Lipid - B100102T BS104 Unit 3 (2) PDF
Document Details
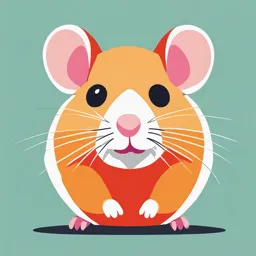
Uploaded by TimelyField6938
Integral University
Tags
Summary
This document provides an introduction to lipids, covering their structure, classification, and properties. It discusses simple lipids, such as fats and waxes, and also delves into saturated and unsaturated fatty acids.
Full Transcript
Lipid Lipids are a heterogeneous group of organic compounds that are insoluble in water and soluble in non-polar organic solvents. They naturally occur in most plants, animals, microorganisms and are used as cell membrane components, energy storage molecules, insulation, and hormones. Lipids are...
Lipid Lipids are a heterogeneous group of organic compounds that are insoluble in water and soluble in non-polar organic solvents. They naturally occur in most plants, animals, microorganisms and are used as cell membrane components, energy storage molecules, insulation, and hormones. Lipids are a broad group of organic compounds which include fats, waxes, sterols, fat-soluble vitamins, monoglycerides, diglycerides, phospholipids, and others. Lipids are organic compounds that contain hydrogen, carbon, and oxygen atoms, which form the framework for the structure and function of living cells. Classification Of Lipids Lipids are generally classified into three main classes 1. Simple Lipids 2. Compound Lipids 3. Derived Lipids 1. Simple Lipids Simple lipids are the esters of fatty acids with various alcohols. These can be further categorized into fats and waxes. Fats are tri-esters of glycerol and fatty acids. Fats Fats are solids at room temperature. Chemically, fats are triglycerides since, three molecules of fatty acids condense with one molecule of glycerol e.g. three molecules of stearic acid are linked to glycerol to yield glyceryl tri-stearate, a fat. Fats which are liquid at room temperature are called oils. Oils are also esters of fatty acids and glycerol, but the fatty acids found in oils are unsaturated fatty acids. Waxes Waxes are another class of simple lipids. These are the esters of fatty acids with high molecular weight alcohol. Waxes contain one molecule of fatty acids and one molecule of alcohol. The bees wax, the fatty acid constituent is a smaller chain acid, palmitic acid and [16ºC] and alcohol is myristic palmitate. Fatty acids Fatty acids are obtained from the hydrolysis of fats. Fatty acids are organic molecules that are long-chained carboxylic acids with 4-36 carbon atoms. Fatty acids are not found in the free state but remain associated with alcohol to form triglycerides. Fatty acids are stored as an energy reserve (fat) through an ester linkage to glycerol to form triglycerides. The acids which are combined with glycerol in the naturally occurring fats are called fatty acids. These acids contain even number of carbon atoms linked together in long chains which are in general un-branched. On the basis of presence or absence of double bonds, fatty acids may be classified into two main classes- A. Saturated fatty acids (saturated with hydrogen) B. Unsaturated fatty acids A. Saturated fatty acids The simplest fatty acids are unbranched, linear chains of CH2 groups linked by carbon-carbon single bonds with one terminal carboxylic acid group. The term saturated indicates that the maximum possible number of hydrogen atoms is bonded to each carbon in the molecule. Many saturated fatty acids have a trivial or common name as well as a chemically descriptive systematic name. The systematic names are based on numbering the carbon atoms, beginning with the acidic carbon. The table gives the names and typical biological sources of the most common saturated fatty acids. Although the chains are usually between 12 and 24 carbons long, several shorter-chain fatty acids are biochemically important. For instance, butyric acid (C4) and caproic acid (C6) are lipids found in milk. Palm kernel oil, an important dietary source of fat in certain areas of the world, is rich in fatty acids that contain 8 and 10 carbons (C8 and C10). B. Unsaturated fatty acids Unsaturated fatty acids have one or more carbon-carbon double bonds. The term unsaturated indicates that fewer than the maximum possible numbers of hydrogen atoms are bonded to each carbon in the molecule. The number of double bonds is indicated by the generic name— monounsaturated for molecules with one double bond or polyunsaturated for molecules with two or more double bonds. Oleic acid is an example of a monounsaturated fatty acid. Common representative monounsaturated fatty acids together with their names and typical sources are listed in the table. The prefix cis-9 in the systematic name of palmitoleic acid denotes that the position of the double bond is between carbons 9 and 10. Two possible conformations, cis and trans, can be taken by the two CH2 groups immediately adjacent to the double-bonded carbons. In the cis configuration, the one occurring in all biological unsaturated fatty acids, the two adjacent carbons lie on the same side of the double-bonded carbons. In the trans configuration, the two adjacent carbons lie on opposite sides of the double-bonded carbons. Polyunsaturated fatty acids:-Having more than one double bonds e.g. linoliec acid, eleostearic acid etc. In most of the monosaturated fatty acids there is a single bond lying between carbon atoms 9 and 10. This is designated as ∆9. The symbol ∆ with the superscript number nine (9) indicates the positions of the double bond. When there are more than one double bond (polyunsaturated fatty acids), the additional bonds occur between the ∆9 double bond and the methyl terminal end of the chain. The symbol 18:3 signifies that there are three double bonds and symbol ∆ 9,12,15 signifies that the position of double bonds are between carbon atom 9 and 10, 12 and 13 and 15 and 16. Presences of double bonds in the fatty acids lower their melting point considerably. Most plant fats contain unsaturated fatty acids as oleic acid and linoleic acid and hence they are liquid at room temperature. Contrary to this animal fats have more of saturated fatty acids and hence solid at room temperature. Essential and Non Essential Fatty Acids Essential fatty acids: The fatty acids which cannot be synthesized by human body but are essential for the normal maintenance of the body are called essential fatty acids.These fatty acids must be included in our diet. Three polyunsaturated fatty acids, linoleic acid, linolenic acid and arachidonic acid are the essential fatty acids. Non-essential fatty acid: These are the fatty acids which can be synthesized by our body. Thus they need not be included in our diet. They are unsaturated fatty acids and are synthesized from their corresponding saturated fatty acids by introducing a single bond.e.g. palmitoleic acid and oleic acid. 2. Compound Lipids Compound lipids contain some additional groups or elements besides fatty acids are alcohol. The addition group may contain phosphorus, nitrogen, sulphur or it may be a protein. Compound lipids can addition group may contain phosphorus, nitrogen, sulphur or it may be a protein. Compound lipids can be categorized into the following: Phospholipids, Glycolipids, other glycolipids. Phospholipids Phospholipids are those compound lipids which contain a phosphorus atom. Phospholipids are wide spread in bacteria, animal and plant tissues and their general structures are quiet similar. These have been termed as amphipathic and compound since they process both polar and non- polar function. In addition to phosphorus, phospholipids may also contain nitrogen as a key component. There are various types of phospholipids including lecithin, cephalins, plasmalogens, phosphoinositides and phosphosphingosides. 3. Derived lipids Derived lipids are the product of hydrolysis of simple lipids and compound lipids and in addition other lipid like compounds such as steroids, terpenes, fatty acids, alcohols, carotenoids, essential oils etc. Steroids: Steroids are the derivatives of cyclo-pentano-perhydrophenanthrene, a compound consisting of four fused non-planer rings. They are named as A, B, C and D. The rings A, B and C are hexagons and are called cyclohexane rings while D is a pentagon and is called cyclopentane. Properties of Fatty Acid Physical Properties 1. Fats and fatty acids are soluble in organic solvents, such as petroleum ether, benzene and chloroform. They are insoluble in water. 2. Saturated fatty acids are solid at room temperature, while unsaturated fatty acids are liquid. 3. Unsaturated fatty acids show cis-trans isomerism due to presence of double bonds. 4. They are bad conductors of heat. 5. Saturated glycerides containing fats require high temperature for melting, whereas unsaturated glycerides containing fats require relatively lower temperature for its melting. Chemical Properties 1. Hydrolysis: Fats undergo hydrolysis when treated with mineral acids, the alkalies or fat splitting enzyme lipase or hydrolases to yield glycerol and the constituent fatty acids. Hydrolysis by alkalies, such as NaOH or KOH leads to the formation of sodium or potassium salts of fatty acids. The salts are known as soaps and process of its formation is saponification. 2. Hydrogenation: Oils containing unsaturated fatty acids can be hydrogenated in presence of high temperature, pressure and finely divided nickel. By this process the oils are converted into solid fats (glycerides of saturated fatty acids). This reaction forms the basis of the industrial production of hydrogenated oil (vegetable ghee). 3. Hydrogenolysis: Oils and fats are converted into glycerol and a long chain aliphatic alcohol when excess of hydrogen is passed through them under pressure and in presence of copper-chromium catalyst. This splitting of fat by hydrogen is called hydrogenolysis. 4. Halogenation: When unsaturated fatty acids are treated with halogens, such as iodine and chlorine, they take up iodine or other halogens at their double bond site. This process of taking of iodine is called halogenation which is an indication of unsaturation. Iodine number is the percentage of iodine absorbed by a fat. 5. Rancidity: Oils and fats on long storage in contact with heat, light, air and moisture, develop an unpleasant odor. Such oils and fats are known as rancid oils and fats. The rancidity develops due to certain chemical changes taking place in the fat. Hydrolysis of fats is caused by enzyme lipase which is produced by microorganisms present in them. To check the rancidity, it becomes essential to protect oils and fats from air, light and moisture during storage. 6. Emulsification: The process of breaking of large-sized fat molecules into smaller ones is called emulsification. In animals, this process is brought about by bile juice liberated from liver. Other emulsifying agents are water, soaps, proteins and gums. Function of Fatty Acids Biological Signalling Fatty acids are involved in a wide range of biological signaling pathways. Following the dietary intake of polyunsaturated lipids, the products of lipid peroxidation can function as the precursors of powerful signaling mediators. Some examples of such signaling include eicosanoid production, the peroxidation of LDL, and the modulation of metabolic and neurological pathways. The Metabolism of Fatty Acids as a Fuel Source The metabolism of fatty acids involves the uptake of free fatty acids by cells via fatty acid- binding proteins which transport the fatty acids intracellularly from the plasma membrane. The free fatty acids are then activated via acyl-CoA and transported to: 1) the mitochondria or peroxisomes to be converted into ATP and heat as a form of energy; 2) facilitate gene expression via binding to transcription factors; or 3) the endoplasmic reticulum for esterification into various classes of lipids that can be used as energy storage. Energy Storage Fatty acids are also used as a form of energy storage as fat droplets composed of hydrophobic triacylglycerol within specialized fat cells called adipocytes. When stored in this form, fatty acids are important sources of thermal and electrical insulation, as well as protection against mechanical compression. Fatty acids are the preferred form of energy storage over glucose because they produce approximately six times the amount of usable energy. Cell Membrane Formation In particular, cell membranes are composed of a phospholipid bilayer made up of two fatty acid chains bound to glycerol and a hydrophilic phosphate group joined to a smaller hydrophilic compound (e.g., choline). Thus, each phospholipid molecule has a hydrophobic tail composed of two fatty acid chains and a hydrophilic head comprised of the phosphate group. Cellular membranes are formed when two phospholipid monolayers associate with the tails joining in an aqueous solution to create a phospholipid bilayer. Protein Modification Fatty acids play several critical roles via their interaction with various proteins. The acylation of proteins is an important function of polyunsaturated fatty acids, as it is critical for the anchoring, folding, and function of multiple proteins. In addition, fatty acids can also interact with various nuclear receptor proteins and promote gene expression, as several fatty acid-protein complexes function as transcription factors. In this manner, fatty acids have been found to regulate the transcription of genes related to metabolism, cellular proliferation, and apoptosis. Degradation of Fatty Acids Beta (β) oxidation of Fatty Acid Beta-oxidation is the catabolic process by which fatty acid molecules are broken down in the cytosol in prokaryotes and in the mitochondria in eukaryotes to generate acetyl-CoA. Beta oxidation process involves multiple steps by which fatty acid molecules are broken down to produce energy. Location of Beta-Oxidation Beta-Oxidation takes place in the mitochondria of eukaryotes while in the cytosol in the prokaryotes. Substrates: Free fatty acids; H2O. Products: One acetyl CoA, one NADH, and one FADH2 for every removal of a two-carbon group from the fatty acid chain. The beta oxidation of fatty acids involves three stages I. Activation of fatty acids in the cytosol II. Transport of activated fatty acids into mitochondria (via carnitine shuttle). III. Beta oxidation proper in the mitochondrial matrix I. Activation of fatty acids First of all, fatty acid Fatty acid protein transporters allow fatty acids to cross the cell membrane and enter the cytosol, since the negatively charged fatty acid chains cannot cross it otherwise. Then, the enzyme Fatty Acyl-CoA Synthase (or FACS) adds a CoA group to the fatty acid chain, converting it to acyl-CoA. In the cytosol of the cell, long-chain fatty acids are activated by ATP and coenzyme A, and fatty acyl-CoA is formed. This process is called as activation of fatty acids. The short-chain fatty acids are activated in mitochondria. b. ATP is converted to AMP and pyrophosphate (PPi), cleaved by pyrophosphatase to two inorganic phosphates (2 Pi). Because two high energy phosphate bonds are cleaved, the equivalent of two molecules of ATP is used for fatty acid activation. II. Transport of activated fatty acids into mitochondria (carnitine shuttle) If the acyl-CoA chain is long, it needs to be transported across the membrane by the carnitine shuttle. For this, the enzyme Carnitine Palmitoyl Transferase I (CPT1)—bound to the outer mitochondrial membrane—convert the acyl-CoA chain to an acylcarnitine chain, which can be transported across the mitochondrial membrane by carnitine translocase (CAT). Once inside the mitochondria, Carnitine Palmitoyl Transferase II (CPT2)—bound to the inner mitochondrial membrane —converts the acylcarnitine back to acyl-CoA. At this point, acyl-CoA is inside the mitochondria and can now undergo beta oxidation. 3. Beta oxidation proper in the mitochondrial matrix The process of fatty acid oxidation, called beta oxidation, is fairly simple. The reactions all occur between carbons 2 and 3 (with #1 being the one linked to the CoA) and sequentially include the following: A. Dehydrogenation to create FADH2 and a fatty acyl group with a double bond in the trans configuration; B. Hydration across the double bond to put a hydroxyl group on carbon 3 in the L configuration; C. Oxidation of the hydroxyl group to make a ketone; and D. Thiolytic cleavage to release acetyl-CoA and a fatty acid two carbons shorter than the starting one. A. Dehydrogenation In the first step, acyl-CoA is oxidized by the enzyme acyl CoA dehydrogenase. A double bond is formed between the second and third carbons (C2 and C3) of the acyl-CoA chain entering the beta oxidation cycle; the end product of this reaction is trans-Δ -enoyl-CoA (trans-delta 2-enoyl CoA). This step uses FAD and produces FADH2, which will enter Electron Transport System and form ATP to be used as energy. B. Hydration In the second step, the double bond between C2 and C3 of trans-Δ -enoyl-CoA is hydrated, forming the end product L-β-hydroxyacyl CoA, which has a hydroxyl group (OH) in C2, in place of the double bond. This reaction is catalyzed by another enzyme: enoyl CoA hydratase. This step requires water. C. Oxidation In the third step, the hydroxyl group in C2 of L-β-hydroxyacyl CoA is oxidized by NAD+ in a reaction that is catalyzed by 3-hydroxyacyl-CoA dehydrogenase. The end products are β- ketoacyl CoA and NADH + H. NADH will enter the citric acid cycle and produce ATP that will be used as energy. Thus in this step β-hydroxyacyl-CoA undergoes dehydrogenation to form β- ketoacyl-CoA in the presence of β-hydroxyacyl-CoA dehydrogenase. The electrons available as a result of dehydrogenation are accepted by NAD+ to form NADH + H+ which immediately exchanges these electrons with oxygen in the Electron Transport System to form ATP molecules. D. Thiolysis Finally, in the fourth step, β-ketoacyl CoA is cleaved by a thiol group (SH) of another CoA molecule (CoA-SH). The enzyme that catalyzes this reaction is β-ketothiolase. The cleavage takes place between C2 and C3; therefore, the end products are an acetyl-CoA molecule with the original two first carbons (C1 and C2), and an acyl-CoA chain two carbons shorter than the original acyl-CoA chain that entered the beta oxidation cycle. This reaction is called as thiolysis as acyl-CoA acetyltransferase (also known as thiolase) in the presence of CoA-SH causes the cleavage of βketoacyl-CoA to form acetyl CoA and the thioester of the original fatty acid with two carbons less. This cleavage occurs as the β carbon ketone group is a good target for nucleophilic attack by the thiol (-SH) group of the coenzyme A. End of Beta Oxidation In the case of even-numbered acyl-CoA chains, beta oxidation ends after a four-carbon acyl-CoA chain is broken down into two acetyl-CoA units, each one containing two carbon atoms. Acetyl- CoA molecules enter the citric acid cycle to yield ATP. In the case of odd-numbered acyl-CoA chains, beta oxidation ensues in the same way except for the last step: instead of a four-carbon acyl-CoA chain being broken down into two acetyl-CoA units, a five-carbon acyl-CoA chain is broken down into a three-carbon propionyl-CoA and a two-carbon acetyl-CoA. Another chemical reaction then converts propionyl-CoA to succinyl-CoA, which enters the citric acid cycle to produce ATP. Ketone Bodies Ketone bodies or “ketones” are substances produced by the liver during gluconeogenesis, a process that creates glucose in times of fasting and starvation. Ketone bodies are water soluble compounds containing ketone groups that are produced in the liver. These compounds are used in healthy individuals to provide energy to the cells of the body when glucose is low or absent in the diet. There are three ketone bodies produced by the liver- i. Acetone ii. Acetoacetate iii. Beta- hydroxybutyrate Why Ketone Bodies are produced? The ketone bodies are produced under prolonged fasting, intense exercise, starvation, diets restricted in carbohydrates, alcoholism and untreated conditions of type I diabetes mellitus, basically at times when the blood glucose level is low. In these conditions, first the glycogen storage is broken down to be used in the form of energy. But soon, the liver runs out of the glycogen storage and as a last resort the fatty acids are broken down to ketone bodies. The ketone bodies are then transported out of the liver and converted into acetyl coenzyme A (acetyl-CoA). The acetyl-CoA then enters the Krebs cycle to be oxidised for energy. Acetoacetate is formed by the fusion of two acetyl coenzyme A molecules by forming a dimer after losing the -CoAs. Acetone on the other hand, is the spontaneous product of decarboxylation of Acetoacetate and gives a distinguishing sweet breath to the person undergoing ketoacidosis. The 3-β-hydroxybutyrate is formed by the reduction of acetoacetate and acetone is the decarboxylated form of acetoacetate. Acetoacetate and 3-β-hydroxybutyrate are predominantly involved in the transport of energy from liver to other tissues. Functions of Ketones Bodies i. Ketone bodies are formed in the liver and exported to other organs as fuel. ii. Acetone, produced in smaller quantities than the other ketone bodies, is exhaled. iii. Acetoacetate and D-β-hydroxybutyrate are transported by the blood to tissues other than the liver (extrahepatic tissues). iv. Then, they are converted to acetyl-CoA and oxidized in the citric acid cycle, providing much of the energy required by tissues such as skeletal and heart muscle and the renal cortex. v. The brain, which preferentially uses glucose as fuel, can adapt to the use of acetoacetate and D-β- hydroxybutyrate under starvation conditions when glucose is unavailable. vi. In this situation, the brain cannot use fatty acids as fuel, because they do not cross the blood-brain barrier. vii. The production and export of ketone bodies from the liver to extrahepatic tissues allow continued oxidation of fatty acids in the liver when acetyl-CoA is not being oxidized in the citric acid cycle. The synthesis of ketone bodies occurs in the liver. The enzymes for ketone body synthesis are located in the mitochondrial matrix. Acetyl CoA, formed by oxidation of fatty acids, pyruvate, or some amino acids, is the precursor for ketone bodies. Ketogenesis occurs through the following reactions- i. The first step in the formation of acetoacetate, which is the enzymatic condensation of two molecules of acetyl-CoA, catalyzed by thiolase; this is simply the reversal of the last step of β oxidation. ii. Acetoacetyl CoA combines with another molecule of acetyl Co-A to produce β- hydroxyβ-methyl glutaryl-CoA (HMG CoA). HMG CoA synthase, catalyzing this reaction, regulates the synthesis of ketone bodies. HMG CoA lyase cleaves HMG CoA to produce acetoacetate and acetyl CoA. iii. The acetoacetate is reversibly reduced by βhydroxybutyrate dehydrogenase, a mitochondrial enzyme, to β– hydroxybutyrate. iv. Acetone is formed in very small amounts from acetoacetate, which is easily decarboxylated, either spontaneously or by the action of acetoacetate decarboxylase. v. Acetoacetate can be reduced by a dehydrogenase to β-hydroxybutyrate. The carbon skeleton of some amino acids (ketogenic) is degraded to acetoacetate or acetyl CoA and, therefore, to ketone bodies, e.g. leucine, lysine, phenylalanine, etc. Utilization (Metabolism) of Ketone Bodies The ketone bodies, being water-soluble, are easily transported from the liver to various tissues. A basic level of ketone is always maintained in blood however it varies according to age, basal metabolic rate and hepatic glycogen storage. The postprandial level of circulating ketone body ranges