BCH 204 ENZYMES MBBS.docx
Document Details
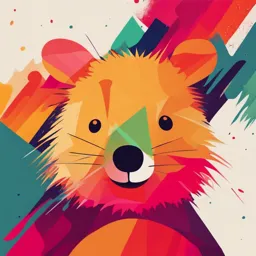
Uploaded by BestPerformingPersonification
Tags
Full Transcript
**DAVID UMAHI FEDERAL UNIVERSITY OF HEALTH SCIENCES, UBURU** **SECOND SEMESTER LECTURE NOTE** **Enzyme kinetics** studies the rates of [enzyme-catalysed](https://en.wikipedia.org/wiki/Enzyme_catalysis) [chemical reactions](https://en.wikipedia.org/wiki/Chemical_reaction). In enzyme kinetics, the [...
**DAVID UMAHI FEDERAL UNIVERSITY OF HEALTH SCIENCES, UBURU** **SECOND SEMESTER LECTURE NOTE** **Enzyme kinetics** studies the rates of [enzyme-catalysed](https://en.wikipedia.org/wiki/Enzyme_catalysis) [chemical reactions](https://en.wikipedia.org/wiki/Chemical_reaction). In enzyme kinetics, the [reaction rate](https://en.wikipedia.org/wiki/Reaction_rate) is measured and the effects of varying the conditions of the reaction are examined. Studying an enzyme\'s [kinetics](https://en.wikipedia.org/wiki/Chemical_kinetics) helps reveal the catalytic mechanism of the enzyme, its role in [metabolism](https://en.wikipedia.org/wiki/Metabolism), how its activity is controlled, and how a [drug](https://en.wikipedia.org/wiki/Drug) or a modifier ([inhibitor](https://en.wikipedia.org/wiki/Enzyme_inhibitor) or [activator](https://en.wikipedia.org/wiki/Enzyme_activator)) might affect the rate. The main properties of enzyme kinetics are how easily the enzyme can be saturated with a substrate, and the maximum rate it can achieve. Knowing these properties suggests what an enzyme might do in the cell and can show how the enzyme will respond to changes in these conditions. An enzyme (E) is typically a [protein](https://en.wikipedia.org/wiki/Protein) [molecule](https://en.wikipedia.org/wiki/Molecule) that promotes a reaction of another molecule, its [substrate](https://en.wikipedia.org/wiki/Substrate_(biochemistry)) (S). This binds to the [active site](https://en.wikipedia.org/wiki/Active_site) of the enzyme to produce an enzyme-substrate complex ES, and is transformed into an enzyme-product complex EP and from there to product P, via a [transition state](https://en.wikipedia.org/wiki/Transition_state) ES\*. The series of steps is known as the [mechanism](https://en.wikipedia.org/wiki/Enzyme_catalysis): This example assumes the simplest case of a reaction with one substrate and one product. Such cases exist: for example, a [mutase](https://en.wikipedia.org/wiki/Mutase) such as [phosphoglucomutase](https://en.wikipedia.org/wiki/Phosphoglucomutase) catalyses the transfer of a phospho group from one position to another, and [isomerase](https://en.wikipedia.org/wiki/Isomerase) is a more general term for an enzyme that catalyses any one-substrate one-product reaction, such as [triosephosphate isomerase](https://en.wikipedia.org/wiki/Triosephosphateisomerase). However, such enzymes are not very common, and are heavily outnumbered by enzymes that catalyse two-substrate two-product reactions: these include, for example, the NAD-dependent [dehydrogenases](https://en.wikipedia.org/wiki/Dehydrogenase) such as [alcohol dehydrogenase](https://en.wikipedia.org/wiki/Alcohol_dehydrogenase), which catalyses the oxidation of ethanol by NAD^+^. Reactions with three or four substrates or products are less common, but they exist. There is no necessity for the number of products to be equal to the number of substrates; for example, [glyceraldehyde 3-phosphate dehydrogenase](https://en.wikipedia.org/wiki/Glyceraldehyde_3-phosphate_dehydrogenase) has three substrates and two products. When enzymes bind multiple substrates, such as [dihydrofolate reductase](https://en.wikipedia.org/wiki/Dihydrofolate_reductase) , enzyme kinetics can also show the sequence in which these substrates bind and the sequence in which products are released. An example of enzymes that bind a single substrate and release multiple products are [proteases](https://en.wikipedia.org/wiki/Protease), which cleave one protein substrate into two polypeptide products. Others join two substrates together, such as [DNA polymerase](https://en.wikipedia.org/wiki/DNA_polymerase) linking a [nucleotide](https://en.wikipedia.org/wiki/Nucleotide) to [DNA](https://en.wikipedia.org/wiki/DNA). Although these mechanisms are often a complex series of steps, there is typically one *rate-determining step* that determines the overall kinetics. This [rate-determining step](https://en.wikipedia.org/wiki/Rate-determining_step) may be a chemical reaction or a [conformational](https://en.wikipedia.org/wiki/Conformational_isomerism) change of the enzyme or substrates, such as those involved in the release of product(s) from the enzyme. Knowledge of the [enzyme\'s structure](https://en.wikipedia.org/wiki/Protein_structure) is helpful in interpreting kinetic data. For example, the structure can suggest how substrates and products bind during catalysis; what changes occur during the reaction; and even the role of particular [amino acid](https://en.wikipedia.org/wiki/Amino_acid) residues in the mechanism. Some enzymes change shape significantly during the mechanism; in such cases, it is helpful to determine the enzyme structure with and without bound substrate analogues that do not undergo the enzymatic reaction. Not all biological catalysts are protein enzymes: [RNA](https://en.wikipedia.org/wiki/RNA)-based catalysts such as [ribozymes](https://en.wikipedia.org/wiki/Ribozymes) and [ribosomes](https://en.wikipedia.org/wiki/Ribosomes) are essential to many cellular functions, such as [RNA splicing](https://en.wikipedia.org/wiki/Splicing_(genetics)) and [translation](https://en.wikipedia.org/wiki/Translation_(biology)). The main difference between ribozymes and enzymes is that RNA catalysts are composed of nucleotides, whereas enzymes are composed of amino acids. Ribozymes also perform a more limited set of reactions, although their [reaction mechanisms](https://en.wikipedia.org/wiki/Reaction_mechanism) and kinetics can be analysed and classified by the same methods **Enzyme assays** [Enzyme assays](https://en.wikipedia.org/wiki/Enzyme_assay) are laboratory procedures that measure the rate of enzyme reactions. Since enzymes are not consumed by the reactions they catalyse, enzyme assays usually follow changes in the concentration of either substrates or products to measure the rate of reaction. There are many methods of measurement. [Spectrophotometric](https://en.wikipedia.org/wiki/Ultraviolet-visible_spectroscopy) assays observe the change in the [absorbance](https://en.wikipedia.org/wiki/Absorbance) of light between products and reactants; radiometric assays involve the incorporation or release of [radioactivity](https://en.wikipedia.org/wiki/Radioactivity) to measure the amount of product made over time. Spectrophotometric assays are most convenient since they allow the rate of the reaction to be measured continuously. Although radiometric assays require the removal and counting of samples (i.e., they are discontinuous assays) they are usually extremely sensitive and can measure very low levels of enzyme activity. An analogous approach is to use [mass spectrometry](https://en.wikipedia.org/wiki/Mass_spectrometry) to monitor the incorporation or release of [stable isotopes](https://en.wikipedia.org/wiki/Stable_isotope) as the substrate is converted into product. Occasionally, an assay fails and approaches are essential to resurrect a failed assay. The most sensitive enzyme assays use [lasers](https://en.wikipedia.org/wiki/Laser) focused through a [microscope](https://en.wikipedia.org/wiki/Microscope) to observe changes in single enzyme molecules as they catalyse their reactions. These measurements either use changes in the [fluorescence](https://en.wikipedia.org/wiki/Fluorescence) of [cofactors](https://en.wikipedia.org/wiki/Cofactor_(biochemistry)) during an enzyme\'s reaction mechanism, or of [fluorescent dyes](https://en.wikipedia.org/wiki/Fluorescent_dyes) added onto specific sites of the [protein](https://en.wikipedia.org/wiki/Protein) to report movements that occur during catalysis. These studies provide a new view of the kinetics and dynamics of single enzymes, as opposed to traditional enzyme kinetics, which observes the average behaviour of populations of millions of enzyme molecules **Allosteric Enzymes** The word *allosteric *stems from two words in Greek: *allos *which means *other, *and *stereos* which means *space.* Together, it reflects the type of regulation in which the binding of a molecule on one site directly impacts the binding of another molecule on another *spatially distinct* site. Allosteric enzymes are a group of regulatory enzymes whose catalytic activities are controlled by noncovalent binding to other molecules called effectors or modulators. Enzymes are regarded as the keys that control cellular activities. A single cellular task is accomplished through a series of interconnected biochemical reactions in the metabolic pathway. Each reaction must take place in sequence and be catalyzed by a specific enzyme that only acts upon its substrate. The resulting product from the catalyzed reaction typically acts as the substrate of the next reaction. Thus, the abundance and the activity of the enzymes in the corresponding pathway influence the metabolic flux,* *or the turnover rate of the metabolites, which, in turn, affect the overall cellular activities. The overall rate of the entire metabolic pathway is governed by one chemical reaction of the pathway called the rate-limiting reaction.* *Also known as the rate-determining step,* *this metabolic reaction proceeds at the slowest rate and is regulated through the activity of a regulatory enzyme, whose catalytic activity relies on its interaction with a smaller signal molecule. The interaction can occur in the form of covalent modification* *or in non-covalent interaction **Characteristics** 1\. Allosteric enzymes are multi-subunit and possess a catalytic and regulatory site Allosteric enzymes are generally multi-subunit proteins, consisting of one subunit that performs a *catalytic* function and at least, another subunit that performs a *regulatory *function. The *catalytic *subunit of an allosteric enzyme is similar to other typical enzymes: it contains an *active site* that binds to the complementary substrate to initiate the enzyme's catalytic activity. The *regulatory* subunit comprises a *regulatory *site*, *also referred to as an *allosteric* site, which binds to an effector molecule to influence the activity of the catalytic subunit. 2\. Allosteric enzyme activities are regulated by the binding to its regulatory site The binding of the effector to the regulatory site transmits messages to the catalytic subunit in the form of conformational changes, to modify conformational changes of the active site, thereby influencing the catalytic activity of the enzyme. The binding of effectors to the regulatory site to the catalytic activity can be heterotrophic or *homotropic.* If the effector is a small [*ligand*](https://conductscience.com/enzymes-as-biocatalysts/) molecule, and its binding to the regulatory site on an allosteric enzyme affects the binding on the catalytic subunit of the substrate or *another *ligand, the enzyme is said to exhibit a *heterotropic *interaction. Conversely, *homotropic *interaction refers to when the binding of its substrate or ligand on one subunit of an allosteric enzyme affects the binding affinity of the *same substrate* on *another *subunit of the enzyme. The binding of the effector to the allosteric site can trigger *positive cooperativity *when the binding increases the affinity between the successive binding of the substrate and the active site. Effector-regulatory site binding can also invoke *negative* *cooperativity *if the binding activity of the enzyme that follows is impeded. 3\. The kinetics of allosteric enzymes fits a sigmoid growth curve The kinetics of most enzymes follow the hyperbolic curve of the Michaelis-Menten Equation. At the initial stage of an enzymatic reaction, the relationship between the reaction turnover rate and the substrate concentration is linear, given a constant enzyme concentration the rate of the reaction increases as the substrate concentration increases. **Examples of allosteric enzymes that regulate notable metabolic pathways** ***Phosphofructokinase* *(PFK)**** *is the key regulatory enzyme in glycolysis, the cellular production of energy from the breakdown of carbohydrate molecules. Glycolysis results in the production of pyruvate and the generation of high-energy molecules, adenosine triphosphate (ATP). The enzyme PFK possesses two active sites, which bind to its substrates, fructose-6-phosphate (F6P) and ATP. It also contains another regulatory site that binds to ATP or adenosine monophosphate (AMP). ATP acts as a negative effector and AMP as a positive effector, and both regulate PFK enzymatic activity in relation to the availability of cellular energy. When energy is depleted, glycolysis is stimulated through the binding of AMP, which is more abundant than ATP, to the regulatory site. AMP binding increases the PFK catalytic activity so that glucose is metabolized and ATPs are produced. In contrast, when cellular energy is sufficient, ATP concentration is high. Instead of AMP, surplus ATP molecules bind to the regulatory site, resulting in the reduction in the enzyme affinity to its substrate, F6F, temporarily inhibiting glycolysis. ***Isocitrate dehydrogenase (IDH) ***catalyzes the primary control point of the citric acid cycle. Also known as the *Krebs cycle *or the *tricarboxylic acid *(TCA) cycle, it is the central pathway in aerobic metabolism, which yields energy from the degradation of acetyl CoA and provides building blocks for the biosynthesis of amino acids, heme, and nucleic acids. Human IDH exists as two sets of heterodimers, one acting as a catalytic subunit and the other as the regulatory subunit. Under normal circumstances, citric acid or TCA, the product of the first reaction of the cycle, binds to the allosteric site on the regulatory subunit, causing the alteration in the subunit conformation. This change in the conformation of the regulatory subunit is relayed to the catalytic subunit to induce the binding of the substrate, isocitrate, to the active site and initiate the enzyme catalytic activity. Under diminishing cellular energy, the cellular concentration of adenosine diphosphate (ADP) is high, and the rate of the overall cycle is enhanced through the binding of ADP and Mg^2+^ to the allosteric site, adjacent to where TCA is bound. ADP-binding does not induce conformational change per se, but the catalytic activity of the enzyme is further intensified through the stabilization of the substrate-binding. ***Aspartate transcarbamoylase (ATCase) ***is the enzyme that catalyzes the rate-limiting and committed step of pyrimidine biosynthesis. The pathway produces pyrimidine, which is a component of nucleic acids. ATCase consists of a large catalytic subunit and a smaller regulatory subunit. The regulatory subunit binds to the final product of the pathway, cytidine triphosphate (CTP) when it reaches sufficient cellular concentration and inhibits ATCase catalytic activity. The binding of the pathway product to the regulatory enzyme serves as a *feedback*mechanism, which signals a pause in the activity of the pathway.