Applied Surface Science: Grafting Silazanes to Improve Vial Durability - PDF
Document Details
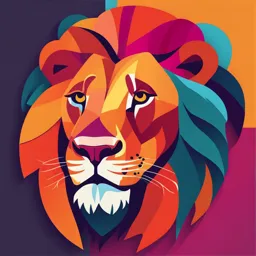
Uploaded by BetterThanExpectedVigor7993
Hangzhou Normal University
Ruolin Zhou
Tags
Summary
This document presents research on a method for modifying the inner surfaces of pharmaceutical glass vials using silazanes to enhance their chemical durability. The process involves grafting silazanes onto the glass surface, increasing the water contact angle and improving resistance to hydrolysis. Techniques used include colorimetric staining and hydrothermal aging tests with a goal to improving the barrier properties, and findings are discussed in detail.
Full Transcript
Applied Surface Science Highly efficient grafting of silazanes to the inner surface of the vials under mild conditions by surface engineering to improve the chemical durability --Manuscript Draft-- Manuscript Number: APS...
Applied Surface Science Highly efficient grafting of silazanes to the inner surface of the vials under mild conditions by surface engineering to improve the chemical durability --Manuscript Draft-- Manuscript Number: APSUSC-D-24-16348 Article Type: Full Length Article Keywords: Silazanes; Pharmaceutical Vials; Surface Modification; Hydrolytic resistance Abstract: As the composition of drugs has become more complex, the chemical durability of glass has been challenged. In this study, two silazanes, n-n-butyl-azido-2,2- dimethoxysilazane (BADMACP) and hexamethyldisilazane (HMDS) were grafted on the inner surface of the vials by O-Si bonds to form a functional hydrolysis-resistant surface with excellent stability. The success of the chemical modification was confirmed by colorimetric staining experiments, FT-IR, water contact angle (WCA) etc. The WCA was increased from unmodified 65° to more than 100°, and improved the hydrolytic resistance of the glass. Hydrothermal aging tests indicated that metal ion precipitation is greatly reduced, effectively reducing the risk of glass delamination. It is worth mentioning that the surface modification engineering of the inner surface of the vials with HMDS and BADMSCP can significantly improve the barrier properties of the vials without affecting the appearance and the transmittance of the vials. Powered by Editorial Manager® and ProduXion Manager® from Aries Systems Corporation Graphical Abstract (for review) Click here to access/download;Graphical Abstract (for review);ZRL-TOC.docx The following graphic will be used for TOC: After modifying by BADMACP and HMDS under mild and catalyst-free conditions, the inner surfaces of vial were transformed them from hydrophilic to hydrophobic, enhancing hydrolytic resistance while keeping light transmittance stable. Highlights (for review) Highlights The inner surface of vials was chemically grafted and modified by two silazanes through surface engineering in a catalyst-free, mild, efficient and robust manner. Following modification, the water contact angle on the inner surface of the vials was elevated from 65° to above 100°. As indicated by the hydrothermal aging test, the improvement in hydrolysis resistance is greater than 86% after modification. The surface engineering had no impact on the light transmittance of vials. Manuscript Click here to view linked References Highly efficient grafting of silazanes to the inner surface of the vials under mild conditions by surface engineering to improve the chemical durability Ruolin Zhou, Shengyao Tan, Fangrui Xie, Jing Dong, Jianping Zhu, Linxuan Fang* and Lianbin Wu* College of Material, Chemistry and Chemical Engineering, Key Laboratory of Organosilicon Chemistry and Material Technology, Ministry of Education, Key Laboratory of Organosilicon Material Technology, Hangzhou Normal University, Zhejiang, Hangzhou, 311121, Zhejiang, People’s Republic of China. CORRESPONDING AUTHOR FOOTNOTE Corresponding Author: *To whom correspondence should be addressed. Tel & Fax: +86 0571-28868905. *E-mail: [email protected] *E-mail: [email protected] ABSTRACT As the composition of drugs has become more complex, the chemical durability of glass has been challenged. In this study, two silazanes, n-n-butyl-azido-2,2-dimethoxysilazane (BADMACP) and hexamethyldisilazane (HMDS) were used separately to efficiently modify the inner surfaces of vials under mild conditions. These two silazanes were reacted with the -OH groups on the inner surface of the vials through Si-N bonds, and grafted by O-Si bonds to form a functional hydrolysis- resistant surface with excellent stability. The success of the chemical modification was confirmed by colorimetric staining experiments, Fourier transform infrared spectra, water contact angle and the morphology of the inner surface of the vials before and after modification. The water contact angle was increased from unmodified 65° to more than 100°, and improved the hydrolytic resistance of the glass by modifying the hydrophobicity of the inner surface of the glass vial. Hydrothermal aging tests were performed before and after modification defined by the pharmacopeias, and the volume of 0.01 M HCl consumed during titration decreased by 88% and 86%, indicating that metal ion precipitation is greatly reduced, effectively reducing the risk of glass delamination. It is worth mentioning that the surface modification engineering of the inner surface of the vials with HMDS and BADMSCP can significantly improve the barrier properties of the inner surface of the vials without affecting the appearance and the transmittance of the vials. Keywords: Silazanes, Pharmaceutical Vials, Surface modification, Hydrolytic resistance 1. Introduction Glass is widely recognized as the most commonly employed pharmaceutical packing material, primarily due to its inherent characteristics. These include high transparency, enabling clear visualization of the contents; high temperature resistance, which allows it to withstand various sterilization and storage conditions; high density, contributing to its robustness; excellent processability, facilitating manufacturing into diverse packaging forms; remarkable barrier properties against gases and liquids, safeguarding the integrity of the enclosed drugs; and, most crucially, a certain degree of chemical durability [1, 2]. Nevertheless, in recent years, with the growing complexity of drug compositions, the chemical durability of glass has encountered significant challenges [3, 4]. Many pharmaceutical agents intended for injection exist in the form of aqueous solutions. Hydrolytic resistance constitutes a crucial aspect of chemical resistance. There have been numerous instances where several pharmaceutical manufacturers have voluntarily recalled their drugs on account of glass delamination and other related issues. Glass delamination stands as the principal problem that impinges upon the chemical resistance of glass. There exist two primary erosion mechanisms of water on glass. Firstly, it encompasses the diffusion of water into the glass and the exchange of hydrogen ions with alkali metal (such as sodium or potassium) ions. This process is termed leaching. Secondly, it involves the dissolution of the silicate backbone of the glass (particularly the Si-O bond). When ion exchange and/or selective dissolution becomes the predominant mechanism, a leached layer will be formed. This leached layer can be easily separated and then enter the water. Furthermore, the exposure of Si-OH groups on the glass surface endows the glass surface with a high surface energy. This leads to the inner wall of the vial having a hydrophilic capacity. As a result, drugs adhere to the wall. Such adhesion gives rise to drug residue, which in turn impacts the utilization and quality of the drug reagent [8-11]. In an attempt to address the aforementioned issues, numerous reports have proposed various solutions. Bake-on siliconization represents a well-established method for coating the surface of pharmaceutical glass with a silicone oil coating. This silicone oil coating can not only transform the glass surface from hydrophilic to hydrophobic but also confer a certain degree of lubricating properties, which is of great significance for syringes. Moreover, bake-on siliconization enables the formation of hydrogen bonds between the glass surface and the silicone oil, endowing them with a certain level of chemical stability [13, 14]. Nonetheless, there is an inherent risk associated with the migration and shedding of silicone oils. Such migration and shedding can lead to contamination of the pharmaceutical agent. Additionally, the shed silicone oils may interact with proteins, thereby posing a risk of triggering unwanted immune responses [16, 17]. However, inducing cross-linking of the silicone oils on the glass surfaces through catalysis (for instance, by means of heat, plasma, or radiation) has the potential to further mitigate their interaction with the drug product. Even though cross-linked silicone holds the potential to enhance product quality, it has been emphasized that cross-linked silicone syringes remain suboptimal regarding the distribution of sub-visible particles of the drug product [18, 19]. Besides, the application of silicone oil, in recent years, a relatively novel and effective approach is to deposit a coating on the surface of pharmaceutical glass via chemical vapor deposition so as to improve the hydrolytic resistance of the glass. This process needs to be conducted in a specific CVD reactor, yet the required temperature is high, reaching 500 ℃ or even exceeding 600 ℃, and this method might impact the transparency of the glass vials [20, 21]. The existing methods mentioned above are beset with diverse issues like high energy consumption, influencing the appearance of the glass, and the risk of migration and peeling of the coating, among others. There is an urgent necessity for a mild and efficient method of glass surface modification that can enhance the effect of mitigating pharmaceutical glass packaging delamination on drug quality and reduce the surface energy of the glass, while also minimizing the additional impact of the modified layer on the drug. Surface engineering can be employed to complete the modification of the inner surface of vials simply and efficiently under mild conditions using Si-N bonds. Organosilicon reagents are typically characterized by excellent biocompatibility [22-24] and low surface energy [25-27]. Among them, silazanes are frequently used as hydrophobic modifiers due to the high reactivity of the Si-N bonds therein [28, 29]. In this study, BADMSCP and HMDS, two silazanes were selected for modifying the inner surface of the vials. The uniformity and completeness of the surface modification were initially judged by colorimetric staining experiments. The success of the surface modification was determined by the appearance of the C-H peak in the FTIR spectrometer and the change of the water contact angle from hydrophile to hydrophobicity. The stability of the modification was confirmed by the comparison of the contact angle of the glass vials fragments before and after extraction, indicating that it is not easily dislodged. The transparency of the vials before and after modification was measured by UV-vis NIR. Most importantly, the water-resistance test for the inner surface of the vials was conducted in accordance with the pharmacopoeia [30, 31]. Significantly, the hydrolytic resistance of the inner surface of the vials was tested under high temperature and pressure according to the pharmacopoeia. The morphology of the inner surface of the modified and unmodified vials before and after the hydrolytic resistance test was observed by SEM. The aim of this work is to demonstrate the feasibility of modifying the inner surface of vials with small molecule silazanes to improve the hydrophobicity of the surface and the hydrolytic resistance. 2. Materials and methods 2.1. Materials Twenty-milliliter vials were procured from Schott. The spray gun was purchased from Pressey. Ethanol, toluene, dichloromethane, methyl red, sodium hydroxide, hydrochloric acid, hydrogen peroxide, sulfuric acid, hexamethyldisilazane (HMDS), and hexamethyldisiloxane (MM) were furnished by Sinopharm Chemical Reagent Co., Ltd. (Shanghai, China). N-n-butyl-aza-2,2- dimethoxysilazane (BADMSCP) was supplied by Shanghai Myriad Chemical Technology Co., Ltd. (Shanghai, China). Methylene Blue (MBB) was provided by Beijing Huawei Ruike Chemical Technology Co. Ultrapure water (UPW) was generated by the Ultrapure Water Tank (UPTA-10, Shanghai Li-Chen Bang Xi Instrument Technology Co., Ltd, Shanghai, China). The UV equipment was offered by Hitech Ned Limited (UV-2LD, Shenzhen, China). All the reagents were of analytical purity and were utilized directly without undergoing further purification. The piranha solution was prepared by mixing concentrated sulfuric acid and 30% hydrogen peroxide in a 7:3 ratio. 2.2. Modification of vials with silazanes by surface engineering The process and mechanism of BADMSCP and HMDS modifying the inner surface of vials through surface engineering are depicted in Scheme 1. Prior to modifying pharmaceutical glass vials, the inner surface of the vials is etched with piranha solution to eliminate impurities from the glass surface and enhance the -OH content on the surface. This enables the silazanes reagent to interact more fully with the glass surface. The silazane solution is evenly sprayed onto the inside of the vial using a spray gun. For each 20 mL vial, 200 μL of silazane solution is sprayed. The vial is then plugged with a rubber stopper, and a needle is inserted to equalize the internal and external air pressure. Subsequently, it is placed in an environment with different temperatures to react for a specific duration. The specific reaction conditions are presented in Table 1. At the end of the reaction, the vials are sequentially cleaned with toluene, ethanol and ultrapure water (UPW) for 5 min each through ultrasonic cleaning to remove impurities such as unreacted solution that may remain on the vials surface. Thereafter, the vials are placed in an oven at 120 ℃ for 30 min to dry. Once cooled down to room temperature, the vials modified by the silazanes on the surface are obtained. Table 1 Summary of conditions for modifying the inner surface of vials with BADMSCP and HMDS. silazane condition BADMSCP HMDS temperature /℃ 25 120, 150, 180 time/min 120 40, 80, 120, 180 solvent MM MM ratio to solvent 1:5, 2:5, 3:5, 4:5, 5:5 100% (Vsilazane :Vsolvent) spraying volume/μL 200 200 Scheme 1. Schematic diagram of the modified vial inner surface with silazanes. 2.3. Characterization 2.3.1 Colorimetric staining Colorimetric staining experiments serve as the initial analytical tool utilized to determine the efficacy of grafting with silazanes and enable visualization of the homogeneity of grafting through staining [32, 33]. The entire vials is filled with a 0.05 wt% methylene blue solution. After 30 min, it is ultrasonically cleaned with ultrapure water for 5 min to wash away the floating color. Upon drying, the staining of the top, body and bottom of the vials is observed. Changes in the staining situation can initially be used to judge whether the surface modification is successful, as well as the uniformity and completeness of the surface modification. Colorless staining indicates that the ion exchange between methylene blue and glass is weak. 2.3.2 Hydrothermal aging tests In accordance with the guidelines stipulated by the United States Pharmacopeia (USP) and the European Pharmacopeia, the internal surface of the vial undergoes hydrothermal ageing and hydrolytic resistance tests. Hydrothermal aging of each vial is conducted in a separate hydrothermal reactor. The heating and natural cooling process is carried out in an oven. The combination of these two can replace the role of autoclaves in testing. The specific operation diagram is shown in Scheme. S1. During the process, strict attention should be paid to the consistency and precision of the length of rinsing, the fill volume of ultrapure water in the vials, and the warming process. The vials are subjected to an ultrasonic cleaning process with ultrapure water for 5 min and then drained. Subsequently, 22 mL of ultra-pure water (90% of the vial volume) is added to each cleaned vial. The vial is transferred to a polytetrafluoroethylene container containing 30 mL of ultra-pure water, and the hydrothermal reactor is assembled. The hydrothermal reactor is then transferred to an oven at 100 ℃. During the transfer, the oven temperature drops slightly. When the oven temperature rises to 100 ℃, it is set to 121 ℃. This temperature setting process ensures that the temperature of the reactor will not rise too quickly, thus ensuring the safety of the experiment. When the oven temperature rises to 121 ℃, autoclaving is carried out for 1 h. Subsequently, the oven switch is turned off and it is cooled naturally for 12 h to ensure that the hydrothermal reactor can be opened safely. During this process, the vials are heated and pressurized for a much longer time than recommended by the pharmacopoeia. Afterwards, the reactor is opened, the vial is removed, and then the pH of the water in the vial is determined by a pH meter, which provides a quick way to know the change in pH. Additionally, titration with 0.01mol/L HCl using methyl red as a color developer is carried out according to the test method recommended by the Pharmacopoeia. 2.3.3 Characterization Methods for Surface and Structural Properties The vials were carefully broken to select flat bottom fragments which were then washed with ethanol to remove any potential debris or dust. Fourier transform infrared, water contact angle tests and scanning electron microscope characterization were performed on the inner surface side of the fragments. Fourier transform infrared (FT-IR) spectra were recorded using a spectrometer (Antaris, Nicolet 7000, Thermo Fisher, Waltham, MA, USA) in the range of 4000 to 400 cm-1. The water contact angle (WCA) of the vials was measured by using the DSA30 video optical contact angle analyzer (KRUSS, Hamburg, Germany), and the results are reported as the average value from three different sites on the inner surface of the vial fragments. A ZEISS ultra plus field emission scanning electron microscope (FE-SEM, ZEISS, sigma500, Oberkochen, Germany) was employed to examine the morphological changes of the inner surface of the vials before and after modification, as well as before and after hydrothermal aging tests, including the sidewall cross-section morphology. 3. Results and discussion 3.1. Properties of the Inner surface of vials In this study, a cyclic silazane BADMSCP and a linear silazane HMDS were selected for surface modification of the inner surface of vials respectively. The resulting hydrolysis-resistant functional surfaces were successfully formed on the interior surface of the vials. The optimal reaction conditions were identified through the design of experiments involving different reaction temperatures, reaction durations, and ratios with solvents. Additionally, the quality of the modified vials was evaluated by various methods. Firstly, the vials modified with BADMSCP and HMDS were stained with methylene blue solution and compared with the unmodified vials. The blue color indicates that the glass surface has been corroded through ion exchange with the methylene blue solution. The darker the color, the more severe the corrosion. Five different ratios of BADMSCP and MM (1:5, 2:5, 3:5, 4:5, 5:5) were studied, respectively named B-1, B-2, B-3, B-4, and B-5. Fig. 1a displays photographs of the vials obtained after staining by directly modifying the inner wall with different BADMSCP:MM ratios without the activation treatment with piranha solution. It can be seen that the staining becomes lighter as the ratio of BADMSCP increases, but the blue color can still be clearly observed. In contrast, after etching with piranha solution followed by surface modification, it can be found that the staining has become almost unobservable with the increase in the concentration of BADMSCP (Fig. 1b), demonstrating the fact that the increase in the hydroxyl content on the inner surface of the glass vials enables the silane reagent to modify the glass surface more adequately, thereby improving the integrity of the surface modification. Therefore, all subsequent modifications were performed after the vials had been etched with the piranha solution. It was observed that BADMSCP can efficiently complete the modification process at room temperature without a catalyst. BADMACP is a five-membered heterocyclic compound featuring a silicon-nitrogen (Si-N) moiety. It undergoes a facile ring-opening reaction to modify hydroxyl groups present on the glass surface. This method is termed a "ring-opening click" reaction due to its simplicity, high yield, wide applicability, absence of by-products and the utilization of easily removable solvents. Additionally, -OMe in the BADMSCP structure is readily hydrolyzed to -OH, which further reacts with the -OH on the glass surface, leading to stronger bonding of the modified reagent to the glass surface. Higher temperatures are required for modification using HMDS in the absence of a catalyst. To investigate the effect of HMDS on the modification of the inner surface of vials, a series of reaction temperatures and durations were explored. For a range of temperatures (120℃, 150℃, 180℃) and reaction times (40 min, 80 min, 120 min), it was observed that the staining phenomenon decreased significantly with increasing temperature and reaction time. Almost no staining could be observed in vials modified with HMDS at 180℃ for 80 min (Fig. 1e). HMDS is a commonly used surface modifier, often reported to be employed in the surface modification of silica. It reacts with the -OH groups on the surface, enabling the highly reactive -Si (CH3)3 on HMDS to be bonded onto the surface of SiO2. This can transform the surface of silica from hydrophilic to hydrophobic [36- 38], thereby improving its dispersion and compatibility in the polymer matrix. The Si-OH on the glass surface is similar to that on the surface of silica, which is highly informative. Although the activity of HMDS is not as reactive as that of BADMSCP with Si-OH, it has the advantage of low cost. This surface modification engineering can be conducted in an efficient manner without the necessity for catalysts and without the necessity for higher temperatures, which greatly simplifies the conditions required for the reaction. This approach has the potential to maximise cost savings and reduce the possibility of contamination. Fig. 1. The pictures of vials after staining with methylene blue solution. (a) Vials directly surface- modified with BADMSCP; Vials activated by Piranha solution to activate the inner surface of the vials and then modified with (b) BADMSCP and HMDS at (c) 120 ℃, (d) 150 ℃ and (e) 180 ℃. The changes in chemical bonding on the surface of the vials before and after the silanisation treatment were explored by FT-IR in Fig. 2a. It’s noted that vials after silanisation display the appearance of a new characteristic peak at around 1460 cm-1, which is the bending vibration of C- H, and a peak at 3000 cm-1 which corresponds to the stretching vibration of C-H. The presence of C-H characteristic peak in the FT-IR characterization further substantiated that BADMSCP and HMDS were successfully grafted onto the surface of the vials via the surface engineering. Therefore, hydrophobic functional groups can be grafted onto the glass surface through this surface modification engineering to improve the hydrophobicity of the glass surface and improve the hydrolytic resistance. In addition, the modified glass vials showed no other obvious new characteristic peaks, it might be due to the fact that the modified layer is a single molecular layer, and it is difficult for FT-IR to characterize other molecular structure changes on the glass surface. Moreover, it can be observed with Fig. S2a and UV-vis-NIR transmittance test in Fig. 2b that after the internal surface of the vials is modified with two silazane reagents through surface engineering, the transparency and light transmittance of both vials have not changed significantly. Fig. 2. (a) FTIR and (b) UV-vis-NIR spectra of blank, B-1, B-2, B-3, B-4, B-5 and HMDS. The inner surface of the blank vials exhibits hydrophilic properties, with a water contact angle of approximately 65°. After being etched by the piranha solution, more hydroxyl groups will be present on the inner surface, which further decreases the water contact angle to around 47°. Meanwhile, the solvent MM remains inert throughout this modification process and can thus offer a stable medium environment for the reaction. And then, after the Surface engineering treatment with two kinds of silazanes, the contact angle of the inner surface changes from hydrophilic to hydrophobic (Fig. 3), which is due to the conversion of hydrophilic Si-OH on the glass surface to hydrophobic groups, hydrophobic angle test diagram is shown in Fig. S1. At room temperature, the water contact angle can be stabilized above 100° at the ratio of BADMSCP to MM of 5:5. The surface-modified vials were extracted with a good solvent n-heptane under magnetic stirring for 2 h. For HMDS, the reaction temperature and duration on the inner surface of the vials exert a significant influence on the water contact angle. But the effect of temperature will be much greater than the effect of time after 80 min, and the water contact angle cannot stabilize above 100° after treating at 120 ℃ and 150℃ for 3 h, respectively. When the reaction temperature reaches 180 ℃, the water contact angle can be stabilized above 100° after the temperature duration exceeds 80min, and it shows a slight upward trend. Because the energy of Si-N bond in HMDS is slightly stronger, a certain temperature is required to promote the reaction. The coating grafted on by the silazanes would not be detached by the organic solvent extraction, and still maintained the original water contact angle. In other reports on the treatment of vials with silicone, such as with silicone oil, the silicone oil is detached by the extraction of organic solvents such as n-heptane. Hence, we are able to claim that the silazanes used in this paper is chemically grafted to the glass surface, creating a strong chemical bond with relatively high chemical stability. Fig. 3. Water contact angles at the bottom of the inner surface of vials (a) modified by different ratio of BADMSCP and MM solutions and (b) modified by HMDS at different temperatures and times. The micro-morphology of the surface of the vials treated with the two silanising reagents was observed by FE-SEM. As in Fig. 4, the surfaces of the vials before and after modification are both relatively flat with no obvious difference, until the surface was enlarged to the point that the grain of sprayed Au could be seen, and it could still be seen that the surfaces of the vial fragments are homogeneous and flat. In comparing the cross-sections of fragmented vials post-modification with those pre-modification, it is exceedingly difficult to observe a clearly distinguishable modified layer from the glass surface. The results demonstrate that the modification of the inner wall of the vials by the two silazanes described in this paper did not result in any notable alterations in the appearance of the vials, whether macroscopic or microscopic.This is attributed to the fact that this surface modification engineering solely modifies the chemical composition of the glass surface, endowing it with hydrophobic properties while not significantly altering the overall nature of the glass. Fig. 4. FE-SEM images of the internal surface fragments of blank vials, vials modified by BADMSCP, HMDS and after the 121℃ hydrolytic resistance tests (Blank 121, BADMSCP121 and HMDS 121). 3.2 Hydrolytic resistance Visual observation of the water contained in the vials after the hydrolytic resistance experiments revealed no difference in appearance compared to that before the test. In particular, there were no visible flakes and the liquid in the vials remained pure and clear, as shown in Fig. S3. The pH value of the ultrapure water used in the experiment was tested to be 7.0 - 7.2. However, the pH value of the water in the vials without surface modification for the hydrolytic resistance test increased to 8.37 due to the release of metal ions from the ion exchange between the water and the glass surface. As depicted in Fig. 5, the pH value of the vials modified with BADMSCP decreased significantly with the increase in the concentration of BADMSCP, indicating that the hydrolytic resistance of the vials would be improved by the silazanes. Particularly for sample B-5, the elevation of its pH value is extremely minimal. In fact, it is nearly indistinguishable from the pH value of high-purity water per se. When modified with HMDS, it was observed that the hydrolytic resistance gradually increased with the extension of the reaction time and the increase of the reaction temperature. The hydrolytic resistance reached the optimum at a temperature of 180 ℃ and a time of 120 min or more. Aside from pH determination, the aqueous solution in the vials that have undergone hydrothermal aging testing was titrated with 0.01 M HCl titrant in accordance with the pharmacopoeia. When titrating the aqueous solution subjected to hydrothermal aging in the unmodified vials, the consumption of 0.01 M hydrochloric acid titrant was 1.55 mL per 100 ml. The volume of 0.01 M HCl consumed by the B-5 vials was 0.19 mL per 100 ml, representing an 88% decrease in HCl volume. The volume of HCl consumed by the vials treated with 200 μL of HMDS for 3 h at 180 ℃ decreased to 0.21 mL, which corresponded to an 86% decrease. In comparison to other reports, such as Pierre-Luc et al. who employed an amorphous alumina coating deposited on the surface of pharmaceutical glass, the optimal performance was observed for films with intermediate thicknesses between 250 and 550 nm, consuming a volume of HCl close to 0.1 mL, which represented an 80% reduction compared to the type I upper limit of 0.5 mL. In this experiment, the consumption of hydrochloric acid in unmodified vials is much greater than the upper limit of type I reported in the literature. This is because, to ensure the safe use of the hydrothermal reactor, natural cooling and natural pressure reduction are adopted for the hydrothermal reaction kettle after the hydrothermal aging test in this paper. With a cooling time of 12 hours, the heating and pressurization time is significantly extended, resulting in an increase in the volume of consumption of 0.01 M HCl. Compared with traditional pharmacopoeia, this is a more stringent water resistance test that imposes more aggressive testing conditions on vials. Consequently, employing the percentage reduction of the volume consumption of HCl titrant as the comparison parameter is more scientifically sound. FE-SEM photographs of the vials were also taken that had undergone the hydrolytic resistance test. It could be observed that the surface of the glass that had not undergone surface modification would have large water-stained traces due to hydrothermal aging after the hydrolytic resistance test. Some parts of the glass would also have breakage and surface detachment, indicating that the phenomenon of severe glass corrosion had occurred. However, in the cross-sectional FE-SEM images of the fragments of vials whose inner surfaces had been modified by the silazane surface engineering, no similar phenomena were observed. This further verified that this surface engineering can significantly enhance the hydrolytic resistance of the inner surfaces of the vials. When the contact angle is relatively low, the glass surface exhibits good wettability but poor water resistance, making it susceptible to water-induced damage, such as surface blurring or the formation of small pits. However, when the contact angle is increased to a higher value through treatment. For instance, in this study, surface engineering using silazanes is employed to raise the contact angle of the vial's interior to 100° or above. At this point, the glass surface exhibits strong hydrophobicity. It shields the inner surface of the vial in a manner analogous to an umbrella, the mechanism schematic diagram is shown in Scheme 2. In the same aqueous environment, the glass maintains its chemical integrity and shows significantly improved water resistance. This enables it to withstand prolonged immersion or repeated rinsing with water. Surface engineering replaces the -OH groups on the glass surface with organic hydrophobic groups, such as -(CH3)3, effectively shielding water diffusion into the glass and preventing ion exchange between H+ and metal ions such as Ca2+, Na+, Mg2+, and K+ in the glass's inner layers. Simultaneously, this process inhibits the external water from damaging the Si-O-Si backbone of the glass, preventing selective dissolution and significantly improving the hydrolytic resistance of the vial’s interior surface. Therefore, the hydrolytic resistance of the vials has been remarkably enhanced through this surface engineering modification. Fig. 5. pH changes of vials modified with (a) different ratios of BADMSCP and MM, (b) HMDS under different reaction temperatures and times after hydrothermal aging experiment. Scheme 2. Schematic diagram of hydrolytic resistance of the inner surface of the vial after surface modification. 4. Conclusion In summary, two silazanes (BADMSCP and HDMS) were successfully employed to modify the inner surface of pharmaceutical glass vials under mild and catalyst-free conditions. After modification, the hydrophobicity of the inner surface of vials can be significantly improved with the water contact angle increasing from 65° to 101°. It also showed good hydrolytic resistance in the hydrothermal aging test. SEM analysis revealed that the inner surface of the vials remained flat after the hydrothermal aging test, with no evidence of glass delamination or dehiscence in contrast to unmodified vials. It is worth mentioning that the modification engineering of the inner surface of the vials with HMDS and BADMSCP can significantly improve the barrier properties of the inner surface of the vials without affecting the appearance of the vials. References E. Guadagnino, M. Guglielmi, F. Nicoletti, Glass: The best material for pharmaceutical packaging, Int. J. Appl. Glass Sci, 13 (2022) 281-291. R.A. Schaut, W.P. Weeks, Historical review of glasses used for parenteral packaging, PDA J. Pharm. Sci. Technol., 71 (2017) 279-296. R.G. Iacocca, M. Allgeier, Corrosive attack of glass by a pharmaceutical compound, J. Mater. Sci., 42 (2007) 801-811. D. Jenke, J. Castner, T. Egert, T. Feinberg, A. Hendricker, C. Houston, D.G. Hunt, M. Lynch, A. Shaw, K. Nicholas, D.L. Norwood, D. Paskiet, M. Ruberto, E.J. Smith, F. Holcomb, Extractables characterization for five materials of construction representative of packaging systems used for parenteral and ophthalmic drug products, PDA J. Pharm. Sci. Technol., 67 (2013) 448-511. G. Kabirdas B, S.J.I.J.o.P.S. Sharda M, D. Research, Glass delamination in sterile formulations and Drug Recalls: Int. J. Pharm. Sci. Dev. Res., 8 (2022) 1−5. N. Zadbuke, S. Shahi, B. Gulecha, A. Padalkar, M. Thube, Recent trends and future of pharmaceutical packaging technology, J. Pharm. Bioallied Sci., 5 (2013) 98-110. Panighello S, Pinato O. Investigating the effects of the chemical composition on glass corrosion: a case study for type I vials. PDA J. Pharm. Sci. Technol., 74 (2020) 185-200. E. Chan, A. Hubbard, S. Sane, Y.F. Maa, Syringe siliconization process investigation and optimization, PDA J. Pharm. Sci. Technol., 66 (2012) 136-150. N.P. Mellott, C.G. Pantano, A mechanism of corrosion-induced roughening of glass surfaces, Int. J. Appl. Glass Sci, 4 (2013) 274-279. U. Rothaar, M. Klause, B. Hladik, Comparative delamination study to demonstrate the impact of container quality and nature of buffer system, PDA J. Pharm. Sci. Technol., 70 (2016) 560-567. S.h. Gin, J.M. Delaye, F. Angeli, S.J.n.M.D. Schuller, Aqueous alteration of silicate glass: state of knowledge and perspectives, NPJ Mater. Degrad., 5 (2021) 1-20. J. Zhao, V. Lavalley, P. Mangiagalli, J.M. Wright, T.E. Bankston, Glass delamination: a comparison of the inner surface performance of vials and pre-filled syringes, AAPS PharmSciTech, 15 (2014) 1398- 1409. S. Funke, J. Matilainen, H. Nalenz, K. Bechtold-Peters, H.-C. Mahler, F. Vetter, C. Müller, F. Bracher, W. Friess, Optimization of the bake-on siliconization of cartridges. Part II: Investigations into burn-in time and temperature, Eur. J. Pharm. Biopharm., 105 (2016) 209-222. S. Funke, J. Matilainen, H. Nalenz, K. Bechtold-Peters, H.-C. Mahler, W. Friess, Optimization of the bake-on siliconization of cartridges. Part I: Optimization of the spray-on parameters, Eur. J. Pharm. Biopharm., 104 (2016) 200-215. S. Funke, J. Matilainen, H. Nalenz, K. Bechtold-Peters, H.C. Mahler, W. Friess, Silicone migration from baked-on silicone layers. Particle Characterization in Placebo and Protein Solutions, J. Pharmacol. Sci., 105 (2016) 3520-3531. C.F. Chisholm, K.R. Soucie, J.S. Song, P. Strauch, R.M. Torres, J.F. Carpenter, J.A. Ragheb, T.W. Randolph, Immunogenicity of structurally perturbed hen egg lysozyme adsorbed to silicone oil microdroplets in wild-type and transgenic mouse models, J. Pharmacol. Sci., 106 (2017) 1519-1527. G.B. Melo, N. Cruz, G.G. Emerson, F.A. Rezende, C.H. Meyer, S. Uchiyama, J. Carpenter, H.F. Shiroma, M.E. Farah, M. Maia, E.B. Rodrigues, Critical analysis of techniques and materials used in devices, syringes, and needles used for intravitreal injections, Prog. Retin. Eye Res., 80 (2021) 100862. V. Thakare, T. Schmidt, O. Rupprechter, J. Leibold, S. Stemmer, A. Mischo, D. Bhattacharjee, P. Prazeller, Can cross-linked siliconized PFS come to the rescue of the biologics drug product?, J. Pharmacol. Sci., 109 (2020) 3340-3351. R.A. Depaz, T. Chevolleau, S. Jouffray, R. Narwal, M.N. Dimitrova, Cross-linked silicone coating: a novel prefilled syringe technology that reduces subvisible particles and maintains compatibility with biologics, J. Pharmacol. Sci., 103 (2014) 1384-1393. K.C. Topka, B. Diallo, M. Puyo, E. Chesneau, F. Inoubli, S. Ponton, C. Genevois, D. Samelor, R. Laloo, D. Sadowski, C. Charvillat, T. Teramoto, F. Senocq, T. Sauvage, H. Vergnes, M.-J. Menu, C. Dussarrat, B. Caussat, V. Turq, C. Vahlas, N. Pellerin, Silicon oxynitride coatings are very promising for inert and durable pharmaceutical glass vials, ACS Appl. Eng. Mater., 1 (2023) 3268-3283. P.-L. Etchepare, D. Samelor, H. Vergnes, B. Caussat, C. Vahlas, Barrier properties and hydrothermal aging of amorphous alumina coatings applied on pharmaceutical vials, Surf. Coat. Technol., 425 (2021) 127711. M. Razavi, R. Primavera, A. Vykunta, A.S. Thakor, Silicone-based bioscaffolds for cellular therapies, Mater. Sci. Eng., C, 119 (2021) 111615. M. Zare, E.R. Ghomi, P.D. Venkatraman, S. Ramakrishna, Silicone-based biomaterials for biomedical applications: Antimicrobial strategies and 3D printing technologies, J. Appl. Polym. Sci, 138 (2021) 50969. T. Mladenovic, F. Zivic, N. Petrovic, S. Njezic, J. Pavic, N. Kotorcevic, S. Milenkovic, N. Grujovic, Application of silicone in ophthalmology: a review. NPJ Mater. Degrad., 17 (2024) 3454. L. Zhang, A.G. Zhou, B.R. Sun, K.S. Chen, H.Z.J.N.C. Yu, Functional and versatile superhydrophobic coatings via stoichiometric silanization, Nat. Commun., 12 (2021) 982. J. Li, M. Du, Y. Cheng, S. Wang, J. Chen, S. Hu, H. Zhang, H. Zhang, Condensation and hydrophobicity of the surface hydroxyl groups between organosilane modified water glass and microsilica, Ceram. Int., 49 (2023) 21278-21286. M. Zhou, X. Liu, F. Xu, Y. Pei, L. Wu, L.-C. Tang, Facile UV-induced surface covalent modification to fabricate durable superhydrophobic fabric for efficient oil–water separation, Polymers, 15 (2023) 2505. D. Jeong, D.-G. Kim, I.H. Do, H. Lee, Hydrophobic passivation of ultra-high-Q silica wedge resonators using hexamethyldisilazane, Opt. Lett., 46 (2021) 2019-2022. Y. Zhan, R. Grottenmüller, W. Li, F. Javaid, R. Riedel, Evaluation of mechanical properties and hydrophobicity of room-temperature, moisture-curable polysilazane coatings, J. Appl. Polym. Sci., 138 (2021) 50469. C.o. Europe, Ph. Eur, C.o. Europe (Ed.), Counsil of Europe, Strasbourg, 2010, 363–367. D. Hunt, General Chapter Evaluation of the Inner Surface Durability of Glass Containers, USP-NF, 2012. D. Ditter, A. Nieto, H.-C. Mahler, H. Roehl, M. Wahl, J. Huwyler, A. Allmendinger, Evaluation of glass delamination risk in pharmaceutical 10 mL/10R vials, J. Pharmacol. Sci., 107 (2018) 624-637. D. Ditter, H.C. Mahler, H. Roehl, M. Wahl, J. Huwyler, A. Nieto, A. Allmendinger, Characterization of surface properties of glass vials used as primary packaging material for parenterals, Eur. J. Pharm. Biopharm., 125 (2018) 58-67. C. Sloey, C. Gleason, M. Akers, Y. Nashed-Samuel, J. Phillips, An evaluation of the glass vial hydrolytic resistance method, PDA J. Pharm. Sci. Technol., 73 (2019) 212-219. D. Kim, J.M. Zuidema, J. Kang, Y. Pan, L. Wu, D. Warther, B. Arkles, M.J. Sailor, Facile surface modification of hydroxylated silicon nanostructures using heterocyclic silanes, J. Am. Chem. Soc., 138 (2016) 15106-15109. B. Lin, S. Cui, X.-y. Liu, X.-d. Shen, Y. Liu, G.J.C.N. Han, Preparation and characterization of HMDS modified hydrophobic silica aerogel, Solid State Sci., 7 (2011) 1042-1045. X. Wang, H. Zhao, Y. Su, C. Zhang, C. Feng, Q. Liu, J. Shen, Low-temperature preparation of mechanically robust and contamination-resistant antireflective coatings for flexible polymeric glasses via embedding of silica nanoparticles and HMDS modification, ACS Appl. Mater. Inter., 11 (2019) 37084-37093. C.C. Chang, Y.-T. Wu, L.P.J.J.o.C.T. Cheng, Research, Preparation of HMDS-modified silica/polyacrylate hydrophobic hard coatings on PMMA substrates, J. Coat. Technol. Res., 13 (2016) 999-1007. T. Vukovic, J. Røstad, U. Farooq, O. Torsæter, A. van der Net, Systematic study of wettability alteration of glass surfaces by dichlorooctamethyltetrasiloxane silanization─a guide for contact angle modification, ACS Omega, 8 (2023) 36662-36676. Supplementary Material for on-line publication only Click here to access/download Supplementary Material for on-line publication only ZRL-Supporting.docx