Appunti Albanese.PDF
Document Details
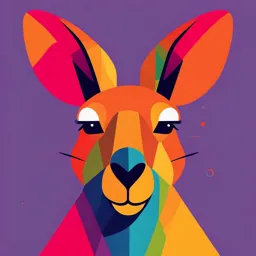
Uploaded by SmittenGradient
Tags
Full Transcript
Thermal Heavy molecules are Catalytic broken down into HYDROCARBON CRACKING CONVERSION...
Thermal Heavy molecules are Catalytic broken down into HYDROCARBON CRACKING CONVERSION lower MW Hydrocracking PROCESSES hydrocarbons REFORMING and Structural modification without Steam ISOMERIZATION size variation Cracking INCREASING Polymerization or SIZE alkylation CRACKING Cracking is a process whereby heavy molecules contained in crude oil are BROKEN down, with HOMOLITIC OR HETEROLITIC DISSOCIATIONS, into hydrocarbons of lower molecular weight. THERMODYNAMIC From a thermodynamical point of view, cracking is an ENDOTHERMIC PROCESS ( there are more broken bonds are more than newly formed bonds), so HEAT SHOULD BE PROVIDED as efficiently as possible to remain around temperatures T > 300-350 °C in order to obtain acceptable reaction rates. It’s known that THERMODYNAMIC STABILITY DECREASES WHEN THE MOLECULAR WEIGHT INCREASES, as shown in the FRANCIS DIAGRAM. This diagram reports the HYDROCARBONS FREE ENERGY OF FORMATION along with the TEMPERATURE. It considers the thermal treatment of the heavier residues in order to obtain lighter fractions (higher quality). As highlighted in the diagram, the most interesting compounds are PARAFFINS, which lines have a stiffer slope than those representing olefins. Due to this, the T REQUIRED to convert PARAFFINS INTO At a T higher or lower than 880 K, only OLEFINS is LOWER than the one required for the OPPOSITE a single product is favoured, the one with ΔG° lower than zero.+ REACTION. Since thermodynamic plays an important part in thermal cracking, the latter is based on the use of Francis diagram. Let’s consider the decomposition reaction of a butane molecule: Free energies are divided by 4 since the 𝐶𝐻3 𝐶𝐻2 𝐶𝐻2 𝐶𝐻3 → 2𝐶𝐻2 = 𝐶𝐻2 + 𝐻2 diagram depicts them in kcal/mol of carbon. This reaction can be written as the sum of ΔG°R= 0 when both terms of the two semi-reactions: abovementioned relation are equal. This happens at the intersection of the two 𝐶𝐻3 𝐶𝐻2 𝐶𝐻2 𝐶𝐻3 → 4𝐶 + 5𝐻2 sloped lines, at a temperature value of 880 K 4𝐶+5𝐻2 → 2𝐶𝐻2 = 𝐶𝐻2 + 𝐻2 (607 °C) → both products are favourite Gibbs free partial molar energies are: At a higher temperature: 0 0 ∆𝐺𝑅0 = ∆𝐺10 + ∆𝐺20 ∆𝐺𝑓,𝑏𝑢𝑡𝑎𝑛𝑒 ∆𝐺𝑓,𝑒𝑡ℎ𝑖𝑙𝑒𝑛𝑒 ∆𝐺𝑅0 > ⇒ 0 4 4 2 4 2 4 Moreover: 1. If two lines are PARALLEL, the transformation reaction of a product into another one is ALWAYS FAVOURED IN A SPECIFIC DIRECTION. 2. Hydrocarbons stability is lower than C and H stability. By LENGTHENING cracking reaction TIME, a PYROLYSIS reaction is obtained. 3. SATURATED HYDROCARBONS depict NEARLY PARALLEL lines among them. 4. ACETYLENE has a NEGATIVE slope. The higher the T, the higher its stability. 5. The HIGHER the MOLECULAR WEIGHT, the LOWER the thermodynamic STABILITY. 6. COKE FORMATION is NOT AVOIDABLE for thermodynamic reasons and its production surely leads to reactor clogging and catalyst poisoning. For these reasons, reactors shall be periodically cleaned, and the catalyst must be regenerated by burning coke deposits. THERMAL CRACKING The thermal cracking has been the FIRST process to be used in 1913. Hydrocarbon C–C bond cleavage was carried out at 450-500 °C, generating gas, gasoline, gasoil and a huge amount of heavier products WITHOUT HIGH VALUE. In fact, thermal processes have been replaced by catalytic, affording MORE VALUABLE GASOLINE, with higher OCTANE NUMBER, a parameter that indicates the resistance to detonation of gasoline (ONStandard = 98). Nowadays, thermal processes are still used in refinery only for particular applications, for example, the TRANSFORMATION OF PARAFFINS INTO OLEFINS at HIGH TEMPERATURE, around 800 °C. Usually, the ADDITION OF INERT STEAM is done in order to DECREASE HYDROCARBONS PARTIAL PRESSURE, to work as SOURCE OF THE HEAT required and to REMOVE SOME COKE through the GASIFICATION reaction (C + H2O → CO + H2), increasing the amount of LOWER OLEFINS. RADICAL MECHANISM When hydrocarbons are heated at HIGH T WITHOUT CATALYSTS, HOMOLITIC BOND CLEAVAGE occurs, and a RADICAL MECHANISM starts. The major difference between a radical and cation is that there is no isomerization but there is an electron extraction. The first homolysis of C–C bond is followed by the formation of ETHYLENE AND A PRIMARY RADICAL, according to the EMPIRIC RULE β which states that the bond cleavage happens in β position from the carbon atom with the unpaired electron. The new radical makes β cleavage until a METHYL RADICAL is formed (.CH3). The latter EXTRACTS A H radical from another hydrocarbon molecule, forming CH4 and a MORE STABLE SECONDARY RADICAL. The repetition of these steps leads to the formation of huge amounts of ETHYLENE, a small amount of METHANE and α-OLEFINS. PRIMARY RADICALS can also extract, as well as methyl radical, a H radical from another paraffin. However, this rarely occurs, as showed by LOW AMOUNT OF PARAFFINS. RADICAL ISOMERIZATION does not occur, but a long chain primary radical can fold and extract a H radical from a SECONDARY OR TERTIARY POSITION. This causes a reduction of ethylene and increase of GASOLINE. INFLUENCE OF PROCESS VARIABLES Temperature Pressure Time Reaction rate varies Rate increases with P Contact time according to until 300-400 atm, INCREASES the Arrhenius equation THEN SLOWLY percentage of k = Ae-Ea/RT DECREASES SECONDARY So for a ΔT of 10-20 °C For the generation of OLIGOMERIZATION at 400-600°C, the SMALL MOLECULES is PRODUCTS and COKE velocity doubled favored LOW PRESSION FORMATION At T HIGHER than Not easy to measure 400°C the CRACKING in thermal cracking is favored at CHAIN since it's DIFFICULT END TO EVALUATE THE REACTOR VOLUME CATALYTIC CRACKING It’s a GAS PHASE PROCESS catalyzed by an ACIDIC COMPOUND able to INCREASE THE RATE AND THE SELECTIVITY of cracking reactions toward high values products. It was firstly introduced by Eugene Houdry in 1924, and it rapidly REPLACED THERMAL CRACKING. He begun working with SILICA-ALUMINA and changed his feedstock FROM LIGNITE TO HEAVY LIQUID TAR. By 1930, he had produced small samples of gasoline with promising features as a motor fuel. The catalytic cracking is structured as follows: 1) CRACKING 2) RECOVERY and SEPARATION of products through DEHYDROGENATION/ISOMERIZATION 3) CATALYST REGENERATION RAW MATERIALS employed in catalytic cracking come from DISTILLATION UNDER VACUUM or from BITUMEN RESIDUES (deprived of asphaltenes). Products are: CnC10 : LIGHT DIESEL with LOW AMOUNT OF AROMATICS employed as fuel and HEAVY DIESEL with HIGH AMOUNT OF AROMATICS used as fuel in refineries. After each cracking cycle, CATALYSTS may be POISONED by TAR and CARBON RESIDUES. In order to REMOVE a significant part of the POISONING TAR, a VAPOR STRIPPING is performed, followed by a HIGH TEMPERATURE AIR COMBUSTION (carbon burning). 50% of US produced gasolines resorts to this process employing AMORPHOUS SILICA-ALUMINA (70% YIELD) and CRYSTALLINE ZEOLITE (85% YIELD) catalysts. CARBOCATIONIC MECHANISM Due to the use of an acidic catalyst, the reaction has a CARBOCATIONIC MECHANISM which starts with: UNSATURATED HYDROCARBONS: the MARKOVNIKOV ADDITION of a catalyst deriving H+ to a DOUBLE BOND; PARAFFINS: Bronsted acid EXTRACTS THE HYDRIDE ION from the paraffin molecule in order to FORM THE CONJUGATED BASE and HYDROGEN; 𝑅3 𝐶𝐻 + 𝐻𝐴 → 𝑅3 𝐶 + 𝐴− + 𝐻2 The propagation step entails the TRANSFER OF THE POSITIVE CHARGE from a primary to a more stable tertiary position. This can lead to the elimination of another proton and the generation of BRANCHED ALKANES, BRANCHED ALKENES, CYCLOALKENES AND AROMATIC molecules with high value. SYNTHETIC AMORFOUS ALUMINIUM-SILICATES CATALYSTS These catalysts were the FIRST used for cracking processes because of their GOOD REACTIVITY, HIGH CONVERSION, GASOLINE SELECTIVITY (45% of the INITIAL FEED, with high amount of olefins and aromatics) AND EASE OF PREPARATION. The PRODUCTION PROCESS consists in 3 main steps: Preparation of Addition of Dehydration at porous silica gel aluminum salt T > 400 °C The preparation of porous silica gel leads to the formation of spherical particles of SILICA HYDROGEL with SILANOL GROUPS AT THE SURFACE. During the second phase, those groups react with ALUMINUM SALTS Al(OH)3 X 3H2O, incorporating them on SILICA SURFACE and generating a NEGATIVE CHARGE ON Al3+, which is compensated by various HYDRATED CATION absorbed on the surface. In fact, silanol as it is not acidic, but it must be functionalized. At high temperature, DEHYDRATION occurs and the BRONSTED acidic sites become LEWIS acidic sites, used in the catalytic activity. This type of catalysts showed LOW THERMAL STABILITY, especially in the presence of steam which occurs during the STRIPPING AND REGENERATION STEP, causing SINTERING, ZEOLITE CATALYSTS Zeolites are NATURAL CRYSTALLINE CATALYSTS with a POROUS 3D STRUCTURE composed by SODIUM ALUMINO-SILICATES. Zeolites are synthesized by MIXING Na2SiO3 and NaAlO2 with a proper ratio and in a specific pH range so that the gel can form and then precipitate. By heating up the precipitate gel, it is possible to PROMOTE the CRYSTALLIZATION PROCESS so that SOME COMPOUNDS (QUATERNARY AMMONIUM SALTS usually) CAN STICK WITHIN THE ZEOLITE CAVITIES. This determines STRUCTURE and SIZE of the zeolite itself. By VARYING THE SIZE of the R GROUP of the ammonium salt, it is possible to affect the DIMENSION OF THE ZEOLITE CAVITIES. Zeolites catalysts are characterized by: HIGHER SELECTIVITY towards C5-C10 gasolines. HIGHER H TRANSFER ACTIVITY rather than hydrocarbon chain breakage activity. The hydrocarbon chain fracture (cleavage) STOPS AT HIGHER MW VALUES after the transfer of hydride ions to carbocations and after the olefins hydrogenation. HIGH REACTION RATE due to the HIGH CONCENTRATION OF REACTANTS within pores. A LOWER AMOUNT of OLEFINS is formed. The major part of the generated olefins are HYDROGENATED BEFORE BEING DESORBED FROM THE CATALYST. COKE and COKE PRECURSORS are the HYDROGEN SOURCE. NAPHTHENES DEHYDROGENATION produces AROMATIC COMPOUNDS. COMPARISON BETWEEN ZEOLITES AND AMORPHOUS ALUMINIUM-SILICATES ALUMINIUM-SILICATES ZEOLITES Gasoline YIELD: 30-35% with octane Gasoline YIELD: 50% with octane number 92-94 number 90-92 Feed REQUIRED to be asphaltenes and Possibility to use CHEAPER FEED as metal free vacuum or thermal cracking distillation Lower activity and selectivity, so residues or materials subjected to the RECYCLE of some gasoil is hydrogen treatments in order to required to increase gasoline yield decrease their amount of metals, and decrease fuel oil asphaltenes and other coke precursors High formation of OLEFINS and Recycle is NOT necessary due to the NAPHTHENES high activity caused by the HIGH Huge formation of COKE CONCENTRATION of reactants INSIDE PORES High formation of PARAFFINS and AROMATICS because of high hydrogen pressure inside pores PLANT FOR CATALYTIC CRACKING 3 major characteristics/ Solid particles fall inside the regenerator. In parameter to addition to the catalyst particles, also coke check: fills the catalyst pores. So, in order to Efficiency regenerate the catalyst, we need to reach a Pressure drop so high T able to burn it Erosion No need of a furnace The preheated FEED, usually composed by ATMOSPHERIC DISTILLATION GASOIL, is sent to a to the RISER (200 t per hour), a LONG TUBE (Ø: 1-2 m; l: 60 m) in which the catalytic cracking OCCURS IN 2-4 SEC. The TEMPERATURE required for the reaction (T > 500 °C) is provided by the heating of the coke and the CONTACT WITH HOT CATALYTIC PARTICLES, causing the feed to vaporize and so the propelling of catalyst and hydrocarbons mix up to the riser. The catalyst particles come from the REGENERATOR, where the coke present on the catalytic surface is burnt at temperatures around 680°C - 720°C. These temperature are obtained through the combustion of air. At the top of the riser there is the CATALYST STRIPPER, which, using STEAM to improve the removal of products from pores, SEPARATES PRODUCTS from the CATALYST PARTICLES. This separation is helped by the presence of CYCLONES. The products are sent to the FRACTIONATOR, a simple distillation column, necessary to separate the LIGHTER FRACTIONS (C1-C2, < gasoline, propane, and butane) from LIGHT AND HEAVY GAS OIL AND SLURRY. Depending on TEMPERATURE and RESIDENCE TIME, some NON- SELECTIVE THERMAL CRACKING reactions can occur, so, even if this type of plants has been extensively used, but, in time, INNOVATIONS to improve it have been found: MILLISECOND CATALYTIC CRACKING (MSCC) REACTOR This type of reactor MINIMIZE THE NON-SELECTIVE POST RISER CRACKING, causing a high INCREASING in GASOLINE SELECTIVITY (+2,3%). The FEED is injected PERPENDICULARLY to the catalyst flow and the PRODUCTS are separated from the catalyst in 2 steps: inside a PRIMARY SEPARATOR and, then, EXTERNALLY to the reactor, in CYCLONES. Thermal processes are minimized due to the ABSENCE OF THE RISER, which reduces the reaction zone, and due to the FAST catalyst-products SEPARATION. The CATALYST STRIPPING is more efficient (we have an increase in liquid yeald), due to the HIGHER TEMPERATURE present, and to the REDUCED HYDROGEN content of the coke. TWO RISERS CRACKING WITH INTERMEDIATE CATALYST COOLER In this reactor there are 2 RISERS working at DIFFERENT CONDITIONS OF TEMPERATURE and an intermediate CATALYST COOLER. This type of structure allows to INCREASE Lower T SELECTIVITY AND YIELDS FOR LIGHT OILS, and to reduce coke formation, thanks to the DIFFERENT CONDITIONS at which the two risers are working. They work also with different feed. FRESH FEEDSTOCK, which is EASY to CRACK but DIFFICULT TO VAPORIZE, DIFFUSE, AND ABSORB Higher T on the active centers of the catalyst, is injected in the FIRST STAGE RISER. Instead, the HEAVY CYCLE OIL (HCO), which has the OPPOSITE ADSORPTION AND REACTION PROPERTIES, is injected in the SECOND STAGE RISER. As regard to the exhausted catalyst, a part is EXCLUDED FROM THE REGENERATION STEP and is then MIXED with the regenerated one in order to DECREASE THE TEMPERATURE. The presence of GASES is due to an unselective HYDROCRACKING. RxCat FCC TECHNOLOGY This technology consists in directing a portion of PRE-STRIPPED CATALYST (not treated with steam) to the MIXING CHAMBER, and not to the regenerator. By combining it with the hot regenerated catalyst, the resulting stream is COOLER, but with a HIGHER CATALYST/OIL RATIO, that ensures HIGHER CONVERSION AND SELECTIVITY AT LOWER TERMPERATURE, avoiding the thermal cracking. Therefore, it allows to INCREASE GASOLINE AND PROPYLENE YIELD, DECREASING GAS YIELD. By manipulating the operational conditions, it is possible to improve the olefins production and so to reach the market objective. HIGH SEVERITY DOWN FLOW FCC Solution to the BACK- MIXING PROBLEM (particles could go back and reduce selectivity) that leads to a NOT EFFICIENT CONTACT with catalyst, and so to lower conversion. Here a DOWN FLOW is exploited, which makes the reactor similar to a PFR. It requires HIGHER TEMPERATURES (650 °C), CATALYST/OIL RATIOS, CATALYST CYRCULATION and RAPID SEPARATION, but it can lead to SHORTER CONTACT TIME, HIGHER CONVERSION, OLEFINS SELECTIVITY AND REDUCED SECONDARY REACTIONS. CONCERNS EXCESSIVE CATALYST LOADING Cyclone erosion and fines generation HIGH CATALYST CIRCULATION Insufficient regenerator and stripper residence time Hydraulic instability EXCESSIVE CATALYST FINES Increased particulate matter emissions HYDROCRACKING This process consists in a CATALYTIC cracking in the presence of HYDROGEN (which happens anyway), which is introduced in order to USE LESS VALUABLE FEEDS, as crude oils and oils derived from simple carbon distillation. The addition of hydrogen leads to a MORE FLEXIBLE PROCESS but, the need of a hydrogen source brings HIGHER OPERATIVE AND INVESTMENT COSTS. However, if a UNIT OF REFORMING, able to generate hydrogen, is already present in the plant, it’s possible to choose this process because of the ADVANTAGES that it implies: Cracking under MILDER CONDITIONS WITHOUT FORMATION OF COKE OLEFINS SATURATION DESULFURIZATION, DEAZOTATION AND DEOXYGENATION Catalysts are Ni or Pt BASED with W, Co AND Mo OXIDES on SiO2 OR Al2O3 SUPPORT. This process can be carried out according to 2 different processes: SINGLE STAGE The first process of this type, that was developed as a SINGLE STAGE, led to LOW CONVERSION (< 60%), with reduced decreasing of MW. The CATALYSTS used contained Co, Mo, Ni SULFIDES, as classical catalysts to promote hydrogenation, supported on ACIDIC ALUMINA, necessary for the cracking. These catalysts were particularly SENSITIVE TO NITROGEN compounds and NH3 formed, which led to the inhibition of the acidic sites. TWO STAGES So, because of these drawbacks, a TWO STAGES process was developed, which consisted in: FIRST STEP: At first there are the desulfurization and deazotation steps which avoid later the deactivation of the catalyst. Catalyst is divided in multiple fixed beds and, being the reaction exothermic, the temperature is adjusted through gas recycling between catalyst beds. SECOND STEP: acidic catalyst with low hydrogenation activity. Unreacted feed is recycled. Use of an aqueous solution containing an amine with a good solubility in water (like ethanolamine) Used in order to keep the T at the right value This gas doesn’t need a scrubber because we already eliminate H2S in first stage Amines which absorbed the acidic gases (H2S) → they must be regenerated The process starts with a first reactor filled with HYDROGENATION PROMOTING CATALYST divided in multiple fixed bed. Inside this reactor DESULFURIZATION AND DEAZOTATION REACTIONS occur at mild conditions and taking advantage of the presence of hydrogen. Then, there is a HIGH-PRESSURE SEPARATOR aimed to separate the products of this first stage from the hydrogen, which can be RECYCLED after another DESULFURIZATION TREATMENT in the AMINE SCRUBBER. Products are sent to a LOW-PRESSURE SEPARATOR in which the GASES generated exit as OFF-GAS, whereas the LIQUID part is heated and sent to the FRACTIONATOR. There high valuable products are separated, with particular interest in the production of DIESEL. The BOTTOM of this distillation column is heated again and sent to a SECOND REACTOR, similar to the first one, with CRACKING PROMOTING ACIDIC CATALYST as Pt-ZEOLITES. After this step, the stream undergoes the same previous passages (High/Low pressure separators and fractionator). ZEOLITES are particularly useful catalysts both for CATALYTIC CRACKING AND HYDROCRACKING, due to their unique properties: Strongly ACIDIC catalytic sites UNIFORM PORE STRUCTURE High STABILITY AND DURATION High SELECTIVITY FOR REAGENTS (*), PRODUCTS(**) OR TRANSITION STATES(***) (shape selectivity) Higher GASOLINE YIELDS (*) REAGENT SELECTIVITY: only molecules smaller than a critical value can enter the pores and get into the active sites (linear hydrocarbon)→ The structure of zeolites is ideal to ensure some selective conversion (**) PRODUCT SELECTIVITY: only certain products can exit active sites and move through channels (p-xylene). Important for PET: its production through a cracking process is smaller than for other isomers. (***) TRANSITION STATE SELECTIVITY: some reactions do not occur since the transition state requires more space than available one in the cavity. HYDROCRACKING OF BIOMASS-DERIVED MATERIALS Recent studies have demonstrated the possibility to use alternative starting material, instead of hydrocarbons, in example, CELLULOSE obtained from RESIDUES of agricultural products: in this case, before HYDROCRACKING, cellulose needs a PRETREATMENT IN ALCHOL to LOWER the MWs, reaching in this way C2-C9 YIELDS around 89%. FEEDSTOCK PROPERTIES It’s fundamental to consider the COMPOSITION OF THE FEEDSTOCK, which is going to be treated. In fact, the presence of particular species can lead to lower yields and catalyst poisoning, so loss of money. Olefines and asphalthenes (presence of specific treat. with solv) Promote the coke formation and the reversible inhibition of the catalyst (Hydrogenation to saturated compounds) Sulfur Its presence increases the SO2 emissions during catalyst regeneration (Removal with amine washing or Claus process) Nitrogen, Oxygen and Arsenic species Nitrogen can generate ammonia, which neutralize the acidic catalyst site, instead oxygen and arsenic can poison Pt catalyst Organometallic compounds They are transformed into heavy metals (Fe, Ni, V) that, depositing over the catalyst, can reduce its activity, furthermore V causes sintering Na and K permanently neutralize zeolites acidic site The removal of all this dangerous species can be performed through a HIGH PRESSURE (5-20 atm) HYDROTREATING, with a catalyst based on Co-Mo, Ni-Mo or Ni-W, on feedstocks before the cracking. HYDROTREATING Hydrotreating can be used for: the REMOVAL of SULFUR COMPOUNDS by catalytic process. The H2S thus formed can be removed by AMINE WASHING and it can be furtherly transformed into Sulfur through the CLAUS PROCESS. the removal of O, N and As compounds FROM THE REFORMING FEED in order to PREVENT PT CATALYST POISONING. Moreover, OLEFINS HYDROGENATION REDUCES the COKE FORMATION over the catalyst. the DESULFURIZATION of GASOIL for DIESEL FUELS and FUEL OIL; S, N and METAL REMOVAL from CATALYTIC CRACKING FEED in order to control the CATALYST DEACTIVATION. Hydrotreating can be: MILD → UPGRADING LUBE OILS properties such as scent, color and stability. HIGH P → REMOVAL of S, O, N compounds and REAROMATIZATION. The catalyst employed are Co-Mo, Ni-Mo or Ni-W. P: 5-20 atm. HYDROTREATING HYDROCRACKING Remove heteroatoms and saturate Severe form of hydroprocessing: C-C bonds + sulfur, nitrogen, oxygen break C-C bonds + drastic reduction and metals removed + olefinic and of MW aromatic bonds saturated Minimal cracking Higher yield of fuel products Minimal conversion: 10-20% 50%+ conversion Products suitable for further Product more appropriate for diesel processing or final blending: than gasoline reforming, catalytic cracking, hydrocracking Inside this gas line there are both new hydrogen and recycled one C6 rich fraction isomerization (Benzene formation) Pt catalyst→ sensible to sulfur due to deactivation Used to remove H2S Instead of having a stabilizer, here we have the addition steam in order to remove of H2S from hydrocarbons GENERAL FEATURES ON PETROLEUM PRODUCTS The PETROCHEMICAL INDUSTRY is based on transforming LOW valuable raw materials into HIGH VALUABLE PRODUCTS, which usually contain a HIGHER H/C RATIO, with 2 possible processes. RESIDUE CONVERSION Conversion processes related to the TREATMENT OF THE RESIDUE are acquiring a noteworthy importance year by year. This is mainly due to economic reasons since HEAVY CRUDE OILS require ADDITIONAL TREATMENTS. By performing a conversion, it is possible to RECOVER A LARGE AMOUNT OF THE RESIDUE to save as much as possible and to reduce wastes. Residue features: HEAVY, DIRTY, LOW VALUE, LIMITED USES. HIGH MW, STABLE AND CARBON RICH, HYDROGEN DEFICIENT. Contains SATURATES, AROMATICS, RESINS AND ASPHALTENES. Impurities are S, N AND HEAVY METALS. Residue is a GENERAL TERM that collects DISTILLATION RESIDUES, HEAVY OILS, BITUMEN AND TAR SAND. HYDROCRACKING Hydrogenation Processes HYDROTREATING VISBREACKING (T) Carbon Removal Processes COKING (T) Thermal (T) or catalytic (C) ASPHALTENES EXTRACTION (T) CATALYTIC CRACKING (C) RESIDUE CATALYTIC CRACKING As far as catalytic cracking for residue conversion is concerned, GASOIL FLUID CATALYTIC CRACKING (FCC) has faced SEVERAL IMPROVEMENTS AND DEVELOPMENTs so that a HIGHER AMOUNT OF GASOLINE COULD BE GENERATED. In order to be able to perform this operation, some SPECIAL CATALYSTS ARE REQUIRED due to the HIGH AMOUNT OF METALS INSIDE THE FEED. Indeed, the content of asphaltenes and catalysts metal poisoners should always be monitored. In particular, the metal content of Ni and V shall never exceed 10-30 ppm otherwise a hydrogen treatment would be required. Ni LOWERS LIQUIDS YIELD and INCREASE COKE FORMATION; V REDUCES both the CONVERSION AND YIELD and downgrade the catalyst. COKE DEPOSITION ON CATALYST is more recurring than in normal gasoil catalytic cracking and novel research focus on the development of new catalysts. From the process point of view, this leads to a reduced residence time and some regeneration step. Modern reactors are equipped with CATALYST COOLING SYSTEMS WITHIN THE REGENERATOR UNIT or with a SECOND REGENERATION UNIT in order to remove the EXCESS HEAT generated during coke combustion. CATALYTIC CRACKING is suitable for residues with A CONRADSON CARBON INDEX (weight of the obtained residue after having heated up until complete vaporization and vapor combustion) LOWER THAN 15%, which is more or less the 40-50% OF THE AVAILABLE RESIDUES. VISBREAKING It’s a plant for thermal cracking able to convert DISTILLATION RESIDUES into gas, naphtha, distillates, and residues with LOWER VISCOSITY than the feed, namely, to reduce the amount of residue in favor of MIDDLE DISTILLATES. The main aim is to REDUCE THE VISCOSITY from 1500 cSt to 600 cSt. The condition of TEMPERATURE AND PRESSURE are set specifically for the type of residues treated and, usually, are around 450-480 °C and 4-5 atm. The plant can lead to a CONVERSION in gas and distillates is about 10-50%, depending on the conditions of the feed. The cracking reactions occur inside the FURNACE AND CONTINUES IN THE SOAKER, which is a sort of ABSORBER EQUIPPED WITH TRAYS in order to INCREASE CONTACT TIMES and to avoid UNDESIRED PRODUCT TO REVERSE BACK in the furnace. By increasing the residence time of the feed, the operating T can be decreased which means an energy saving. At the top of the soaker, there is the QUENCH ZONE where the catalytic reactions are stopped. The FRACTIONATING TOWER enables to separate products. Reactions are ENDOTHERMIC (the outlet product exits at A LOWER TEMPERATURE than the inlet feed). The temperature in further decreased to stop cracking. The major drawback is that PERIODIC CLEANING WITH PLANT STOP is necessary. To overcome this problem, a NEW DESIGN was developed to CUT OFF SECTIONS DURING CLEANING, without stopping the plant. TWO STAGE THERMAL CRACKING It is a more SOPHISTICATED thermal process focusing to the MAXIMIZATION OF GASOIL. A portion of DISTILLATE is RECOVERED from the fractionating column and the vacuum column is fed to a SECOND FURNACE and subjected to a SECOND STAGE CRACKING in order to get a higher amount of gasoil. The main part of gasoil can be used to UPGRADE RESIDUE PROPERTIES by dilution, and satisfy fuel oil specifications. COKING Coking is a refinery unit operation that UPGRADES HEAVY COMPOUNDS (bottom products from distillation), turning them into HIGHER-VALUE PRODUCTS. Coke isn’t always a by-product, in fact, it can be used as a FUEL, for anode manufacturing in aluminum industry, for graphite electrodes manufacturing in steel industry, depending on its FEATURE, in particular, its THERMAL ESPANSION COEFFICIENT (CTE) by heating. The coke formation in enabled by a THERMAL CRACKING characterized by A LONG-LASTING RESIDENCE TIME. Working AT HIGH TEMPERATURE AND LONG CONTACT TIMES allows the DISSOCIATION in SIMPLE ELEMENTS, as reported on Francis Diagram. Altogether with black carbon coke, this process produces HIGH OCTANE NUMBER GASOLINES but with a HIGH AMOUNT OF OLEFINS. This means that the product is RATHER UNSTABLE. However, it can be STABILIZED THROUGH HYDROGENATION. Cocking consists of THREE PHASES: 1. PARTIAL EVAPORATION and CRACKING in the FURNACE. 2. VAPORS CRACKING inside COKE DRUMS. 3. CRACKING and POLYMERIZATION OF LIQUIDS inside coke drums until they are CONVERTED INTO VAPORS AND COKE (18-24 h). EFFECT OF THE TEMPERATURE: as the T INCREASES, the COKE YIELD DECREASES but its HARDNESS INCREASES (its removal from coke drums is difficult). EFFECT OF THE PRESSURE: as the P INCREASES, GASES AND COKE YIELDS INCREASE whereas LIQUID (C>5) YIELD DECREASES and the GASOLINE ENDPOINT IS LOWERED. Higher pressure improves the coke quality by lowering the Coefficient of Thermal Expansion (CTE) and electrical resistivity. The PRESENCE OF A RECYCLE SYSTEM has the SAME EFFECT AS THE PRESSURE. It is essential to optimize the gasoline endpoint. So, there are SEVERAL TECHNOLOGIES which are able to produce coke: DELAYED COKING It represents one of the MOST EMPLOYED TECHNIQUES for coking processes. Feedstock: material coming from THE VACUUM UNIT (otherwise used as road asphalt). Purpose: transform asphalt-like material to produce HIGHER VALUE PRODUCTS (gasoline, diesel fuel, LPG and petroleum coke). The FRESH FEED enters the bottom of the FRACTIONATING COLUMN where it is mixed with RECYCLED MATERIALS coming from the washing section. This mixture exiting from the BOTTOM OF THE COLUMN is then sent to a FURNACE where it is heated at the COKING T (485-505 °C). The heating causes the HEAVY LIQUID MATERIAL TO CRACK or change into a COMBINATION OF SMALLER MOLECULE GAS and liquid products. CONDENSED VAPOUR is added from the furnace pipes in order to keep a minimum FLOW RATE CONSTANT. The hot compounds then enter the COKER UNIT. This unit has two coke drums that are placed in parallel so that they can OPERATE IN PAIRS IN A SEMI-BATCH MODE. The material at HIGH T AND LOW P coming from the furnace unit is INJECTED INTO THE BOTTOM OF THE DRUM where it is further cracked into gaseous products. COKE PILES UP WITHIN THE DRUM whereas the HC vapours exit the column from the top and RETURN TO THE FRACTIONATOR FOR PRODUCT RECOVERY. Fuel gas and LPG are recovered from fractionator as fuel application or other purposes. Naphtha is recovered and sent to other refinery units for gasoline production. Light Coker Gas Oil (LCGO) and Heavy Coker Gas Oil (HCGO) are sent to Hydrotreating for processing into diesel and other products. The OFFLINE DRUM is STEAMED, VENTED AND COOLED before the drum is opened to atmosphere. After the drum is opened, the PETROLEUM COKE IS CUT FROM THE DRUM USING HIGH PRESSURE WATER. This petroleum coke is similar to coal, and it is typically used as fuel in power plants. As the coke is cut by high pressure water, COKE AND WATER FLOW INTO THE COKE PIT, where the WATER IS SEPARATED and RECYCLED BACK TO THE CUTTING WATER SYSTEM. Conversion 80% FLUIDISED BED COCKING The plant consists in A COKE FLUIDIZED BED REACTOR COUPLED WITH A FURNACE-BURNER UNIT. Coke is kept IN MOTION BY A STEAM FLOW entering from the bottom of the reactor and by the forming vapour during its gradual rise towards the top of the reactor. The FEED IS DIRECTLY SENT TO REACTOR R where it meets the SCORCHING COKE (500-550 °C). By subtracting that heat from the coke, the feed undergoes CRACKING, AND IT TURNS INTO VAPOUR. This vapour goes up across the RECTIFYING COLUMN and it is split into DIFFERENT FRACTION CUTS. The amount of vapour that did not undergo cracking or has a high boiling T return into the 500-660 °C 3-4 atm cracking unit. NOVEL COKE is produced by CRACKING INSIDE the REACTOR, and it DEPOSITS ON THE EXISTING COKE. The COLD COKE laying on the bottom of R is sent to THE BURNER B. In the burner, coke undergoes a PARTIAL COMBUSTION with AIR so that its temperature is raised before being sent back to R. A fixed amount of the produced coke is DIRECTLY COLLECTED FROM THE BOTTOM of the burner whereas COMBUSTION GASES ARE COLLECTED BY A CYCLONE (C) on top of B so that carbonaceous particles can be removed. Fluidised bed coking generates more gasoil but less gasoline than delayed coking. Heavier fractions can be employed as feedstock, but this requires higher installation costs. Delayed Products Fluid Coking* Coking* Gas ≤ C4 7,5 6,5 Gasoline 17 10 Gasoil 53,5 72 Coke 22 11,5 FLEXICOKING The main difference in respect to other plants is the ADDICTION OF A GASIFIER to the fluid coking near the burner. In this way the process is more flexible because around 90% of the feed is converted into LIQUIDS AND GAS and the EXCESS OF COKE can be further converted into gas useful as energy source or raw material for chemical products. ASPHALTENES EXTRACTION Asphaltenes are the HEAVIEST PETROLEUM components, mostly polycyclic molecules, containing a lot of impurities, SOLID AT ROOM TEMPERATURE. Their removal from the feed INCREASES GASOIL YIELD during the cracking or hydrocracking and, furthermore, they can be used as fuels in the cement industry and in ASPHALT MANUFACTURE. The DEASPHALTING PROCESS by solvents allows the recovery of non- asphaltene materials both from the atmospheric residue and from the vacuum residue. The targets of this process are: ASPHALTENES REMOVAL from feed to LUBE OIL MANUFACTURE. INCREASE OF THE GASOIL YIELD from oils to be FED TO CRACKING OR HYDROCRACKING PLANTS. TAR PRODUCTION. MULTISTAGE DEASPHALTING UNIT Need to reduce the pressure in order to have again a liquid propane LIGHT PARAFFINS, from propane to pentane, are employed as SOLVENT. This process resorts to the DIFFERENT SOLUBILITY IN SOLVENTS for the separation of hydrocarbons. The EXTRACTED MATERIAL is called DEASPHALTED OIL (DAO) and it is mainly composed of LOW MW PARAFFINS. AROMATIC AND HEAVIER FRACTIONS remain in the ASPHALTENIC REFINED PRODUCT. Heat added to top EXTRACTION STAGE TO DECREASE SOLUBILITY and INCREASE PRECIPITATION of asphaltenes Original deasphalting process operated at 400 psig and solvent was recovered both FROM PRODUCT OIL and FROM ASPHALT RESIDUE by flashing to 1 atm. Usually, YIELD OF DEASPHALTING PROCESSES ranges from 75% to 80%. However, the METAL CONTENT INCREASE WITH THE YIELD. It is to figure out the best compromise between DAO yield and metal content (and its removal). RESIDUUM OIL SUPERCRITICAL EXTRACTION (ROSE) PROCESS Modern processes, as ROSE PROCESS (Residuum Oil Supercritical Extraction), take advantage of the use of SUPERCRITICAL SOLVENTS to extract high yields of asphaltenes, with LOW INVESTMENT COSTS and ENERGY SAVINGS. Indeed, due their characteristics the EXTRACTION AND PHASE SEPARATION RATES of a supercritical fluid are SIGNIFICANTLY HIGHER compared to those observed in typical extraction processes. In this new technology, HYDROCARBON SUPERCRITICAL SOLVENTS, as CO2, are used to extract products without asphaltenes. The solvent CHOICE depends on the desired DEASPHALTED OIL (DAO) purity and yield, which increase the simplicity in further manufacturing. DAO STRIPPER and asphaltenes stripper allow an efficient SOLVENT RECOVERY, which can be an ECONOMICAL ADVANGE both because it’s recovered as SUPERCRITICAL FLUID, therefore cost of recompression are cut, and the UNITS are SMALLER, with consequent lower investment costs and energy consumption. STEAM CRACKING It’s the most important industrial process because it produces many starting materials for petrochemical industry, such as: o ETHYLENE (140 x 106 t/a)→ nowadays steam cracking is the most used method but there also others: ▪ Partial C2H2 hydrogenation ▪ EtOH dehydration ▪ Coke combustion gases o PROPYLENE (70 x 106 t/a), o C4 OLEFINS o and HEAVIER UNSATURATED COMPOUNDS. The CHOICE OF THE FEEDSTOCK for this process depend upon the market for the most obtained products. Historically, NAPHTHA, NATURAL GAS AND ETHANE have been the most used. STEAM CRACKING RADICAL MECHANISM Steam cracking is a THERMAL CRACKING process and takes advantage of the HOMOLYTIC BREAKING OF BONDS, caused by the HIGH TEMPERATURES (900 °C), of high MW molecules. In particular, molecules undergo β-CLEAVAGE, causing the generation of ETHYLENE AND PROPYLENE. In the β-CLEAVAGE, a HIGHER ENERGETIC PRIMARY RADICAL, which is easily formed because of the temperature, tends to generate a SECONDARY RADICAL, that is transformed into a DOUBLE BOND. This path is possible because the ENERGY DIFFERENCE between primary and secondary radicals is not very large. The overall ENTHALPIC INCREASE is compensated for by the INCREASE IN ENTROPY that results from breaking of LARGER MOLECULES INTO SMALLER ONES. Because of this increasing of number of moles, the reaction is also LOW PRESSURE DRIVEN: the addiction of STEAM is necessary to decrease the partial pressure of hydrocarbons. from ETHANE From PROPANE As regard to the steam cracking, not only chain shortening reactions are favored, but also ISOMERIZATION AND CYCLIZATION. As secondary reactions there are olefin polymerization, alkylation, and condensation of aromatic molecules. These reactions lead to a mixture of PRODUCTS AT VARIABLE COMPOSITION AND ECONOMICAL VALUE, which depend on the DIFFERENT FEEDSTOCKS: Pyrolisis KINETIC AND THERMODYNAMIC OF THE PROCESS All olefins are THERMODYNAMICALLY UNSTABLE with respect to H2 and COKE, whose formation is unavoidable, so, the PRODUCT DISTRIBUTION is mainly regulated by KINETIC PARAMETERS: TEMPERATURE At 400 °C hydrocarbon chains are BROKEN in the MID of the molecules By RAISING T the chain breaking is shifted towards the TERMINAL part of the molecule and so producing a higher amount of lower olefins REACTION VELOCITY increases with T RESIDENCE TIME SHORT tr provides HIGHER AMOUNT OF OLEFINS and less formation of by- products as coke and oligomers HYDROCARBON PARTIAL PRESSURE The formation of lower olefins leads to a pressure increase but the reaction is favored at LOWER PRESSURE STEAM is added to DECREASE HYDROCARBON PARTIAL PRESSURE AND MINIMIZE COKE FORMATION through the water-gas shift reaction (C + H2O → CO + H2), which enables to extend the duration of the continuous process. It also reduces OLIGOMERIZATION by favoring the fragmentation Steam furnishes some HEAT needed for the endothermic reaction The PROCESS CONDITIONS to obtain a higher amount of interested products should be evaluated by OPTIMIZING the previous KINETIC PARAMETERS and by FINDING SOLUTION TO THE PROCESS DEMANDS: Very HIGH TEMPERATURE (900 °C) for very SHORT TIME ( 90%) can be obtained in the PRESENCE of t-BuOH, which is formed in situ by co-feeding small amounts of WATER to the same reactor. In the PURIFICATION step, ISOOCTENE is SEPARATED from the UNREACTED FEED (raffinate C4). Moreover, a t-BuOH enriched stream can also be recovered and RECYCLED to the reactor. Isooctene can be HYDROGENATED to isooctane for markets with strict olefin limitations. GASOLINE BLENDING PRODUCT BLENDING is the operation that, at the end of every refinery, blends STREAMS in various proportions to produce FINISHED REFINED PRODUCTS, whose properties meet all applicable industry and government standards, at MINIMUM COSTS. The various STANDARDS requires concern about PHYSICAL PROPERTIES (density, volatility and boiling range), CHEMICAL PROPERTIES (sulfur content, aromatics content), and PERFORMANCE CHARACTERISTICS (octane number and smoke point) and they are constantly ANALIZED AND MONITORED to meet all the blend specifications for the required product volume. Production of each finished product requires MULTI-COMPONENT BLENDING (Typically, gasoline is a mixture of 6–10 blendstocks and diesel fuel is a mixture of 4–6 blendstocks) because: Refineries produce no single blend component in SUFFICIENT VOLUME to meet demand for any of the primary blended products such as gasoline, jet fuel, and diesel fuel; Many blend components have properties that satisfy some, but not all, of the relevant STANDARDS for the refined product; COST MINIMIZATION dictates that refined products be blended to meet specifications. The gasoline blend is more or less influenced, in terms of QUANTITY, by the most important PROCESSES in a refinery: CATALYTIC CRACKING 34 % REFORMING 28 % ALKYLATION 19 % ISOMERIZATION 15 % And the QUALITY OF COMPOMENTS also depends on this: Nowadays, the usual practice is to blend all of these streams and then desulfurize them in a single distillate hydrotreater Where RVP is the REID VAPOUR PRESSURE, namely a measure of the volatility of the mixture. DESULFURIZATION The desulfurization is one of the most widespread catalytic processes due to the DECREASING CRUDE OIL QUALITY and the LIMITATIONS on sulfur allowed in fuels, which caused the necessity of HUGE INVESTMENTS ON SULPHUR CONTROL unit in many refining processes. Furthermore, it is used to PRESERVE CATALYSTS FROM POISONING in many fundamental processes that uses NOBLE METAL-BASED catalysts, such as reforming, catalytic cracking and hydrocracking. It is also used to REDUCE the CORROSION during refining processes or to REDUCE HARMFUL EMISSIONS. Sulfur atoms of various SULFURETED COMPOUNDS react with H2, which is generated through REFORMING unit, and are transformed into HYDROGEN SULPHIDE H2S, a toxic compound which can be converted to innocuous ELEMENTAL SULPHUR through the CLAUS PROCESS. The HYDRODESULFURIZATION reactions are EXOTHERMIC, so, to favor their thermodynamic, they are conducted at LOW AND CONTROLLED TEMPERATURE (300-400 °C) and LOW PRESSURE (8-40 atm), to avoid coke formation and hydrocracking. During these treatments, other HETEROATOMS, such as oxygen and nitrogen, are converted into WATER or AMMONIA, and OLEFINS are HYDROGENATED to paraffins. These side reactions represent an unwanted HYDROGEN CONSUMPTION, so, it’s fundamental to evaluate the H2 amount needed, according to the FEEDSTOCK treated (heavier feedstock as gas oil required more hydrogen consumption, if compared to gasoline and kerosene). Typical reactions are: HYDRODESULFURIZATION CATALYSTS In the first part of the plant, the feed is properly mixed with H2. The mixture is then brought to the PROPER CONDITIONS before entering the REACTOR where the temperature is controlled through H2 ADDICTION ON Gaseous CATALYTIC BED. stream Usually, the CATALYSTS present are TRANSITION METAL SULPHIDES, charged as metal oxides and converted to the metal sulfides in the reactor. The most used mixture are MOLYBDENUM and COBALT SULFIDES (15-20%) ON ALLUMINA SUPPORT. Co is used to INCREASE ACTIVITY and to LIMIT SINTERIZATION. The catalyst is prepared through impregnation of γ-Al2O3 with aqueous ammonium molybdate and cobalt nitrate and is converted to the OXIDE through dehydration and calcination. Afterwards, it is treated with GASEOUS DILUTED H2S STREAM or MERCAPTANS present in the reactor mixture, causing the SULFURATION that is the cause of the ACTIVITY. The catalyst may be REGENERATED through controlled COMBUSTION at T