Weathering of Rocks: A Comprehensive Study PDF
Document Details
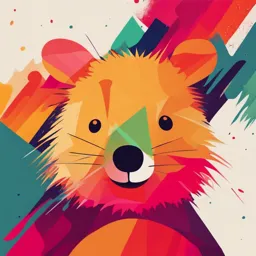
Uploaded by FriendlyTrust
University of KwaZulu-Natal - Westville
Tags
Summary
This document provides a detailed explanation of weathering processes, covering both physical and chemical aspects. It explores various types of mechanical weathering, chemical weathering (including hydrolysis, dissolution, and oxidation), factors affecting weathering rates, and the fate of weathering products. The content includes illustrations, diagrams, and examples to enhance understanding.
Full Transcript
WEATHERING Weathering Weathering = chemical weathering (decomposition) + mechanical weathering (disintegration) of rocks and minerals at the Earth’s surface MECHANICAL (PHYSICAL) WEATHERING Mechanical weathering Physical forces break rock into smaller fragments without ch...
WEATHERING Weathering Weathering = chemical weathering (decomposition) + mechanical weathering (disintegration) of rocks and minerals at the Earth’s surface MECHANICAL (PHYSICAL) WEATHERING Mechanical weathering Physical forces break rock into smaller fragments without changing its mineralogical and chemical composition. Mechanical weathering increases the surface area of the fragments. Volume remains constant at 1 m3 Surface area increases Types of mechanical weathering Ice (frost) wedging Salt crystallization Sheeting and spheroidal weathering Thermal expansion Abrasion Biologically induced mechanical weathering Ice (frost) wedging alternate freezing and thawing of water in fractures and cracks promotes the disintegration of rocks water expands as it freezes (by 9%), exerting a force great enough to break rock effective in cold climates and mountainous regions with frequent freeze/thaw cycles Michael Hambrey Boulder Fractured by Frost Action Salt crystallization water containing dissolved salts (groundwater, sea water) may penetrate into cracks upon evaporation salt crystal growth can produce pressure that results in rock fracturing effective in coastal areas and desert regions where rising groundwater evaporates Honeycomb weathering Sheeting Sheeting: fracturing into slabs (0.1-10 m thick) parallel to the rock surface. Sheeting in granite, Enchanted Rock, Texas Sheeting commonly caused by a decrease in overburden pressure due to erosion (unloading) Spheroidal weathering Spheroidal weathering: the peeling away (exfoliation) of concentric shells of decayed rock. ≈ onion skin weathering caused by water seeping into the fractures of jointed rocks and causing chemical weathering. Thermal expansion During short-term heating of a rock mass, only the margin heats and expands, producing differential stresses that cause the rock exterior to peel off. effective during forest fires, possibly also in areas with large daily temperature variations Biologically induced mechanical weathering The roots of trees can grow into cracks in rock and wedge them open. Abrasion The mechanical wearing of rock surfaces by friction and impact of particles moving in a flow of water, wind or ice. Yardangs CHEMICAL WEATHERING Chemical weathering Chemical weathering is the breakdown of rocks by chemical decay of their minerals. As a result, new minerals are formed that are stable at the earth’s surface. The most important agent involved in chemical weathering is water. The most important processes involved are: hydrolysis, dissolution, oxidation Chemical weathering is generally a combination of several processes acting together. Hydrolysis Decomposition reaction in the presence of water under acidic conditions. – Water absorbs CO2 (from air/ bacterial decay of vegetation) and forms carbonic acid. – Carbonic acid ionizes to form hydrogen and bicarbonate ions. H+ ions enter a mineral’s crystal structure and replace metallic cations, which may be leached away in solution. Hydrolysis A hydrolysis reaction of orthoclase (alkali feldspar), a common mineral found in igneous rock, yields kaolinite, silicic acid, and potassium. 2KAISi3O8 + 2H+ + 9H20 → 2K+ + H4Al2Si2O9 + 4H4SiO4 (orthoclase + hydrogen ion + water → potassium ion +kaolinite + silicic acid ) Hydrolysis Water breaks cation bonds in silicate minerals. Yields… Dissolved cations. Alteration residues. Clay minerals. Iron oxides (rust). Marshak: Fig B8 Dissolution Acidic conditions aid in the dissolution of minerals, particularly carbonates. Water reacts with CO2 to form carbonic acid. H2O + CO2 >>> H2CO3 The acid then decomposes minerals. H2CO3 + CaCO3 >>> Ca+2 + 2HCO3- (carbonic acid + calcite >>> calcium ion + bicarbonate ion) Dissolution Minerals and rock materials are dissolved by a liquid agent (generally water). Cango caves were formed during the last 20 million years by rainwater seeping through fissures in the limestone. Gradually the water dissolved the limestone, forming an extensive network of subterranean caverns and tunnels. When the acidic oxygen in the rainwater comes into contact with the calcium carbonate in the limestone, a crystalline solution is formed, which hardens and eventually accumulates as stalagmites, stalactites and flowstones. Oxidation Oxidation: any chemical reaction in which an atom or molecule looses electrons. Oxygen dissolved in water is the most common oxidising agent. – most elements are oxidized at the Earth’s surface. – but below the groundwater table conditions are generally reducing Oxidation is especially important in the weathering of Fe-rich minerals. The oxidation of the iron in a ferromagnesian silicate starts with the dissolution of the iron. For olivine, the process looks like this where olivine in the presence of carbonic acid is converted to dissolved iron, carbonate, and silicic acid: Fe2SiO4+ 4H2CO3 —> 2Fe2+ + 4HCO3– + H4SiO4 olivine + (carbonic acid) —> dissolved iron + dissolved carbonate + dissolved silicic acid In the presence of oxygen, the dissolved iron is then quickly converted to hematite: 2Fe2+ + 4HCO3– + ½ O2 + 2H2O —->Fe2O3 + 4H2CO3 dissolved iron + bicarbonate + oxygen + water —->hematite + carbonic acid Hydration Absorbtion of water into the crystal structure resulting in the formation of a hydrous mineral. e.g., hydration of anhydrite to gypsum CaSO4 + 2H2O → CaSO4.2H2O Hydration involves a volume increase that may disrupt the structure of the rock. Factors affecting chemical weathering Climate Mineral composition Time Rock structure Presence of soil Slope Climate Moisture and heat promote chemical reactions. Chemical weathering – is intense in humid, tropical environments (lots of water and high T) – is slow in polar regions (low T) and deserts (paucity of water). Warm, humid climates also tend to have more plants. – Plant decomposition results in the production of organic acids that promote chemical weathering. Mineral composition The rate of chemical weathering of rocks is related to the stability of the minerals in the rock. Slate Marble Both from 1780’s Time The longer a rock or mineral is exposed to weathering the more weathering takes place. Rock structure Heavily fractured/ jointed rocks weather faster than massive rocks. Jeff Foott/DRK Presence of soil Weathering and soil formation are positive-feedback processes. – if soil starts to form, rock weathers more rapidly and more soil is formed. – partly because water in soils is more acidic Slope Weathering residues are chemically stable. – > as they build up the rate of chemical weathering decreases – > fresh rock weathers faster than weathered rock On steep slopes the products of weathering are eroded and fresh rock is continually exposed to renewed weathering attack. Slope The slope of the weathering area can also influence the type of sediment produced. For example, rapid mechanical erosion of granitic mountains results in an accumulation of sediment rich in quartz and feldspar. If the same mountains were low hills, chemical weathering will predominate, the feldspars will alter to clay and be removed, and the sediment will become dominated by quartz. Weathering of granite Weathering of granite A granite decomposes by the combined effects of dissolution, hydrolysis, and oxidation. – Feldspar, mica, and ferromagnesian minerals weather to clay minerals and soluble Na+, K+, and Mg2+ ions. – The quartz grains remain essentially unaltered. Weathering of basalt Weathering of basalt Plagioclase feldspar and ferromagnesian minerals form clay minerals and soluble ions (Na+, Ca2+, Mg2+). The iron from ferromagnesian minerals, together with iron from magnetite, forms iron oxides. Weathering of limestone Dissolution and hydrolysis leave behind only the nearly insoluble impurities in limestones (mainly clay and quartz). Weathered Limestone Fate of the weathering products Material in solution (Na, K, Mg, Ca ions) taken up by plants groundwater fluvial runoff (into lake, sea; -> biochemical and chemical sediments) Fate of the weathering products Residual material (clay minerals, Fe-oxides) remains at the site of weathering in the weathering profile becomes eroded and transported by fluvial, aeolian, and other processes (-> siliciclastic sediments) Mechanical vs chemical weathering Both processes are interrelated. Chemical weathering aids fragmentation by opening channels for water to penetrate the rock. Fragmentation speeds chemical decay as the rate of chemical reactions increase with increasing rock surface area. Intensity of physical and chemical weathering in relation to rainfall and temperature Weathering rates f (lithology) – chemical & mineralogical composition f (climate) – temperature, precipitation, vegetation SOILS Terms Bedrock: unaltered, solid rock of any kind. Regolith: unconsolidated, weathered material that rests on and is derived from the weathering of bedrock. (regos = blanket, lithos = stone). Soil: regolith at the Earth surface that contains organic matter and is able to support vegetation. Soils Soils are produced by: The mechanical and chemical weathering of bedrock. The organic matter derived from the decay of dead plants and animals. Soils develop from the surface downwards. The soil surface experiences the greatest weathering. Soil horizons Characteristic layers (soil horizons) develop below the surface Water leaches material from upper layers that is transported downwards Some material is deposited in lower soil layers. Soil profile The vertical succession of soil horizons is called a soil profile. There are numerous types of soils that are characterized by different soil profiles. Soil Taxonomy Hierarchy Kingdom Phylum Class Order Order 12 Family Suborder 63 Genus Species Great group 250 1400 Sub group Family 8000 19,000 Series The O horizon The top layer of a soil consisting of variably decayed organic matter (OM). The A horizon May either underlie an O horizon or lies directly beneath the surface. Dark-coloured because of the presence of humus, but becomes lighter coloured downwards. Humus: decomposed residue of plant and animal matter Cations are leached and clays are removed from the A horizon. Chernozem Ah Bw Bk The E horizon A light - coloured zone below the A horizon with little or no OM. E = eluviation process by which water removes material from soil and transports it downwards E horizon The B horizon Enriched in clays and cations removed from the A (and E) horizon (illuviation). Accumulation of clay may produce a hard, tough soil horizon known as a hardpan. Chernozem Ah Bw Bk The C horizon Deepest horizon of a soil profile. Consists of rock in various stages of weathering. Grades downwards into fresh bedrock. Note: O, A, E, B, C horizons are not all developed in all soil profiles. Factors affecting soil formation Parent material Topography Vegetation Drainage Time Climate Parent Material Bedrock or sediment from which soil forms. Influences type and nutrient richness of a soil (e.g., quartzite vs basalt). Topography Influences the availability of water and the rate of soil accumulation. Soils thin with an increase in slope gradient, as water and weathered material migrate downslope. decrease in soil thickness Topography Refers to the concept of accommodation space Space available for “sediment” to be stored and to accumulate within decrease in soil thickness Vegetation Contributes OM to soil. Produces CO2 and O2 involved in chemical weathering. Decay of plants releases organic acids that enhance chemical weathering. The root system of plants protect soil from erosion. Drainage Type of soil strongly depends on the height of the groundwater table. A high ground water table results in poorly drained soils - bog-type soil saturated with water may have a high content of OM. Poor drainage = water-logged = reducing conditions Time The longer the time the thicker the soil produced. Soils develop over the course of decades to thousands of years, depending on climate. Climate Precipitation and temperature influence soil formation - as it effects the rate of weathering and plant growth. Soil formation is most rapid in warm, moist climates. Climate Tropical and subtropical regions soils are deeply weathered, low OM content Arid regions limited plant growth, low OM content, salt precipitation Humid temperate regions soils are relatively clay-rich and organic-rich Cool to temperate, swampy regions OM-dominated soils Soils of tropical, humid climates Laterite (Oxisol) Lacks a B horizon high temperatures and rainfall so extensive leaching of Ca, Na, K, Mg and SiO2 during decomposition of silicate minerals mainly Fe and Al oxides (± quartz, kaolinite, organic matter). In some regions Fe and Al oxides are concentrate to form ore deposits. Bauxite: Al ore with Al2O3>30% Bauxite main source of Aluminium Soils of arid and semi- arid climates Aridisol Low content of OM. Leaching of soluble ions is limited. Carbonate crusts may form in the B horizon. Known as caliche (or calcrete)