Biological Chemistry 1A Metabolism Notes (Autumn 2024) PDF
Document Details
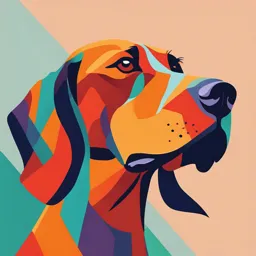
Uploaded by PraiseworthyOganesson4789
University of Edinburgh
2024
Peter Kirsop
Tags
Related
- Lecture 22: Introduction to Biochemistry (Year 1 2021 MIBS 65101) PDF
- Lecture Notes: Introduction to Metabolism and Glycolysis PDF
- Advanced Biochemistry Lecture Notes PDF
- Carbohydrates Chemistry & Metabolism Part 1 Lecture Notes 2023-2024 PDF
- CHEM2770 Lecture #1 - Introduction to Biochemistry PDF
- FTEC-50c Lecture Notes PDF
Summary
These are lecture notes for the Biological Chemistry 1A course on Metabolism, covering topics such as the introduction to metabolism, definitions of catabolism and anabolism, driving metabolism, thermodynamics, and different pathways like Glycolysis. The document has notes from Autumn 2024 semester by Dr. Peter Kirsop of the University of Edinburgh .
Full Transcript
Biological Chemistry 1A Metabolism Dr. Peter Kirsop [email protected] 6 Lectures, Autumn 2024 Books In addition to the BC1 coursebook you can, if you wish, consult the following in the library: Biochemistry 7th Edition, J. M. Berg, J. L. Tymoczko & L. Stryer:...
Biological Chemistry 1A Metabolism Dr. Peter Kirsop [email protected] 6 Lectures, Autumn 2024 Books In addition to the BC1 coursebook you can, if you wish, consult the following in the library: Biochemistry 7th Edition, J. M. Berg, J. L. Tymoczko & L. Stryer: – Chapter 15,16 and 17 Introduction Metabolism is the process by which biological molecules are broken down and re-synthesised complex (>1000 chemical reactions even in E. coli) network of enzymatic reactions highly regulated metabolic imbalances can lead to ill-health and disease Metabolic cycle Introduction Food Energy Energy Building blocks DNA, protein, membrane Waste (urea, NH3, H2O, CO2) Introduction Metabolic diseases such as diabetes mellitus, metabolic syndrome and obesity are major and growing public health problems. Diabetes affected 422 million worldwide in 2014. In 2019, diabetes was the direct cause of 1.5 million deaths and 48% of all deaths due to diabetes occurred before the age of 70 years.1 Diabetes is a leading cause of blindness (24,000 new cases / yr and highest users of dialysis, need for organ transplantation and increased heart disease. Obesity defined as body mass index (BMI) ≥30kg/m2 is present in 25% of UK population. 1 World Health Organisation. Fact sheets Diabetes. https://www.who.int/news-room/fact-sheets/detail/diabetes Introduction A better understanding of fatty acid metabolism will help in the control of general health and the curing of disease Different organs – different metabolism Eukaryote metabolic pathways, so far. Definitions Catabolism. Catabolic processes are the breaking down of relatively complex molecules to simpler ones. This is to either salvage components or to generate energy Anabolism. Anabolic processes involve the net use of energy to build more complex molecules from simpler ones. Metabolism = catabolism + anabolism catabolism Carbohydrate, fats CO2 + H2O + useful energy Useful energy + simple precursors anabolism complex molecules Definitions Cofactor. A substance, such as an ion or coenzyme, which must be associated with an enzyme it for it to function Coenzyme. A non-protein organic substance, which combines with a specific protein, an apoenzyme, to form an active enzyme. Apoenzyme. The protein component of an enzyme, to which the coenzyme attaches to form an active enzyme. Driving metabolism catabolism Carbohydrate, fats CO2 + H2O + useful energy Useful energy + simple precursors anabolism complex molecules The overall metabolic process must be thermodynamically favourable to occur, and this will be when the overall free energy, ΔG, is negative. A reaction can occur spontaneously if ΔG is negative A system is at equilibrium and no net change can take place if ΔG is zero An input of free energy is required to drive a reaction if ΔG is positive Driving metabolism - thermodynamics Free-energy difference ΔG between the products and reactants of a reaction – this determines spontaneity ΔG also shows the energy required to initiate the conversion of reactants to products The free-energy change provides information about the spontaneity of a reaction but not the rate of a reaction 3 conditions to consider: A reaction can occur spontaneously if ΔG is negative A system is at equilibrium and no net change can take place if ΔG is zero An input of free energy is required to drive a reaction if ΔG is positive Driving metabolism - thermodynamics The overall free-energy change for a chemically coupled series of reactions is equal to the sum of the free-energy changes of the individual steps A B+C DGo’ = +21 kJmol-1 B D DGo’ = -34 kJmol-1 A C+D DGo’ = -13 kJmol-1 Thus, a thermodynamically unfavourable reaction sequence can be converted into a favourable one by coupling it to another spontaneous reaction e.g. the hydrolysis of a sufficient number of ATP molecules Driving metabolism - thermodynamics For example Sugars and fatty acids are sources of energy Complete oxidation of a fuel such as glucose C6H12O6 + 6 O2 6 CO2 + 6 H2O Releases lots of energy (ΔGo’ = -2850 kJmol-1) Complete oxidation of palmitate, a C16 fatty acid C16H32O2 + 23 O2 16 CO2 + 16 H2O Releases a lot more energy (ΔGo’ = -9781 kJmol-1) Driving metabolism - ATP Adenosine triphosphate (ATP) ATP hydrolysis drives many reactions Intracellular [ATP] controlled at 2-10 mM Driving metabolism - ATP Important features of ATP: resonance stabilisation electrostatic repulsion metal binding (Mg2+) Glycolysis Glycolysis is the sequence of reactions that metabolises glucose to pyruvate with the accompanying production of energy. Overall, glycolysis converts glucose, a six carbon molecule, into two three carbon units and two molecules of adenosine triphosphate, ATP. In humans, glucose in the blood enters most cells by a specific transporter and is metabolised in the cytosol which is part of the cytoplasm. Carbohydrates - monosaccharides Monosaccharides may be classified as either aldoses or ketoses. The -ose part indicates it is a carbohydrate. The ald- prefix indicates that the carbonyl is present as an aldehyde and the ket- prefix indicates that the carbonyl is present as a ketone. CHO H OH HO H H OH H OH CH2OH ribose glucose fructose an aldopentose an aldohexose a ketohexose Carbohydrates - polysaccharides Polysaccharides are formed by the same type of chemistry that causes glucose to cyclise – loss of water. OH OH O O H O HO HO OH HO OH OH OH H H OH OH O HO O HO O OH HO OH H OH H This is a disaccharide as it is formed from two monomer units. Carbohydrates - polysaccharides Large numbers of monomer units may polymerise in the same way. This is cellulose, formed from glucose, with 1,4 β linkages: This is amylose, also formed from glucose, but with 1,4 α linkages: Mammals can hydrolise α linkages but not β linkages. Glycolysis - glucose Glucose interconverts between alpha and beta form by mutorotation which is reversible ring opening of each anomer to the open chain aldehyde form. 37.3% 0.01% 62.6% Glycolysis - glucose Glucose interconverts between alpha and beta form by mutorotation which is reversible ring opening of each anomer to the open chain aldehyde form. OH anomeric carbon O HO H+ HO OH OH OH glucose - pyranose form OH HO HO OH OH O H+ O HO HO OH OH glucose - pyranose form anomeric carbon Glycolysis – overall process C6H12O6 + 2NAD+ + 2ADP + 2P 2CH3(CO)COO- + 2ATP + 2NADH + 2H+ Overall 10 reactions in 3 stages Two reactions involve energy investment of ATP. Three reactions pay back the cost in the form of NADH and ATP. Glycolysis – steps Sources of Glucose Most of the sugar we consume is sucrose, a disaccharide. Sucrose is two monosaccharides, 6 membered ring glucose and 5 membered ring fructose. The average person consumes about 24 kilograms of sugar each year, or 33.1 kilograms in developed countries, equivalent to over 260 food calories per day. Sources of Glucose Enzymes in the mouth partially break down sucrose into glucose and fructose, and acid in the stomach breaks it down further. The majority of sugar digestion happens in the small intestine catalysed by the enzyme sucrase. Glycolysis – step 1 Phosphorylation of glucose by ATP to give glucose-6-phosphate catalysed by Hexokinase 6 CH2OH 6 CH OPO 2− 2 3 ATP ADP 5 O 5 O H H H H H H 4 1 4 H 1 OH H 2+ OH Mg OH OH OH OH 3 2 3 2 H OH Hexokinase H OH glucose glucose-6-phosphate glucose + ATP glucose-6-phosphate + ADP + H+ Glycolysis – step 1 Nucleophilic attack of an alcohol OH on an anhydride Need a base to deprotonate the OH to make it more like –O- Product is resonance stabilised Glycolysis – step 1 Energetically - we can break this down into 2 coupled steps, phosphorylation of glucose and hydrolysis of ATP: DGo’ (kJmol-1) Glucose + Pi Glucose-6-P + H2O +13.8 ATP + H2O ADP + Pi -30.5 Glucose + ATP G-6-P + ADP -16.7 Overall DG –ve so equilibrium lies in favour of products Glycolysis – step 1 DGo’ (kJmol-1) Glucose + Pi Glucose-6-P + H2O +13.8 ATP + H2O ADP + Pi -30.5 Glucose + ATP G-6-P + ADP -16.7 This reaction is catalysed by a hexokinase enzyme which binds the glucose in a water-free environment for reaction to take place with ATP. This is by an induced fit mechanism Glycolysis – step 1 Induced fit: Enzyme-substrate interactions. Binding of glucose to Hexokinase induces a large conformational change. CH2OH glucose OH O MgATP OH HO OH hexokinase CH2OH O OH OH HO OH open glucose-bound low affinity for MgATP binds MgATP The enzyme active site is at an interface between protein domains connected by a flexible hinge region. This allows access to the active site, while positioning active site residues, and for this step exclusion of water, following a substrate-induced conformational change. Glycolysis – step 1 Structure of a human hexokinase - a dimer with glucose and G-6P bound The substrates sit in a deep, water- free cleft to avoid ATP hydrolysis in the absence of glucose In addition, the glucose-6-phosphate produced in Step 1 inhibits this hexokinase so keeping the rate of production under control. Glycolysis – step 1 The hexokinase has an aspartic acid residue at the active site which acts as a base to deprotonate the – OH of glucose Glycolysis – step 2 Glycolysis – step 2 Transformation of Glucose-6-phosphate to Fructose-6-phosphate catalysed by PhosphoGlucose Isomerase (PGI) 6 CH OPO 2− 2 3 5 6 CH OPO 2− 1CH 2OH H O H 2 3 O H 4 H 1 5 H HO 2 OH OH OH H 4 3 OH 3 2 OH H H OH Phosphoglucose Isomerase glucose-6-phosphate fructose-6-phosphate This an isomerisation reaction converting a pyranose to a furanose Glycolysis – step 2 This is the converting of a 6-membered sugar to a 5-membered sugar O H 2- C CH2OPO3 O CH2OH 2- CH2OH O H OH O3POH2C O HO H HO H OH HO OH H OH H OH HO H OH OH OH H OH HO CH2OPO32- CH2OPO32- Ring opening Ring closure (Hemi-acetal hydrolysis) (Hemi-ketal formation) isomerisation Isomerisation catalysed by acid-base chemistry using active site residues on enzyme surface - no cofactor involved. Glycolysis – step 2 Glycolysis – step 2 Mechanism of isomerisation keto-form enol-form Here it happens twice an ‘enediol’ is the intermediate Glycolysis – step 2 Acids and bases at the active site of PGI enzyme catalyse the reaction Glutamic acid (Glu), Histidine (His) and Lysine (Lys) act together as a H+ shuttle/relay. “A catalytic triad” of residues Glycolysis – step 3 Conversion of fructose-6-phosphate to fructose-1,6-bisphosphate catalysed by PhosphoFructoKinase (PFK) Phosphofructokinase PFK 6 CH OPO 2− 1CH2OH 6 CH OPO 2− 1CH2OPO32− 2 3 2 3 O ATP ADP O 5 H HO 2 5 H HO 2 H 4 3 OH Mg2+ H 4 3 OH OH H OH H fructose-6-phosphate fructose-1,6-bisphosphate Mechanism O- O- O- - O P O P O P O Ad 2- CH2OH O O O O3POH2C O 2- O3POH2C CH2OPO32- O HO HO + ADP + H+ OH OH HO HO Glycolysis – step 3 Allosteric regulation (or allosteric control) is the regulation of a protein by binding an effector molecule at a site other than the enzyme's active site. The site to which the effector binds is termed the allosteric site. Glycolysis – step 3 The active site binds to the sugar, shown in orange, and an ATP, shown in red The enzyme also has regulatory binding sites at the top and bottom--the other ADP molecules bind (*) ATP/ADP binding to regulatory site – causes conformational change – turns enzyme off Glycolysis – overview of first three steps CH2OH O The Embden-Meyerhof Pathway OH HO OH HK OH CH2OPO32- O glucose OH HO OH OH PGI G-6-P 2- O3POH2C O CH2OH HO HO OH PFK F-6-P 2- O3POH2C CH2OPO32- O HO Overall, 2 ATP 2ADP + 2H+ OH HO F-1,6-BP Glycolysis – step 4 Dihydroxyacetone phosphate (DHAP) 3 CH2OPO32- 1 CH2OPO32- 2- O3POH2C CH2OPO32- 2 O 2 C O O HO 3 H HO CH2 HO H 4 OH 1 OH H 5 OH HO CH2OPO32- H O 6 1 C Fructose 1,6 Bisphosphate F-1,6-BP H 2C OH (F-1,6-BP) open form CH2OPO32- closed form 3 Glyceraldehyde-3- phosphate (GAP) This reaction is catalysed by the enzyme aldolase Glycolysis – step 4 This is a retro aldol reaction, which is a reverse of an aldol reaction R R R R H2O -OH R -OH C O H2O C O C O C O- C O CH2 CH2 CH2 CH2 CH3 H C OH - H C O + enolate ketone R' R' H O C aldehyde R' Aldol: Glycolysis – step 4 This is a retro aldol reaction, which is a reverse of an aldol reaction R R R R H2O -OH R -OH C O H2O C O C O C O- C O CH2 CH2 CH2 CH2 CH3 H C OH - H C O + enolate ketone R' R' H O C aldehyde R' This is a base catalysed mechanism, the aldolase is the source of the base. Glycolysis – step 4 Uses a “Schiff base” mechanism and is160kDa tetrameric (~40kDa monomers). tetramer Type I monomer Schiff’s base named after Hugo Schiff (Imine/Iminium ion chemistry) Glycolysis – step 5 At the end of stage 4 have 2 x 3 carbon units – DHAP and GAP. Only GAP is further metabolised by step 6 in the glycolytic pathway. That would mean that half the sugar would be “wasted” as a dead-end metabolite DHAP To deal with this, an enzyme converts DHAP into GAP called Triose Phosphate Isomerase (TIM or TPI) Glycolysis – step 5 TIMs are found in many organisms and are active as dimers. Amino acid sequence alignment showed 2 residues involved in the mechanism of the reaction, Histidine (His95) and Glutamic acid (Glu165). A protein loop closes on the DHAP TIM Mechanism Enediol intermediate Acid/base chemistry of TIM Glycolysis – step 5 TIM catalysed DHAP to GAP conversion is very fast, close to the diffusion-controlled limit. TIM is an example of a kinetically “perfect” enzyme. It accelerates isomerisation by a factor of 108 compared with a simple base catalysed reaction. Also, TIM suppresses an undesired side reaction, the decomposition of the enediol intermediate into methyl glyoxal and orthophosphate HO OH O O H CH2 OPO32- H CH3 PO42- enediol methyl intermediate glyoxal In solution, this physiologically useless reaction is 100 times faster than the isomerisation reaction. Glycolysis – step 5 TIM closes around the enediol: TIM prevents the enediol from leaving the enzyme active site – a loop/lid closes to prevent escape. Glycolysis – so far….. The preceding five steps in glycolysis have transformed 1 x glucose into two molecules of GAP, but 2 x ATP molecules have been used The second five steps harvest energy. NAD NAD = Nicotinamide adenine dinucleotide NAD is a coenzyme dinucleotide involved in redox reactions, carrying electrons from one reaction to another. The coenzyme NAD+ was first discovered by Arthur Harden in 1906. He noticed that adding boiled and filtered yeast extract greatly accelerated alcoholic fermentation in unboiled yeast extracts. Glycolysis – overall The Glycolytic pathway CH2OH O O O x2 OH -O OH HO CH3 OH C6H12O6 C 3H 3O3 glucose pyruvate Overall 10 reactions in 3 Stages 2 involve energy investment of ATP 3 pay back the cost in the form of NADH and ATP Glycolysis – overall Free energy change in glucose to pyruvate conversion The breakdown of glucose into pyruvate during glycolysis releases a large amount of energy which is captured for other metabolic processes through coupled reactions. Glycolysis – regulation The four regulatory enzymes are hexokinase (step 1), phosphofructokinase (step 2), and pyruvate kinase (step 10) These control the rate of glycolysis to regulate glucose levels to prevent: Hyperglycemia – glucose levels too high Hypoglycemia – glucose levels to low. Glycolysis – regulation Insulin produced in the pancreas is the hormone that controls the rate of metabolism of glucose Pyruvate – where it goes Further metabolism of pyruvate allows the redox balance to be maintained – regeneration of NAD+ from NADH Pyruvate – where it goes Anaerobic conditions Aerobic conditions Yeast and bacteria Pyruvate – where it goes Anaerobic conditions Pyruvate – anaerobic conditions Catalysed by Lactate Dehydrogenase (LDH). Lactate is produced under anaerobic conditions, for example in muscle during intense exercise. Overall, anaerobic glycolysis in muscle can be shown as: Glucose + 2Pi + 2ADP---------> 2 Lactate + 2ATP + 2H2O + 2H+ Lactate is converted to glucose in the liver by gluconeogenesis. Gluconeogenesis Glucose synthesis from pyruvate during times of starvation Gluconeogenesis is not simply a reverse of glycolysis. 7 steps use the same enzymes but 3 steps are different Gluconeogenesis Gluconeogenesis and glycolysis can occur in different organs and are linked by the Cori Cycle via the bloodstream Pyruvate – where it goes Yeast and bacteria Pyruvate – ethanol in humans The average human digestive system produces approximately 3 g of ethanol per day through fermentation of its contents. This, and any ethanol consumed, is catabolysed by: 1. Conversion to acetaldehyde 2. Acetaldehyde to acetic acid 3. Acetic acid to acetyl CoA which then enters the Krebs cycle In 2014, it was estimated that 4% of deaths worldwide were attributable to alcohol use. Pyruvate – ethanol production in yeast First, a decarboxylation reaction catalysed by pyruvate decarboxylase, which requires a coenzyme derived from vitamin B1 The second step is a reduction reaction catalysed by alcohol dehydrogenase and is zinc-dependant. The conversion of glucose into ethanol is alcoholic fermentation, very important commercially. Ethanol production from fermentation Ethanol production by fermentation accounts for 80% of world production at around 13 million tonnes p.a. The other 20% is produced synthetically from ethene. Fermentation may also be used to produce fuel from waste biomass Ethanol production from fermentation Problems with Fuel from Waste Biomass 1. Large amounts of biomass required – needs very large vessels. 2. Production time scale is long. Takes 48 hours to produce 9% ethanol in water, and process maximum is 14% ethanol. 3. Separation costs of ethanol from media is high 4. Large amounts of waste are produced. Despite this the process is feasible in some countries, and as crude oil costs rise will become more important. Ethanol may be produced from waste plant material and used in “gasohol” manufacture. Other compounds from fermentation Micro-organisms are used in the chemical industry to produce fine chemicals and pharmaceutical products. An example of this is the production of citric acid for food use. More than 1,600,000 tonnes per annum are consumed world-wide. CO2H Asp. niger Molasses (sugar syrup) HO CO2H CO2H citric acid An example of large-scale pharmaceutical production is the synthesis of penicillin by penicillium chrysogenum. More than 12 million kilograms are produced annually, usually by batch fermentation. Penicillin G Penicillin V Other compounds from fermentation If we had to synthesise it in the lab: Other compounds from fermentation Another couple of examples: Produced by cells of Genetically modified E. Coli methanobacter sp., used to make a heat stable mutant grown on methanol. of chymotripsin for washing powders This area is growing rapidly and now fermentation technology is an important field in biological chemistry Pyruvate – where it goes Aerobic conditions Pyruvate – aerobic conditions Conversion to acetyl-CoA and entry to the citric acid cycle a.k.a Kreb’s cycle, tricarboxylic acid (TCA) cycle A large amount of energy can be extracted aerobically by means of the citric acid cycle and the electron–transport chain The reactions of the citric acid cycle occur inside mitochondria, in contrast with those of glycolysis, which take place in the cytosol Hans A Krebs discovered the pathway in 1937 – Awarded Nobel Prize for Medicine in 1953 The Krebs cycle will be covered in next year’s course Biological Chemistry 2. The End. Melvin