UCL Biomaterials (I) Lecture 3 - Cartilage Regeneration PDF
Document Details
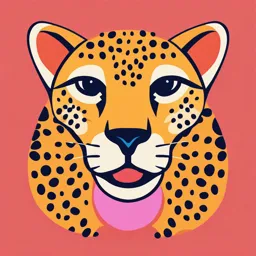
Uploaded by SparklingLoyalty
UCL
Dr Rana Khalife
Tags
Summary
This lecture covers Biomaterials (I) and Cartilage Regeneration by Dr Rana Khalife at UCL. Topics include the causes of cartilage defects, cartilage structure in the human body, current regeneration strategies, and biomaterials used in the process.
Full Transcript
Lecture 3 Biomaterials (I) Cartilage Regeneration Dr Rana Khalife 1 Outline 1. The clinical problem (What are the main causes of cartilage defects?) 2. Cartilage in the human body 3. Current strategies to regenerate cartilage 4. Biomat...
Lecture 3 Biomaterials (I) Cartilage Regeneration Dr Rana Khalife 1 Outline 1. The clinical problem (What are the main causes of cartilage defects?) 2. Cartilage in the human body 3. Current strategies to regenerate cartilage 4. Biomaterials used in cartilage regeneration 2 1. The Clinical Problem - Causes Accidents ❑ Sport injuries ❑ Direct blow – heavy impact on the joint ❑ Wear and tear – long periods of stress (overweight) ❑ Lack of movement – long periods of inactivity or immobility Diseases ❑ Some types of arthritis ❑ Osteoarthritis – mainly in hands, knees, hips and spine ❑ Costochondritis – inflammation of the cartilage that connects a rib to the breastbone ❑ Tumours that involve cartilage removal ❑ Other genetic disorders ❑ The most common cause of disability, representing around 6% of disabled people of 30 years and older 3 2. Cartilage Structure in the Human Body Articular cartilage does not have blood vessels, nerves, or lymphatics It is composed of a dense extracellular matrix (ECM) with a sparse distribution of highly specialized cells called chondrocytes The ECM is principally composed of water, collagen, and Each zone varies in structural, proteoglycans, and other functional and mechanical non-collagenous proteins properties, responds to different and glycoproteins stimuli and the cells in each zone 4 secrete different proteins 2. Cartilage Structure in the Human Body From the superficial zone to the deep zone, the proteoglycan content gradually increases. In the superficial zone, the collagen fibers are aligned parallel to the surface. Collagen fibers in the middle zone are unaligned and tangential to the cartilage surface. In the deep zone, collagen fibers are arranged radially. The collagen fibers in the calcified zone tend to arborize with little organization and mineralization. 5 Cartilage: The three types of cartilage 1. Hyaline cartilage This type of cartilage has a glassy appearance when fresh, hence its name, as hyalos is greek for glassy. It looks slightly basophilic overall in H&E sections. Hyaline cartilage has widely dispersed fine collagen fibres (type II), which strengthen it. most common, found in the ribs, nose, larynx, trachea. It is a precursor of bone. 6 Cartilage: The three types of cartilage 2. Fibrocartilage This is the strongest kind of cartilage, because it has alternating layers of hyaline cartilage matrix and thick layers of dense collagen fibres oriented in the direction of functional stresses. It is found in invertebral discs, joint capsules, ligaments 7 Cartilage: The three types of cartilage 3. Elastic cartilage In elastic cartilage, the chondrocytes are found in a threadlike network of elastic fibres within the matrix. Elastic cartilage provides strength, and elasticity, and maintains the shape of certain structure such as the external ear, epiglottis and larynx. 8 Cartilage ECM Composition in the Human Body The extracellular matrix surrounds each chondrocyte and they rarely communicate by direct signals Component Percentage Function Water 65 - 80 % wet weight Transport and distribute, lubrication, withstanding loads Collagen 60 % dry weight Provides shear and tensile properties, stabilising the matrix Proteoglycans 10 – 15 % dry weight Osmotic properties, ability to resist compressive loads, interaction with collagen hyaluronic acid, 25 – 30 % dry weight Organisation and maintenance of the glycosaminogly macromolecular structure of the cans, and extracellular matrix elastin 9 Mechanism of Cartilage Formation - Chondrogenesis During skeletal development, chondrocytes arise from MSCs to synthesise the templates for the developing bone Chondrogenesis occurs as a result of condensation of MSCs, which express collagens I, III and V, and chondroprogenitor cell differentiation with expression of cartilage-specific collagens II, IX, and XI 10 Cartilage Damage - Osteoarthritis The main function of cartilage is to produce a low friction surface capable of withstanding load, thus protecting the underlying bone However, it has limited capacity for repair… There is a finely regulated balance of degradation and synthesis – this leads to major long-term problems Early in the process of damage there is a rapid loss of glycosaminoglycans (the collagen network can not retain them!) Collagen damage is difficult to repair (low rate of matrix synthesis) Time and mechanical wear - chronic and progressive degenerative joint disease - osteoarthritis 11 Cartilage Damage Thinning of the Loss of articular chondrocytes cartilage Inflammatory Degradation of repair response collagen Formation of hematoma (dark area) Types based on the depth of injury: (A) Micro-damage - blunt trauma to the chondrocytes and matrix without visible disruption of the articular cartilage surface (B) Chondral fracture - disruption of the articular cartilage of variable depth down to the calcified tidemark (C) Osteochondral fracture with a penetrating fracture through the articular cartilage and into the subchondral bone Ulrich-Vinther, M. et al. 2003. Articular Cartilage Biology. Journal of the American Academy of 12 Orthopaedic Surgeons. 11 (6), pp. 421-430 Cartilage Damage and Repair Repair mechanisms: Inhibitors of apoptosis - reduce tissue damage after traumatic injury to the joint surface and decrease the potential for late osteoarthritic effects Proliferative response of surviving cells in the region of the injury to repair the tissue – increased production of type II collagen and matrix macromolecules Organisation of the hematoma into a fibrin clot that develops into a fibrovascular reparative tissue – MSCs from the underlying bone marrow proliferate and undergo chondrocyte differentiation Ulrich-Vinther, M. et al. 2003. Articular Cartilage Biology. Journal of the American Academy of 13 Orthopaedic Surgeons. 11 (6), pp. 421-430 3. Current Strategies for Cartilage Regeneration Noncell-based therapies ❑ Debridement Limitations Donor-site morbidity ❑ Marrow stimulation Poor quality of ❑ Mosaicplasty regenerated tissue ❑ Total joint replacement Tissue grafts and autologous transplantation - Limitations – Tissue overgrowth or thickening may require secondary surgery – Possible allergic response to the graft – Short-term discomfort and swelling, and potentially overall rejection of the graft 14 Tissue Engineering in Cartilage Regeneration There are two main approaches for regeneration of deficient osteochondral interface and full-thickness cartilage using tissue-engineering approaches: 1. One is to develop artificial cartilage constructs to mimic the architectural features, mechanical properties, and thus biological functions of native cartilage tissues. 2. The other tissue-engineering approach emphasizes more on regenerative medicine. 15 Tissue-engineering strategy for treatment of osteochondral interface and full- thickness cartilage defects with cell-laden hydrogel constructs. 16 https://www.sciencedirect.com/science/article/pii/S1742706117300363#f0005 Tissue Engineering in Cartilage Regeneration Classical TE approach (autologous chondrocyte implantation) Isolating cells Expanding cells in a monolayer to achieve adequate numbers Incorporating the expanded cells in a scaffold material Generation of neo-tissue after a further period of culture Scaffold-free implants Cells with no matrix are cultured and allowed to form aggregates - cartilaginous material (difficult to control the shape of the implant) Cells cultured first in an alginate gel where a matrix forms - cells are then released and cast as a cell slurry into a membrane insert or mold, where after a period of further culture a cartilaginous implant is formed (shape and thickness control) 17 Challenges of Tissue Engineered Cartilage Modulating chondrocyte phenotypes ❑ Scaffold material selection ❑ Mechanical stimulation ❑ Growth factor addition ❑ Genetic modification Recreating the complex architecture of cartilage ❑ Correct structural arrangement ❑ Correct ratio of the matrix components Obtaining successful integration of the newly formed tissue ❑ Cartilage-cartilage and cartilage-bone interactions Appropriate biomechanical properties of the final implant ❑ Obtaining a mechanically sound tissue 18 Challenges of Cartilage Regeneration Reminder! – Interface between tissues Junction between mechanically dissimilar materials – such as cartilage and bone The morphological/mechanical gradients at interfaces minimise stress Presence of ‘transition zones’ in tissue-engineered constructs is therefore essential The mechanical properties (e.g. stiffness) can be tailored using scaffolds with internal gradients of mineralisation 19 Challenges in Cartilage Formation in vitro As cell–cell contact is a prerequisite for chondrogenesis – will chondrogenic differentiation be as effective in a scaffold system, where the cell density is lower and the potential for cell–cell contact is reduced? A fundamental question – Are cells cultured in vitro capable of integrating with the surrounding tissues? Another question is – What is the likelihood of in vitro cartilage forming teratomas? And finally – Is it possible to produce viable, functional and durable cartilage? 20 Scaffolds The scaffold should function as a template for cells It should allow for cell retention and accumulation of the extracellular matrix within its structure It should also bear the harsh and dynamic mechanical environment of the articular cartilage repair site The scaffold material will not cause any inflammatory and immunogenic responses The surface chemistry and topography of the material must be adequately engineered to optimise cell–material interactions A 3D structure is necessary for the conservation of the chondrocytic phenotype 21 Scaffolds (II) The geometric parameters of the scaffold (porosity, pore size interconnectivity) affect new tissue formation by influencing cell-seeding efficiency, nutrient and by-product diffusion and extracellular matrix (ECM) accumulation Enhanced mechanical properties that influence the differentiation and the phenotype of the seeded cells and thus the production of ECM The scaffold needs to be able to withstand forces applied during the initial joint loading as the tissue regenerates, while protecting the cells contained within from physical damage 22 4. Biomaterials in Cartilage Regeneration Biomaterials can be used to mimic cartilage due to its avascularity and low cell density Fundamental criteria Consistent mechanical properties with native cartilage ❑ Compressive strength ❑ Lack of friction Integration with adjacent cartilage Durability throughout patient’s lifetime Biodegradability (after providing support for cells, without adverse effects on the newly formed tissue) Biocompatibility 23 4. Biomaterials in Cartilage Regeneration Hydrogel-polymer networks (up to 80 % of cartilage is water!) Natural polymers ❑ Alginate ❑ Heparin ❑ Gelatine ❑ Agarose ❑ Silk ❑ Collagen ❑ Hyaluronan Synthetic polymers ❑ Polyglycolic acid (PGA) ❑ Polylactic acid (PLA) 24 Natural polymeric scaffolds The main aim of the scaffold is to mimic the cartilage extracellular matrix while supporting cell proliferation Natural polymeric scaffolds are made of proteins, polysaccharides or a mixture of the two They have highly hydrated 3D fibrous structures in which cells can be encapsulated or seeded They have specialized chemistry, influencing cell attachment, proliferation and phenotype Natural polymers are often modified to improve their handling, mechanical and degradation properties using crosslinking and surface functionalization 25 Alginate Based Hydrogels Porous alginate scaffold prepared using micro-fluidics Wang, C. et al. 2011. A highly organized three-dimensional alginate scaffold for cartilage tissue engineering prepared by microfluidic technology. Biomaterials. 32, pp. 7118-7126 Some highly organized structures can be achieved using different fabrication techniques They are compromised because of their mechanical features (soft matrix) Their use is limited for in vitro generated chondrogenic tissue 26 Alginate Based Hydrogels Chondrocytes attached to the porous structure of the scaffold Wang, C. et al. 2011. A highly organized three-dimensional alginate scaffold for cartilage tissue engineering prepared by microfluidic technology. Biomaterials. 32, pp. 7118-7126 Chondrocytes are shown to maintain their normal phenotypes within the scaffold They also secrete a great deal of extracellular matrix when seeded in the alginate scaffold 27 Hyaluronan Hyaluronan scaffolds modulate the phenotype and behavior of articular chondrocytes Higher molecular weight hyaluronan leads to a greater collagen fiber maturation It can increase Porous hyaluronan scaffold cartilaginous matrix expression and maintain the chondrocyte phenotype in collagen gels Stoddart, M. J. et al. 2009. Cells and biomaterials in cartilage tissue engineering. Regen. Med. 4(1), 28 pp. 81-98 Silk Silk scaffolds provide mechanical properties superior to those of hydrogels They foster the deposition of cartilage in a homogenous and robust fashion They are slowly biodegradable They are biocompatible Silk fibroin are shown to be superior to the collagen-based materials in terms of cartilage-related outcomes. Comparison of the porosity of silk (B), crosslinked collagen (C), and collagen (D) scaffolds Hofmann, S. et al. 2006. Cartilage-like Tissue Engineering Using Silk Scaffolds and Mesenchymal Stem 29 Cells. Tissue Engineering. 12(10), pp. 2729-2738 Synthetic polymeric scaffolds poly(lactic-co-glycolic acid) PLGA Polyethylene glycol Polydioxanone (PDS) 30