.g., penicillin) and immunosuppressants (e.g., cyclosporin). ○ Biotechnology: Used in bioremediation and industrial processes. ○ Agriculture: Mycorrhizal fungi enhance plant growth, though some fungi (e.g., rusts) are pests.