Marine Biotechnology Notes PDF
Document Details
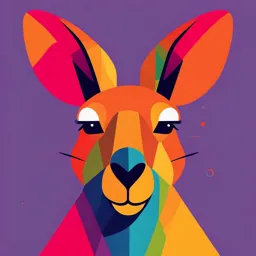
Uploaded by RapidVanadium9602
Joel Sendra Peris
Tags
Summary
These notes provide an introduction to marine biotechnology, covering topics such as marine biodiversity, the differences between marine and terrestrial organisms, research in marine biotechnology, and natural products/secondary metabolites. It discusses the various ways to research marine organisms and compounds, and highlights the vast potential of marine life in terms of natural products.
Full Transcript
Unit 1: Introduction to Marine Biotechnology Joel Sendra Peris 3º Marine biotechnology Index 1. Marine biodiversity and blue biotechnology......................................................................1 2. Marine organisms vs. terrestrials..........
Unit 1: Introduction to Marine Biotechnology Joel Sendra Peris 3º Marine biotechnology Index 1. Marine biodiversity and blue biotechnology......................................................................1 2. Marine organisms vs. terrestrials......................................................................................1 3. Research in Marine Biotechnology...................................................................................2 4. Natural products or secondary metabolites?....................................................................3 5. Bioprospection of the marine environment and NMP screening techniques....................7 1. Marine biodiversity and blue biotechnology – Blue Biotechnology → The application of science and technology to living aquatic organisms for the production of knowledge, goods and services (OECD, 2016). – Currently more than 50% of the drugs on the market are derived from natural terrestrial products. However, because these sources are being exploited and depleted, new sources of nat- ural products are emerging in the marine environment, since… The oceans are the centre of global biodiversity and are considered an abundant source of biological and chemical diversity. They cover more than 70% of the earth's surface and contain more than 300,000 de- scribed species of plants and animals. – This makes the marine environment one of the most diverse regions in the world and the oceans a new and untouched source of potentially useful compounds. 2. Marine organisms vs. terrestrials – Marine organisms → They are rich in bioactive metabolites and their natural products are of- ten of new chemical structure and usually different from their terrestrial counterparts. – The unique properties of marine organisms are the product of their exclusively marine life and the effect exerted by the characteristics of seawater on their chemistry-physiology and metabolism in addition to other adaptations, for example, flexibility of algae in the area intertidal against the rigidity of plants (thanks to the development of gas vesicles or vacuoles to guarantee their buoyancy and light capture, as in Fucus vesiculosus). – Example of a rigid terrestrial plant due to the composition of its cell wall. 1 – Example of a flexible marine plant due to the composition of its cell wall that also has other properties: Presence of polyanionic polysaccharides in the cell wall. The sulfate and carboxyl groups of the cell wall (groups anionic) act like main complexes collectors of metal ions. When there is a high concentration of metal ions in the aquatic environment, the cell wall of the algae prevents their entry into the cytoplasm, acting as an exclusion mechanism, an ionic barrier. At low concentrations of ions in the medium, the high capacity of the cell wall of brown algae (such as Padina gymnospora) in ion exchange was demonstrated, which is 3.5 times higher than in terrestrial plants. 3. Research in Marine Biotechnology – The first investigations related to the use of marine organisms as sources of bioactive metabolites and potentially useful natural marine products started → 1960s. Initially directed to the study of secondary metabolism and then to the discovery of organ- isms and metabolites with potential activities for industrial and biomedical uses. – However, one of the main limitations of the development of bioproducts of marine origin is the availability of biological material. The natural abundance of organisms would not support de- velopment based on natural harvesting. – Some options for the sustainable use of marine resources are: Chemical synthesis, aquaculture of the organism of interest, cell culture, molecular cloning and biosynthesis. Options that require knowledge of the fundamental biochem- ical pathways by which the substances are synthesized. – Example of the potential: Only prokaryotic and eukaryotic microorganisms comprise a vast and undocumented ex- isting biological diversity in the ocean. A phylogenetic, biochemical and physiological diversity that far exceeds that found in the macroorganisms. However, current research suggests that less than 1% of the total diversity of species of marine microorganisms can be cultured by commonly used methods. 2 This means that the application potential of the compounds produced by 99% of marine microorganisms is still unknown being unexploited resources for novel bioactive com- pounds 4. Natural products or secondary metabolites? – Natural product → Any well-defined organic molecule produced by a living organism and not restricted to a secondary metabolite (considered primary metabolite, but not all). – Secondary metabolite → Produced by the alternate biochemical pathway of organisms (when it’s under stress conditions). However, the terms sometimes refer to the same compound. – The confusion is due to the fact that bioprospecting is frequently aimed at obtaining bioact- ive natural products that are generally secondary metabolites. * Example → Ink of an octopus. It’s not necessary to live, not use for eating, reproducing, etc. Also the poisons (Conus magus, produce a poison more potent than morphine. It’s not used be- cause it has to be administrated in the vertebral column). – Koseel was the first to introduce the term "secondary metabolite" in 1891. Primary metabolites are essential for the life and reproduction of cells, secondary metabolites, on the other hand, are only found accidentally and are not essential for the life, for their growth, development and reproduction or survival of the organism that pro- duces them, although they can confer adaptive advantages to the species for their sur- vival in the biological community and environment. The secondary metabolism responsible for these properties is defined as "the biochem- ical pathways that are not necessarily required for the growth or reproduction of an or- ganism, but can be demonstrated genetically, biochemically or physiologically". 3 – Figure → Central metabolic pathways for the production of secondary metabolites: – Main characteristics of secondary metabolites: They can be found in any group of organisms without distinction (sometimes they are formed by relatively small groups of organisms of specific taxonomy, at other times by spe- cific strains but not by taxonomically defined species). They are not essential for growth and reproduction. Usually they show inverse correlation between specific growth rate and the formation of secondary metabolites (generation of metabolites under stress conditions of the organism). Generated as a result of defence mechanisms and / or excessive metabolic production. Formation extremely dependent on environmental conditions. They confer selective and adaptive advantage to the organisms that produce them. A significant number have no apparent biological function. They are smaller and chemically more diverse than the primary metabolites (proteins, lipids, nucleic acids, carbohydrates). They are generated by branched pathways of primary metabolism. 4 As they are dependent on environmental conditions, its production can be regulated by the cultivation conditions. – When metabolic regulation is efficient, the intermediate and terminal products of the primary pathways of organisms, do not accumulate. Instead, certain organisms have genes to produce secondary metabolites in addition to genes necessary for growth. – In these organisms certain steps of the primary metabolism lack regulation, resulting in the oversynthesis of terminal and intermediate products of the primary pathways. In metabolic stress, such accumulated reserves of intermediates can induce subsidiary pathways to form secondary metabolites. * Algae → Stress them with T, salinity, light, hardness of water. – For example, the combination of the fatty acid biosynthetic pathway with monosaccharide biosynthetic pathways has resulted in the formation of macrocyclic antibiotics or macrolides. 5 – This reflects the process by which secondary metabolic pathways are likely to evolve. – Random recombination and mutation of existing genes each time results in the formation of a new chemical entity in a cell. – If the production of the new compound is advantageous, this new biosynthetic capacity will be 'imprinted'. – Example → The catalytic efficiency of enzymes for a new substrate or reaction will be optim- ised or new, more suitable enzymes will be synthesised. In this situation, regulation will be imposed on the new pathway and resistance mechanisms will develop to protect the cell from high concen- trations of its new metabolite and storage or excretion mechanisms will evolve. – Arrangement of genes for the biosynthesis of natural products: This process is used for the discovery of natural marine products through combinatorial biology. Technique by which new genes are generated that are deliberately mixed with pathway genes biosynthetic different. When the genetic process is complemented with an integrated bioassay system to identify and isolate recombinant clones, natural products can be produced with a wide range of biological activities and a high probability of structural novelty (against virus, cancerogenic cells, etc.). – That is, by studying the biosynthesis pathways from primary and secondary metabolism, combinatorial biology and activity bioassays, natural products of very diverse characteristics can be obtained. – However, these routes still do not take advantage of the full potential of natural products that already exist in the marine environment. – So… How to discover new natural products in the marine environment? 6 5. Bioprospection of the marine environment and NMP screening techniques – Bioprospecting → Selective research on biological diversity and local knowledge in order to obtain valuable genetic and biochemical resources from a commercial point of view (CONABIO, 1998). The bioprospecting considers the preservation of biodiversity and its sustainable use. – The use and exploitation of marine resources represents for countries with direct access to the sea an opportunity for their development and can represent a strategy to know, use and con- serve their resources. – Screening programs → The usual steps to obtain a new substance of marine origin include the following steps: 1. Collection and identification of the organism or organisms to be studied. 2. Isolation of the substance. 3. Determination of the structure. 4. Study of bioactive properties. 5. Molecular-genetic enhancement of the organism to overexpress the identified metabol- ite or bioactive substance. – 1) Collection and identification of the organism to study: Advantages: Generally, a small amount of tissue is required (in the case of microorganisms isolated from the water column or sediment, the resource is “infinite”) If the organism can be cultivated, it is not required to go back and alter the habitat again. Moreover, it ensures an adequate supply of biomass for scientific and economic de- velopment. The same organism can generate different bioactive compounds. 7 The production of metabolites can be regulated by culture conditions. Reproducible metabolite production is guaranteed, if the metabolite can be industrially scaled up (microorganisms). Disadvantages: Sampling is often very expensive and sometimes it is limited to manual extraction Scientific divers (depth limitation among others) or use of adequate material (limited to collection during campaigns) (different for sampling macroorganisms or microorgan- isms). Microorganisms because its high rates of division produce superior yields compared to macro-organisms. Microorganism are able to be grown in controlled and small-sized environments. Therefore, the risks of dispersal of the organisms are avoided. Example → Leaks of algae and / or fish in “open” farms. Example → Obtaining biodiesel from the cultivation of microalgae VS intensive soybean cultivation. (Aerial seed dispersal could be a problem and by requiring so much space, dis- places the cultivation of other foods such as wheat). – 2) Isolation of the substance: To isolate a substance, chromatographic techniques such as column chromatography or HPLC are usually used. – 3) Determination of the chemical structure: To characterise a natural product it is necessary to carry out a study of its chemical structure, which requires determining the purity, the elemental composition, as well as its empirical and molecular form. 8 Steps to follow: Determination of the physical characteristics → Melting or boiling point, refractive in- dex, etc. Determination of elemental composition (generally by mass spectrometry -MS-). Determination of the empirical and molecular formula (analysis by spectroscopy IR, or magnetic resonance imaging NMR) Isolation and determination of natural products (analysis by liquid chromatography - HPLC-, or gases -GC). Currently hybrid techniques such as HPLC-MS are very useful. – 4) Study of bioactive properties: Chemical tests and / or bioassays aimed at determining each of the properties of the isol- ated substance. Examples: Antioxidant activity through the DPPH test. Anti-inflammatory activity by the TPA test. Antiproliferative activity in tumour cells through cultures of different cell lines. In vitro assays In vivo assays – So far, what would be a "basic screening" program. – However, there is currently the advanced screening, which is based on the conjunction of techniques and processes through the combination of genomics, bioinformatics, combinatorial chemistry, and high-efficiency or high-throughput culture and screening methods (high through- put screening, HTS), which makes it possible to analyse and characterize a very high number of samples simultaneously in short periods of time. 9 – Image → Reader multi-modal microplates (384 wells) Multiple detectors in parallel for ultra-fast measurements and filter-based system for cell- based assays. Charge/discharge time → 6 sec/ plate. – In any case, the HTS tests developed to evaluate the determined activity of some natural product or metabolite must satisfy the aspects of: Real simulated of the cell, tissue or other natural component when necessary. Possibility of estimating the main parameters that characterize the metabolite of interest. Accurate, fast and efficient. Possibility of being automatic. – The goal is to reduce the time it takes for a drug or substance to reach the market. 10 Unit 2: Biotechnological applications from marine organisms Joel Sendra Peris 3º Marine biotechnology Index 1. Sponges........................................................................................................................... 1 1.1. Classification and distribution....................................................................................1 1.2. Main characteristics...................................................................................................2 1.3. Biotechnological applications....................................................................................2 2. Cnidarians........................................................................................................................ 6 2.1. Classification and distribution....................................................................................6 2.2. Main characteristics...................................................................................................7 2.3. Biotechnological applications....................................................................................7 3. Annelids.......................................................................................................................... 11 3.1. Classification and distribution..................................................................................11 3.2. Main characteristics.................................................................................................12 3.3. Biotechnological applications..................................................................................13 4. Molluscs......................................................................................................................... 15 4.1. Classification and distribution..................................................................................15 4.2. Main characteristics.................................................................................................15 4.3. Biotechnological applications..................................................................................16 5. Lophophores.................................................................................................................. 18 5.1. Main characteristics.................................................................................................18 5.2. Classification and distribution..................................................................................18 5.3. Biotechnological applications..................................................................................19 6. Crustaceans................................................................................................................... 21 6.1. Main characteristics.................................................................................................21 6.2. Classification and distribution..................................................................................22 6.3. Biotechnological applications..................................................................................22 7. Echinoderms.................................................................................................................. 26 7.1. Main characteristics.................................................................................................26 7.2. Classification and distribution..................................................................................27 7.3. Biotechnological applications..................................................................................27 8. Tunicates........................................................................................................................ 29 8.1. Main characteristics.................................................................................................29 8.2. Classification and distribution..................................................................................29 8.3. Biotechnological applications..................................................................................30 9. Fishes............................................................................................................................. 32 9.1. Main characteristics.................................................................................................32 9.2. Classification and distribution..................................................................................32 9.3. Biotechnological applications..................................................................................33 1. Sponges 1.1. Classification and distribution – Although we find records of sponges as early as 350 BC, they were not classified as inverteb- rates until the 17th century. – The phylum Porifera (sponges) consists of about 6000 species, of which only about 150 are freshwater species, while the rest are marine. – They have a very simple taxonomic organisation, with no respiratory, circulatory or digest- ive system. – Their body is formed externally by pores (ostioles) that allow the absorption and expulsion of water flow, a process by which they achieve their respiration, feeding and reproduction. – They are classified into three groups: Calcareous → Their spicules are made of calcium carbonate and have no sponge fibres. They are usually small in size (Clatrina canariensis). Shallow waters Hexactinellids → Also known as silica sponges, they have silica spicules and have a glassy appearance (Hexactinelida sp.). Deep waters Demosponges → These behave like sponges without spicules like the bath sponge or kit- chen sponge, which keep their shape thanks to sponge fibres, a protein close to silk. This is the type in which most sponges are found. They are usually brightly coloured in red, green and blue due to the granules found inside the sponge cells. + 95 % taxon (Xestospongia testudinaria) 1 1.2. Main characteristics – Sponges filter the water by removing suspended particles from the medium, serving as a nat- ural filter for their habitat, and from this process they also obtain the oxygen necessary for life. – Many species have a diet based on microscopic particles, bacteria, protozoa and green al- gae or cyanobacteria. There are also carnivorous species that feed on tiny crustaceans. – Due to the particularity of their diet, and not having a mouth, they feed through pores located on the outside of their body, with which they can capture water from their habitat. The water passes through a filter where it absorbs the nutrients necessary for its diet. – This process is essential for the purification of marine waters, as even the smallest sponge can filter about 20 litres per day. – Marine sponges are a rich source of potent natural products, some of which are considered very important compounds for drug development. – More than 5300 different natural compounds have been discovered from sponges and sev- eral hundred new compounds are added each year. 1.3. Biotechnological applications – In sponges we find many species that not only use their secondary metabolites to protect themselves, but also use them to compete for space and food against other organisms that may even be of the same taxon. – This is especially true for sponges that have a tree-like and encrusting growth (extension into the rock). In these cases they release substances with cytotoxic activity that can be used in anti- tumour drugs (Haliclona permollis, the sponge of this image). 2 – The first studies of sponges as a source of therapeutic agents were in 1950 when Bergmann and co-workers succeeded in isolating three nucleosides with structural novelty: Spongothymidine and sponguridine from the Caribbean sponge Criptotetia criptaa. – These molecules enabled the first synthetically modified drugs of marine origin: Ara-C (cytarabine) → An antitumour agent Ara‐A (Acyclovir/Zovirax) → Inhibits viral DNA polymerase and DNA synthesis of herpes, vaccinia and varicella zoster viruses. Zovirax is the world's best‐selling antiviral drug. – In 2001, rosacelose, a new natural product with anti-HIV activity, was extracted from an aqueous extract of the marine sponge Myxilla rosacea. This compound inhibits the formation of multi-nucleated cells (syncytia) after infection with the virus. – Halichondrin B is a complex isolated from the marine sponge Halichondria okadai, with ex- traordinary potential as an anti-tumour agent. – Haliclona sp. → 190 different metabolites with antifouling (avoid animals to attach to the sur- face of the boats), antimicrobial, antifungal and antimalarial activity have been found in this genus. – Ircinia fasciculata → Potent antimicrobial activity against pathogens. 3 – Compounds isolated from the sponge Hamigera tarangaensis show 100% inhibition of herpes and polio viruses in vitro, while other compounds isolated from the sponge Petrosia weinbergi show activity against feline leukaemia, influenza and corona viruses. – Ethyl esters of esculetin‐4‐carboxylic acid isolated from the Brazilian marine sponge Axinella corrugata show bioactivity against the SARS coronavirus causing COVID‐19, inhibiting 3CL protease, among others, as it appears to be involved in the release of viral replicative proteins. – Mycalysin A and B are nudonucleoside analogues isolated from the marine sponge Micale sp. Mycalysin A inspired the synthesis of Remdesivir, a drug indicated to treat COVID‐19 disease. Remdesivir sold under the brand name of Veklury. – Psammaplin A (PSA) → Phenol isolated from the marine sponge Pseudoceratin purpurea. It has a wide range of bioactivities, especially for anti‐tumour activity. It exhibits anticancer activities by modulating different human enzymes, which in turn regulate replication, transcription, differentiation, apoptosis, proliferation, tumour invasion and DNA migration. It acts as an antiproliferative agent in several human cancer cell lines, such as endo- metrial, breast, brain and lung cancer. 4 – According to the WHO (World Health Organisation), new antimalarial drugs are now needed to cope with the growing number of patients infected with multi‐resistant strains of Plasmodium sp. causing a major health problem in many southern countries. Manzamine alkaloids → The most promising antimalarial compounds that have been dis- covered in several sponges such as the Okinawan sea sponge Anphimedon sp. or Haliclona sp. Kalihinol A → Extracted from Acanthella sp., show selective antimalarial activity against Plasmodium falciparum. A series of free carboxylic acids from the sponge Diacarnus levii shows selective activ- ity against strains of Plasmodium falciparum. – Biofouling organisms such as mussels, barnacles or macroalgae cause serious problems in ship propellers, power plant cooling systems and aquaculture materials. – Natural marine antifouling molecules are less toxic than the chemical equivalents com- monly used in paints. Fatty acid extracted from the sponge Crella incrustans has antifouling activity against various invertebrates. Polyacetylenes calispongin B and calitriol C isolated from. Callyspongia sp are also anti- fouling against barnacle larvae. Cyclopeptides isolated from the sponge Geodia barretti have been shown to be effective in preventing the settlement of sea acorn and mussel colonies. 5 – Apart from these pharmacological, environmental and industrial applications, sponges are also important sources of collagen and the silicon spicules they contain are excellent light transmitters and have advantages over optical fibres (higher refractive indices) avoiding the self‐ shading effect in microalgae cultivation. – There are some trials of sponge cultures under controlled conditions. Some species can be cultured, but these are very complex systems and are not cost‐ef- fective, so products derived from sponges are actually synthesised artificially (chemical synthesis or in other organisms). 2. Cnidarians 2.1. Classification and distribution – Cnidarians → Phylum of about 10,000 species of relatively simple animals (video). – Habitat → Exclusive to the aquatic environment. Mostly marine animals, including corals, hy- drozoans, jellyfish and anemones among others. They inhabit all seas and depths (preferably rocky shores) and range in size from 8 to 15 grams of biomass per liter of culture). Vigorous mixing to ensure high frequency L:D cycles (...). Avoid inhibition of growth due to accumulation of O2. Maintain optimal temperature and pH. 5 3. Microalgae cultivation – Crop factors affecting the growth and accumulation of metabolites in microalgae. – There are several variables that affect the growth and accumulation of metabolites in mi- croalgae. It is important to determine the optimal growth conditions, since it is known that the yield rate (biomass) for the same genus of microalgae it may be different. The light CO2 Nitrates and phosphates Temperature pH Turbulence and mixed * Image → Lag phase, then exponential phase, stationary phase and death phase. In a race- way, stationary phase will imply empting all of it. So it’s better to harvest at the exponential phase. (…) (strains) – The intensity of the light is one of the most important factors for the photosynthetic growth of microalgae. – Cropping systems of microalgae they can be illuminated by artificial light, sunlight, or both. – Systems bioreactors employees at the laboratory level are illuminated internally or extern- ally by artificial light with fluorescent lamps and light-emitting diodes (light emitting diodes, LED) among others. 6 – The specific growth rate of the microalgae It depends on the intensity of the light: – This growth pattern in relation to light intensity is observed in most species of microalgae. * Excess → Photoinhibit, 15 best?? – The CO2 It is the carbon source most used in crops of microalgae. – Since the microalgae can live under high concentrations of carbon dioxide, greenhouse gases, nitrogen dioxide and pollutants in the atmosphere can be sufficient nutrients for microal- gae. – Tetraselmis sp., Chlamydomonas sp., y Nannochloris sp. need less than 15% CO2 for their growth, while species like Scenedesmus sp. y Cyanidium caldariu they tolerate concentra- tions from 80 to 100% respectively. * Interesting when (...). 7 – Other important nutrients are nitrates and phosphates, as well as sodium and silicates. There are several formulated culture solutions available but the requirements are different for each variety of microalgae. – Therefore, according to the objective of the experiment, the composition of the culture me- dium will be defined. For example, if the goal is high biomass productivity (g / L), high concen- trations of nitrates and phosphates will be required. However, to induce the production of meta- bolites and compounds of commercial interest, the nitrate concentration is manipulated to sim- ulate a stressful environment. – Example → A high carbon-nitrogen (C/N) ratio turns out to be efficient to induce the biosyn- thesis of astaxanthine (It is achieved by limiting the nitrogen supply in the presence of an excess of organic carbon sources, such as acetate and glucose in crops mixotrophic). – Example → An excess of Fe can stimulate the accumulation of astaxanthine in Haematococ- cus pluvialis. – Temperature → One of the most important environmental factors that affect the growth and development of living organisms. Therefore, it is required to know an optimal value for a maximum growth rate. Temperature is also important for the dissociation of carbon molecules, making it avail- able for photosynthesis. Temperature influences respiration and photorespiration more markedly than in photosynthesis. However, if the CO2 or light is a limiting factor for photosynthesis, the influence of tem- perature is negligible. The optimum temperature for growing microalgae It is generally between 20 and 24 ºC, however, these can vary depending on the culture medium, the species and the strain used. Commonly, crops of microalgae tolerate temperatures between 16 and 27 ºC. T 35 ºC → Turns out to be lethal for a great number of species. Changes in temperature can also cause alterations in many of the metabolic pathways, including carotenoid biosynthesis. 8 Examples: Chlorella zofingiensis → By increasing the culture temperature there were changes in the absorption efficiency associated with a variation in cell size and pigment levels. Muriellopsis sp. → Cellular accumulation of lutein and astaxanthine when grown at a temperature higher than 33 ºC, however the volumetric level increases up to six times more when the temperature is 28 ºC. Some algae have also been shown to increase carotenoid accumulation at a temper- ature of 40 ºC compared to the rate obtained at the optimum growth temperature of 28 ºC. – The microalgae have different requirements of pH for their growth. At alkaline pH levels, the availability of CO2 may be limiting for growth and photosynthesis of microalgae. The pH range for most crops of microalgae it is between 7 and 9, with an optimal range of 8.2 to 8.7 (although some authors affirm that the optimum is at neutral pH 7.5). An optimal pH in the culture is generally achieved thanks to aeration with air enriched with CO2. In the case of high cell density cultures, the addition of carbon dioxide corrects an in- crease in pH, which can reach a limit value of 9 for cell growth. Buffer solutions are generally used to adjust and maintain the pH of the medium. The pH increases as the age of the crop is older, this is due to the accumulation of minerals and the oxidation of nutrients. Therefore, it is recommended that the initial pH of the culture medium is adjusted to 6.5 before being inoculated. – Turbulence and mixed: For any type of reactor used in the cultivation of algae an efficient mixing must be provided in order to produce a uniform dispersion of the algae, thus eliminating the con- centration gradients of light, nutrients (including CO2) and temperature. Excess mechanical agitation causes turbulence, which can cause permanent damage to the cell structure, affecting growth and metabolite production. On the contrary, insuffi- cient agitation will cause sedimentation and cell death. 9 4. Cyanobacteria – The interest of biotechnology cyanobacterial not only lies in the manipulation of genes to produce improved species through recombinant DNA techniques, but also in the use of unmodi- fied organisms to generate natural products that are unique, that are produced more efficiently or at a lower cost. – Because the cyanobacteria combine all the metabolic flexibility of eubacteria, with nutrition phototrophic oxygenic of eukaryotic algae, suggest a promising future for many applications: 5. Biotechnology applications of cyanobacteria – Arthrospira platensis (Spirulina) → Cyanobacteria most used for both nutritional and thera- peutic aspects. It has a high protein content, which is comparable to meat in terms of dry weight (30- 45%), which is why it is used in the human diet in pills, capsules, desserts, chocolate bars, etc. In animal diets it is intended as a supplement or to replace conventional proteins such as fish or soy meat. 10 It also has a high content of vitamins and minerals, being one of the most abundant sources of vitamin B12, as well as vitamins B1, B2 and provitamin A (β-carotene), which has antioxidant properties. Arthrospira it is also an important source of acid γ-linoleic and polyunsaturated fatty acids and has been used as a source of high added value pigments such as phycocyanin and included in crustacean and fish diets to increase body pigment levels. Arthrospira exerts an immunostimulant effect by increasing resistance to infection in humans, mammals, chickens and fish, as well as by stimulating the production of antibod- ies and cytokines. Compounds like sulfolipids are effective against HIV, while preparations obtained from biomass cyanobacterial are active against the herpes virus, cytomegalovirus, the influenza virus and others. Because its cell wall materials resemble those of bacteria, it is more easily digested than most bacteria. For all these reasons, the Arthrospira It is included in strategic programs of countries such as China, Thailand, Japan, Taiwan, Israel and the USA. – Among the chemical compounds produced by cyanobacteria is the photoproduction of hy- drogen, a clean and renewable energy source, by cyanobacteria such as Synechococcus, Ana- baena cylindrical, Arthrospira and others. – Also, cyanobacteria can be used for photoproduction and release of nitrogenous com- pounds including ammonium and amino acids. – Certain nitrogen-fixing cyanobacteria is used as biofertilizers natural in rice fields. – The ability of cyanobacteria to transform simple compounds into complex organic mo- lecules through photosynthesis can be applied for the enrichment of a particular isotope, such as 14C. 11 – Also, certain isotopic carbon fractions related to biosynthesis of lipids in cyanobacteria can be used as biomarkers of cellular changes over time. – The mobilization of glycogen and other carbon molecules in response to abrupt changes in salinity has been studied in the marine cyanobacteria Agmenellum quadruplicatum, by NMR us- ing al isotope 13 C. 13 – Stable isotopes such as CO2 are indicated for medical diagnostic tests, while pigments such as phycoerythrin and allophycocyanin are used in analysis cytometric and histochemicals (not enter, only know that it is for medical diagnosis test). – The application of phycobiliproteins has a wide market like immunotracers, food, drugs for the cosmetic industry, in clinical diagnosis (as a fluorescent agent) and deodorant production. – Image → Agmenellum quadruplicatum. – In addition to those derived from the crop itself (sink of CO2), cyanobacteria have significant potential for the biodegradation of toxic compounds from polluted waters and soils. They degrade aromatic hydrocarbons such as naphthalene, n-alkanes from crude oil from oil spills, and pesticides. They are capable of removing toxic metals from polluted waters of the mining and indus- trial industries such as cadmium, copper, mercury and zinc. Several of these metals can be removed or even recovered from effluents through waste water processes bioaccumulation and bioabsorption by cyanobacteria. – On the other hand, there are other products cyanobacteria with the ability to bind metals, such as extracellular organic acids and aa. 12 – Likewise, the presence of cyanobacteria in urban wastewater effluents and their great ca- pacity to purify organic and inorganic pollutants places cyanobacteria as important m.o. in waste water treatment programs like bioremediation of domestic and industrial effluents. Recent environmental applications include aspects of biocontrol and bioremediation of aquaculture effluents (Paez-Osuna, 2001). – Figure → All-gas Project. – The natural products of cyanobacteria are classified within the following structural classes: acetogenins, bromophenols, fatty acids, terpenes and sterols, alkaloids, amides, cyclic oligosac- charides and cyclic peptides. Whose potential uses include, for example, antibiotics, antifungals, antitumour activity, cytotoxicity to cancer cells, anti-inflammatories and also neuromuscular toxicity. 13 Unit 3.2: Biotechnological applications of macroalgae Joel Sendra Peris 3º Marine biotechnology Index 1. Macroalgae.......................................................................................................................1 2. Importance of macroalgae culture....................................................................................2 3. Macroalgae culture...........................................................................................................4 4. Biotechnological applications of macroalgae..................................................................11 1. Macroalgae – The Brown algae are grouped in division Phaeophyta, which is characterized by bringing to- gether a large number of species, which present various morphologies, from filamentous algae with a simple structure to algae that can reach several meters in length and with very complex structures. Phaeophyta contains 265 genera with about 1,500-2,000 species, mainly marine as only six genera are from freshwater. – Its brown colour (yellowish green to brownish) is due to the presence of a xanthophyll, a pig- ment that protects from sunlight, called fucoxanthin, which mask the colour of chlorophylls a and c, of the beta carotenes and other xanthophylls. – As reserve substances they have polysaccharides, they do not contain starch, their cell walls are composed of cellulose and alginic acid (importance in biotechnology). – The term of green algae refers by translation to the scientific name Chlorophyta as they have chloroplasts that contain chlorophyll a and b, giving them a bright green colour, as well as the ac- cessory pigments beta carotene (red-orange) and xanthophylls (yellow). The cell walls of green al- gae usually contain cellulose, and they store carbohydrate in the form of starch. As green algae, some 10,000 different species have been described so far, being the most diverse of all algae. They are found in very diverse habitats, including very adverse condi- tions. Only 10% of the species are marine, the rest are freshwater. Many are unicellular, fre- quently flagellated, but others develop multicellular thalli that are never very complex. – The red algae, Rhodophytas, owe their colour to the pigment phycoerythrin. Rhodophytes are photosynthetic and their chloroplasts (called rhodoplasts) present some specific characterist- ics of their group, they are surrounded by two membranes and contain chlorophyll a, in addition to the pigments phycobiliproteins and carotenoids. They are a group of 5,000 to 6,000 species of a great diversity of shapes and sizes. * Image → Wild collection constant, the cultivation is growing. Thanks to aquaculture. 1 2. Importance of macroalgae culture – The algae wakame Undaria pinnatifide, "kombu” Laminaria japonica are collected by artisanal fishermen, they are dried and in some cases they are chopped and then they are commercialized. – For the production of “dry minced alga”, which is the product of the harvesting of wild algae there is a sequence of steps: 1. Collection of algae, either washed up on the shore, or collected from authorized areas. 2. The algae are dried in the sun. 3. Transport to mincing plants (they can have different sizes), from drying areas. 4. Bagging and shipping. – The growing demand for macroalgae has stimulated scientists and technicians to initiate pro- grams to cultivate them, especially those used for human consumption. In some countries such as Japan and China, the cultivation of algae represents an industry that is expanding and in other parts of the world they are working intensively to cultivate them for both; food and industrial pur- poses. – Example → Brown algae are interesting for their high acid content alginic which can be ex - tracted and used as alginate in the pharmaceutical, food, cosmetic and textile, among others in- dustries. – The cultivation is not only solving the problem of over-exploitation that some natural popula- tions of algae have suffered, but is also facilitating their collection, in addition to using those spe- cies that live in areas that are difficult to access; It also reduces the costs of the operation and en- sures that the raw material that industry requires will be always available * Thanks to biotechnology, we can cultivate algae. – The way to cultivate an algae change a lot from one species to another, since their cultiva- tion depends on the life cycle of the algae, but in a generic way, it is possible to differentiate between support and intensive culture. During the support culture the growth of natural populations is favoured while the in- tensive one is the cultivation itself. 2 The intensive culture It can be done by imitating the life cycle of the algae or by taking advantage of the vegetative multiplication phases of the algae (a piece of an algae can give rise to a complete algae). – Image → Cultivation by vegetative multiplication. – In addition to this, the culture can be carried out in the medium (outdoor), in a production plant (indoor) or in a mixed culture, in which the seed preparation is done indoor in the laborat- ory, but the culture is done outdoors. – Figure → Culture outdoor of algae. – Figure → Culture indoor of algae. – The cultivation of algae in ponds, which yields formidable increases in productivity, opens im- portant prospects for this global industry, thanks to experiments carried out by North American and Japanese scientists. * Pond with no roof. – They managed to establish a farm where the common red algae (Chondrus crispus) can be produced in cultivation in quantities 60 times higher than those offered by its natural yield. 3 – For the cultivation of these algae, scientists recommend using pressed wood tanks covered with fiberglass or plastic, with a surface area of 3 m2. – 20 kilos of algae can be placed in each pond and after 30 days 36 can be collected, which yields a weight of 7.2 kilograms; Up to 10 harvests can be obtained each year, which is equal to 72 kilos of dry product. – Currently the main producers of red algae are Indonesia and the Philippines where algae grows on farms that yield more than 30 tons of dry product per hectare per year. In just 3 months a branch of 50 grams can reach up to 5 kilograms. 3. Macroalgae culture Fico-Hatchery → Requirements and Conditions for Cultivation (...): – Instrumental → To produce seedlings of macroalgae the phico-hatchery It must have a labor- atory with the following material resources: Closed space with controlled temperature. Constant availability of good quality UV sterilized filtered seawater. Fluorescent lighting system. Sea water treatment system (mechanical filters and ultraviolet sterilizer). Autoclave for the sterilization of smaller containers and culture media. Drying stove. Fine observation instruments (Microscope and Stereoscopic Loupe). – On the other hand, the laboratory must have the following conditions: Aseptic conditions and cleanliness of the place. Control of possible sources of contamination. Restricted access to the growing area. Washing area with facilities. 4 – Seawater pretreatment: Seawater constitutes the medium in which the early and juvenile life stages of the algae will develop, therefore, its quality is required to be optimal. Biological contamination (by bacteria, fungi or protozoa) or chemical contamination will greatly influence the development of the culture. – Water pretreatment: 1. Mechanical filtration through filters with pore sizes of 100, 50, 25 and 10 μm. 2. Filtration through cartridge filters 1 and 0.45 micron pore size. 3. Finally the water is exposed to UV radiation. – Sterilization of culture vessels: In the first stage of cultivation the containers must be sterile, therefore they must be auto- claved. Materials, both glass and plastic, must withstand the pressure and temperature condi- tions to which they are exposed inside the autoclave. Glassware (flasks, bottles, pipettes, etc.) of smaller volume, previously washed and rinsed, is wrapped and then placed in an autoclave. Subsequently, the material is removed from the autoclave and allowed to cool down to room temperature. 5 – Once this step has been carried out, the previously treated seawater (final filtered at 0.45 μm and irradiated with ultraviolet light) is added to each container and introduced to the autoclave: 121 ºC, 15 PSI pressure, 20 minutes. – The bottles are subsequently removed from the autoclave and stored in a dark chamber for 24 hours. The chamber has a 40W ultraviolet lamp which is activated for 15 minutes to irradiate the material prior to the addition of nutrients and inoculation. – Containers of greater volume, for example 20 liter bottles, are directly sterilized. (...) – Sporulation induction procedure: To induce sporulation, it is necessary to have mature reproductive biological material. Reproductive material is collected by diving and should immediately be stored under conditions of humidity, darkness and low temperature (