ARDS.docx
Document Details
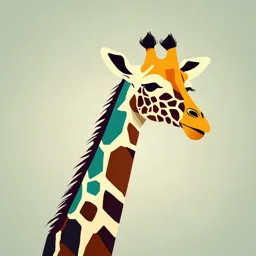
Uploaded by EnergyEfficientForethought
Full Transcript
Acute Respiratory Distress Syndrome Acute respiratory distress syndrome (ARDS) is a clinical syndrome of severe dyspnea of rapid onset, hypoxemia, and diffuse pulmonary infiltrates leading to respiratory failure. ARDS can be caused by diffuse lung injury from many underlying medical and surgical dis...
Acute Respiratory Distress Syndrome Acute respiratory distress syndrome (ARDS) is a clinical syndrome of severe dyspnea of rapid onset, hypoxemia, and diffuse pulmonary infiltrates leading to respiratory failure. ARDS can be caused by diffuse lung injury from many underlying medical and surgical disorders. The lung injury may be direct, as occurs in toxic inhalation, or indirect, as occurs in sepsis (Table 301-1). The clinical features of ARDS are listed in Table 301-2. By expert consensus, ARDS is defined by three categories based on the degrees of hypoxemia (Table 301-2). These stages of mild, moderate, and severe ARDS are associated with mortality risk and with the duration of mechanical ventilation in survivors. The annual incidence of ARDS prior to the COVID-19 pandemic was estimated to be as high as 60 cases/100,000 population. Approximately 10% of all intensive care unit (ICU) admissions involve patients with ARDS. This chapter will focus on non-COVID-19-ARDS. Please see Chap. 199 for more information on COVID. ■ETIOLOGY While many medical and surgical illnesses have been associated with the development of ARDS, most cases (>80%) are caused by a relatively small number of clinical disorders: pneumonia and sepsis (~40–60%), followed in incidence by aspiration of gastric contents, trauma, multiple transfusions, and drug overdose. Among patients with trauma, the most frequently reported surgical conditions in ARDS are pulmonary contusion, multiple bone fractures, and chest wall trauma/flail chest, whereas head trauma, near-drowning, toxic inhalation, and burns are rare causes. The risks of developing ARDS are increased in patients with more than one predisposing medical or surgical condition. Several other clinical variables have been associated with the development of ARDS. These include older age, chronic alcohol abuse, pancreatitis, pneumonia and sepsis (40-60%), [including pandemic COVID pneumonia], and severity of critical illness. Trauma patients with an Acute Physiology and Chronic Health Evaluation (APACHE) II score ≥16 (Chap. 300) have a 2.5-fold increased risk of developing ARDS. ■■CLINICAL COURSE AND PATHOPHYSIOLOGY The natural history of ARDS is marked by three phases—exudative, proliferative, and fibrotic—that each have characteristic clinical and pathologic features (Fig. 301-1). Exudative Phase In this phase, alveolar capillary endothelial cells and type I pneumocytes (alveolar epithelial cells) are injured, with consequent loss of the normally tight alveolar barrier to fluid and macromolecules. Edema fluid that is rich in protein accumulates in the interstitial and alveolar spaces (Fig. 301-2). Proinflammatory cytokines (e.g., interleukin 1, interleukin 8, and tumor necrosis factor α [TNF-α]) and lipid mediators (e.g., leukotriene B4) are increased in this acute phase, leading to the recruitment of leukocytes (especially neutrophils) into the pulmonary interstitium and alveoli. In addition, condensed plasma proteins aggregate in the air spaces with cellular debris and dysfunctional pulmonary surfactant to form hyaline membrane whorls. Pulmonary vascular injury also occurs early in ARDS, with vascular obliteration by microthrombi and fibrocellular proliferation (Fig. 301-3). Alveolar edema predominantly involves dependent portions of the lung with diminished aeration. Collapse of large sections of dependent lung can contribute to decreased lung compliance. Consequently, intrapulmonary shunting and hypoxemia develop and the work of breathing increases, leading to dyspnea. The pathophysiologic alterations in alveolar spaces are exacerbated by microvascular occlusion that results in reductions in pulmonary arterial blood flow to ventilated portions of the lung (and thus in increased dead space) and in pulmonary hypertension. Thus, in addition to severe hypoxemia, hypercapnia secondary to an increase in pulmonary dead space can be prominent in early ARDS. The exudative phase encompasses the first 7 days of illness after exposure to a precipitating ARDS risk factor, with the patient experiencing the onset of respiratory symptoms. Although usually presenting within 12–36 h after the initial insult, symptoms can be delayed by 5–7 days. Dyspnea develops, with a sensation of rapid shallow breathing and an inability to get enough air. Tachypnea and increased work of breathing result frequently in respiratory fatigue and ultimately in respiratory failure. Laboratory values are generally nonspecific and are primarily indicative of underlying clinical disorders. The chest radiograph usually reveals opacities consistent with pulmonary edema and often involves at least three-quarters of the lung fields (Fig. 301-2). While characteristic for ARDS, these radiographic findings are not specific and can be indistinguishable from cardiogenic pulmonary edema (Chap. 305). Unlike the latter, however, the chest x-ray in ARDS may not demonstrate cardiomegaly, pleural effusions, or pulmonary vascular redistribution as is often present in pure cardiogenic pulmonary edema. If no ARDS risk factor is present, then some objective evaluation is required (e.g., echocardiography) to exclude a cardiac etiology for hydrostatic edema. Chest computed tomography (CT) in ARDS Exudative Hyaline Edema Membranes Day: 0 2 7 14 21 . . . Interstitial Inflammation Fibrosis Proliferative Fibrotic FIGURE 301-1 Diagram illustrating the time course for the development and resolution of acute respiratory distress syndrome (ARDS). The exudative phase is notable for early alveolar edema and neutrophil-rich leukocytic infiltration of the lungs, with subsequent formation of hyaline membranes from diffuse alveolar damage. Within 7 days, a proliferative phase ensues with prominent interstitial inflammation and early fibrotic changes. Approximately 3 weeks after the initial pulmonary injury, most patients recover. However, some patients enter the fibrotic phase, with substantial fibrosis and bullae formation. also reveals the presence of bilateral pulmonary infiltrates and demonstrates extensive heterogeneity of lung involvement (Fig. 301-4). Because the early features of ARDS are nonspecific, alternative diagnoses must be considered. In the differential diagnosis of ARDS, the most common disorders are cardiogenic pulmonary edema, bilateral pneumonia, and alveolar hemorrhage. Less common diagnoses to consider include acute interstitial lung diseases (e.g., acute interstitial pneumonitis; Chap. 293), acute immunologic injury (e.g., hypersensitivity pneumonitis; Chap. 288), toxin injury (e.g., radiation pneumonitis; Chap. 75), and neurogenic pulmonary edema (Chap. 37). Proliferative Phase This phase of ARDS usually lasts from approximately day 7 to day 21. Many patients recover rapidly and are liberated from mechanical ventilation during this phase. Despite this improvement, many patients still experience dyspnea, tachypnea, and hypoxemia. Some patients develop progressive lung injury and early changes of pulmonary fibrosis during the proliferative phase. Histologically, the first signs of resolution are often evident in this phase, with the initiation of lung repair, the organization of alveolar exudates, and a shift from neutrophil- to lymphocyte-predominant pulmonary infiltrates. As part of the reparative process, type II pneumocytes proliferate along alveolar basement membranes. These specialized epithelial cells synthesize new pulmonary surfactant and differentiate into type I pneumocytes. Fibrotic Phase While many patients with ARDS recover lung function 3–4 weeks after the initial pulmonary injury, some enter a fibrotic phase that may require long-term support on mechanical ventilators and/or supplemental oxygen. Histologically, the alveolar edema and inflammatory exudates of earlier phases convert to extensive alveolarduct and interstitial fibrosis. Marked disruption of acinar architecture leads to emphysema-like changes, with large bullae. Intimal fibroproliferation in the pulmonary microcirculation causes progressive vascular occlusion and pulmonary hypertension. The physiologic consequences include an increased risk of pneumothorax, reductions in lung compliance, and increased pulmonary dead space. Patients in this late phase experience a substantial burden of excess morbidity. Lung biopsy evidence for pulmonary fibrosis in any phase of ARDS is associated with increased mortality risk. FIGURE 301-3 The normal alveolus (left) and the injured alveolus in the acute phase of acute lung injury and the acute respiratory distress syndrome (right). In the acute phase of the syndrome (right), there is sloughing of both the bronchial and alveolar epithelial cells, with the formation of protein-rich hyaline membranes on the denuded basement membrane. Neutrophils are shown adhering to the injured capillary endothelium and transmigrating through the interstitium into the air space, which is filled with protein-rich edema fluid. In the air space, an alveolar macrophage is secreting proinflammatory cytokines—i.e., interleukins 1, 6, 8 (IL-1, 6, 8) and tumor necrosis factor α (TNF-α)—that act locally to stimulate chemotaxis and activate neutrophils. IL-1 can also stimulate the production of extracellular matrix by fibroblasts. Neutrophils can release oxidants, proteases, leukotrienes, and other proinflammatory molecules, such as platelet-activating factor (PAF). A number of anti-inflammatory mediators are also present in the alveolar milieu, including the IL-1 receptor antagonist, soluble TNF-α receptor, autoantibodies to IL-8, and cytokines such as IL-10 and IL-11 (not shown). The influx of protein-rich edema fluid into the alveolus can lead to the inactivation of surfactant. MIF, macrophage inhibitory factor. (From LB Ware, MA Matthay. The acute respiratory distress syndrome. N Engl J Med 342:1334, 2000. Copyright © 2000 Massachusetts Medical Society. Reprinted with permission from Massachusetts Medical Society.) TREATMENT Acute Respiratory Distress Syndrome GENERAL PRINCIPLES Recent reductions in ARDS mortality rates are largely the result of general advances in the care of critically ill patients (Chap. 300). Thus, caring for these patients requires close attention to (1) the recognition and treatment of underlying medical and surgical disorders (e.g., pneumonia, sepsis, aspiration, trauma); (2) the minimization of unnecessary procedures and their complications; (3) standardized “bundled care” approaches for ICU patients, including prophylaxis against venous thromboembolism, gastrointestinal bleeding, aspiration, excessive sedation, prolonged mechanical ventilation, and central venous catheter infections; (4) prompt recognition of nosocomial infections; and (5) provision of adequate nutrition via the enteral route when feasible. MANAGEMENT OF MECHANICAL VENTILATION (See also Chap. 302) Patients meeting clinical criteria for ARDS frequently become fatigued from increased work of breathing and progressive hypoxemia, requiring mechanical ventilation for support. Minimizing Ventilator-Induced Lung Injury Despite its lifesaving potential, mechanical ventilation can aggravate lung injury. Experimental models have demonstrated that ventilator-induced lung injury can arise from at least two principal mechanisms: “volutrauma” from repeated alveolar overdistention from excess tidal volume (that might also coincide with increased alveolar pressures, or “barotrauma”) and “atelectrauma” from recurrent alveolar collapse. As is evident from chest CT (Fig. 301-4), ARDS is a heterogeneous disorder, principally involving dependent portions of the lung with relative sparing of other regions. Because compliance differs in affected versus more “normal” areas of the lung, attempts to fully inflate the consolidated lung may lead to overdistention of and injury to the more normal areas. Ventilator-induced injury can be demonstrated in experimental models of acute lung injury, in particular with high-tidal-volume (VT) ventilation. A large-scale, randomized controlled trial sponsored by the National Institutes of Health and conducted by the ARDS Network compared low VT ventilation (6 mL/kg of predicted body weight) to conventional VT ventilation (12 mL/kg predicted body weight). Lower airway pressures were also targeted in the low-tidal-volume group (i.e., plateau pressure measured on the ventilator after a 0.5-s pause after inspiration), with pressures targeted at ≤30 cm H2O in the low-tidal-volume group versus ≤50 cm H2O in the hightidal- volume group. The mortality rate was significantly lower in the low VT patients (31%) than in the conventional VT patients (40%). This improvement in survival represents a substantial ARDS mortality benefit. Minimizing Atelectrauma by Prevention of Alveolar Collapse In ARDS, the presence of alveolar and interstitial fluid and the loss of surfactant can lead to a marked reduction of lung compliance. Without an increase in end-expiratory pressure, significant alveolar collapse can occur at end-expiration, with consequent impairment of oxygenation. In most clinical settings, positive end-expiratory pressure (PEEP) is adjusted to minimize Fio2 (inspired O2 percentage) and provide adequate Pao2 (arterial partial pressure of O2) without causing alveolar overdistention. Currently, there is no consensus on the optimal method to set PEEP, because numerous trials have proved inconclusive. Possible approaches include using the table of PEEP-Fio2 combinations from the ARDS Network trial group, generating a static pressure-volume curve for the respiratory system and setting PEEP just above the lower inflection point on this curve to maximize respiratory system compliance, and measuring esophageal pressures to estimate transpulmonary pressure (which may be particularly helpful in patients with a stiff chest wall). Of note, a recent phase 2 trial in patients with moderate-to-severe ARDS demonstrated no benefit of routine use of esophageal pressure-guided PEEP titration over empirical high PEEP-Fio2 titration. Until more data become available on how best to optimize PEEP settings in ARDS, clinicians can use these options or a practical approach to empirically measure “best PEEP” at the bedside to determine the optimal settings that best promote alveolar recruitment, minimize alveolar overdistention and hemodynamic instability, and provide adequate Pao2 while minimizing Fio2 (Chap. 302). Prone Positioning While several prior trials demonstrated that mechanical ventilation in the prone position improved arterial oxygenation without a mortality benefit, a 2013 trial demonstrated a significant reduction in 28-day mortality with prone positioning (32.8 to 16.0%) for patients with severe ARDS (Pao2/Fio2 <150 mm Hg). Thus, many centers are increasing the use of prone positioning in severe ARDS, with the understanding that this maneuver requires a criticalcare team that is experienced in “proning,” as repositioning critically ill patients can be hazardous, leading to accidental endotracheal extubation, loss of central venous catheters, and orthopedic injury. OTHER STRATEGIES IN MECHANICAL VENTILATION Recruitment maneuvers that transiently increase PEEP to high levels to “recruit” atelectatic lung can increase oxygenation, but a mortality benefit has not been established, and in fact, recruitment maneuvers may increase mortality when they are combined with higher baseline PEEP settings. Alternate modes of mechanical ventilation, such as airway pressure release ventilation and highfrequency oscillatory ventilation, have not been proven beneficial over standard modes of ventilation in ARDS management. In one study, lung-replacement therapy with extracorporeal membrane oxygenation (ECMO) was shown to improve mortality for patients with ARDS in the United Kingdom who were referred to an ECMO center (though only 75% of referred patients received ECMO) and thus may have utility in select adult patients with severe ARDS as a rescue therapy. A subsequent study demonstrated that initial use of ECMO in patients with severe ARDS was not superior to use of ECMO as a rescue strategy for patients who failed standard ARDS management. FLUID MANAGEMENT (See also Chap. 300) Increased pulmonary vascular permeability leading to interstitial and alveolar edema fluid rich in protein is a central feature of ARDS. In addition, impaired vascular integrity augments the normal increase in extravascular lung water that occurs with increasing left atrial pressure. Maintaining a low left atrial filling pressure minimizes pulmonary edema and prevents further decrements in arterial oxygenation and lung compliance; improves pulmonary mechanics; and shortens ICU stay and the duration of mechanical ventilation. Thus, aggressive attempts to reduce left atrial filling pressures with fluid restriction and diuretics should be an important aspect of ARDS management, limited only by hypotension and hypoperfusion of critical organs such as the kidneys. NEUROMUSCULAR BLOCKADE In severe ARDS, sedation alone can be inadequate for the patient-ventilator synchrony required for lung-protective ventilation. In a multicenter, randomized, placebo-controlled trial of early neuromuscular blockade (with cisatracurium besylate) for 48 h, patients with severe ARDS had increased survival and ventilatorfree days without increasing ICU-acquired paresis. A subsequent trial demonstrated no mortality benefit for early neuromuscular blockade for 48 h in patients with moderate-to-severe ARDS. This more recent study supports the notion that selective use of neuromuscular blockade might be beneficial in those ARDS patients with ventilatory dyssynchrony despite sedation. GLUCOCORTICOIDS Many attempts have been made to treat both early and late ARDS with glucocorticoids, with the goal of reducing potentially deleterious pulmonary inflammation. Few studies have shown any significant mortality benefit. Current evidence does not support the routine use of glucocorticoids in the care of ARDS patients. OTHER THERAPIES Clinical trials of surfactant replacement and multiple other medical therapies have proved disappointing. Pulmonary vasodilators such as inhaled nitric oxide and inhaled epoprostenol sodium can transiently improve oxygenation but have not been shown to improve survival or decrease time on mechanical ventilation. RECOMMENDATIONS Many clinical trials have been undertaken to improve the outcome of patients with ARDS; most have been unsuccessful in modifying the natural history. While results of large clinical trials must be judiciously applied to individual patients, evidence-based recommendations are summarized in Table 301-3, and an algorithm for the initial therapeutic goals and limits in ARDS management is provided in Fig. 301-5. Please note that these recommendations apply to non-COVID-19-ARDS. Please see recommendations for COVID-19-ARDS in Chap. 199. ■■PROGNOSIS Mortality In the recent report from the Large Observational Study to Understand the Global Impact of Severe Acute Respiratory Failure (LUNG SAFE) trial, hospital mortality estimates for ARDS were 34.9% for mild ARDS, 40.3% for moderate ARDS, and 46.1% with severe ARDS. There is substantial variability, but a trend toward improved ARDS outcomes over time appears evident. Of interest, mortality in ARDS is largely attributable to nonpulmonary causes, with sepsis and nonpulmonary organ failure accounting for >80% of deaths. Thus, improvement in survival is likely secondary to advances in the care of septic/infected patients and those with multiple organ failure (Chap. 300). TABLE 301-3 Evidence-Based Recommendations for ARDS Therapies TREATMENT RECOMMENDATIONa Mechanical ventilation Low tidal volume A Minimized left atrial filling pressures B High-PEEP or “open lung” Bb Prone position Bb Recruitment maneuvers Cb High-frequency ventilation D ECMO Bb Early neuromuscular blockade (routine use) Cb Glucocorticoid treatment D Inhaled vasodilators (e.g., inhaled NO, inhaled epoprosteol) C Surfactant replacement, and other antiinflammatory therapy (e.g., ketoconazole, PGE1, NSAIDs) D aKey: A, recommended therapy based on strong clinical evidence from randomized clinical trials; B, recommended therapy based on supportive but limited clinical data; C, recommended only as alternative therapy on the basis of indeterminate evidence; D, not recommended on the basis of clinical evidence against efficacy of therapy. bAs described in the text, there is no consensus on optimal PEEP setting in ARDS, but general consensus supports an open lung strategy that minimizes alveolar distention; prone positioning was shown to improve mortality in severe ARDS in one randomized controlled clinical trial; recruitment maneuvers combined with high PEEP were shown to increase mortality in one study; ECMO may be beneficial in select patients with severe ARDS; early neuromuscular blockade demonstrated a mortality benefit in one randomized controlled trial in patients with severe ARDS but was not replicated in a subsequent study, suggesting routine use of early neuromuscular blockade in all subjects with moderate-severe ARDS may not be beneficial. Abbreviations: ARDS, acute respiratory distress syndrome; ECMO, extracorporeal membrane oxygenation; NO, nitric oxide; NSAIDs, nonsteroidal anti-inflammatory drugs; PEEP, positive end-expiratory pressure; PGE1, prostaglandin E1. The major risk factors for ARDS mortality are nonpulmonary. Advanced age is an important risk factor. Patients aged >75 years have a substantially higher mortality risk (~60%) than those <45 (~20%). Moreover, patients >60 years of age with ARDS and sepsis have a threefold higher mortality risk than those <60 years of age. Other risk factors include preexisting organ dysfunction from chronic medical illness—in particular, chronic liver disease, chronic alcohol abuse, and chronic immunosuppression (Chap. 300). Patients with ARDS arising from direct lung injury (including pneumonia, pulmonary contusion, and aspiration; Table 301-1) are nearly twice as likely to die as those with indirect causes of lung injury, while surgical and trauma patients with ARDS—especially those without direct lung injury—generally have a higher survival rate than other ARDS patients. Increasing severity of ARDS, as defined by the consensus Berlin definition, predicts increased mortality. Surprisingly, there is little additional value in predicting ARDS mortality from other parameters of lung injury, including the level of PEEP (≥10 cm H2O), respiratory system compliance (≤40 mL/cm H2O), the extent of alveolar infiltrates on chest radiography, and the corrected expired volume per minute (≥10 L/min) (as a surrogate measure of dead space). Functional Recovery in ARDS Survivors While it is common for patients with ARDS to experience prolonged respiratory failure and remain dependent on mechanical ventilation for survival, it is a testament to the resolving powers of the lung that the majority of patients who survive regain nearly normal lung function. Patients usually recover maximal lung function within 6 months. One year after endotracheal extubation, more than one-third of ARDS survivors have normal spirometry values and diffusion capacity. Most of the remaining patients have only mild abnormalities in pulmonary function. Unlike mortality risk, recovery of lung function is strongly associated with the extent of lung injury in early ARDS. Low static respiratory compliance, high levels of required PEEP, longer durations of mechanical ventilation, and high lung injury scores are all associated with less recovery of pulmonary function. Of note, when physical function is assessed 5 years after ARDS, exercise limitation and decreased physical quality of life are often documented despite normal or nearly normal pulmonary function. When caring for ARDS survivors, it is important to be aware of the potential for a substantial burden of psychological problems in patients and family caregivers, including significant rates of depression and posttraumatic stress disorder. Please see Chap. 199 for information regarding COVID prognosis and recovery.