Animal Physiology Part 2 Quiz (PDF)
Document Details
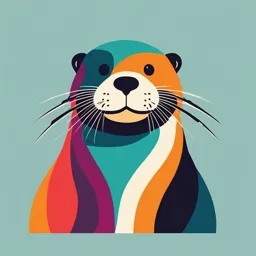
Uploaded by WellBehavedJasper9343
Queen's University
Tags
Summary
This document provides an overview of respiratory gases, gas exchange, and transport in animals. It discusses adaptations for gas exchange in different environments, and the principles underlying gas exchange, including factors influencing the rate of gas diffusion. This document also touches on the differences between water and air as respiratory media and general characteristics of oxygen and carbon dioxide.
Full Transcript
Lectures 1A/B (CH11) – Respiratory Gases, Gas Exchange and Transport Respiratory gases - oxygen and carbon dioxide Much less O2 available to organisms in water vs in air ○ Early earth -> atmospheric O2 levels low ○ Photosynthetic bacteria (cyanobacteria) began produci...
Lectures 1A/B (CH11) – Respiratory Gases, Gas Exchange and Transport Respiratory gases - oxygen and carbon dioxide Much less O2 available to organisms in water vs in air ○ Early earth -> atmospheric O2 levels low ○ Photosynthetic bacteria (cyanobacteria) began producing O2 as a byproduct creating O2 rich environments Adaptations required to transition to extract O2 directly from air because water and air have different properties ○ Air contains much higher O2 concentrations than water ○ Air is less dense than water – easier to move, challenges in maintaining moist surface for gas exchange Evolution of respiratory strategies – to exploit richer O2 supply in air, allowing animals to move onto lab ○ Lungs (terrestrial animals): maximize SA for gas exchange ○ Tracheal systems (insects): deliver O2 directly to tissues ○ Specialized skin (amphibians): cutaneous respiration General characteristics of O2 and CO2 Atmosphere today: O2 (20.95%), Nitrogen (78.08%), CO2 (0.0412%) Evolutionary advantage – presence of high [O2], aerobic metabolism releases much more energy than anaerobic metabolism Partial Pressure of Gas – % of gas in atmosphere x atmospheric pressure ○ Difference in partial pressure of a gas across a surface will determine how much gas will diffuse across that surface Less O2 at high altitudes – atmospheric pressure decreases with altitude ○ %O2 stays the same with altitude change, atm pressure and partial pressure of O2 (PO2) decreases Water Vs Air as Respiratory Media Henry’s Law – solubility of a gas in water is based on how much gas is present above the water (pressure) and how well the gas mixes with the water (solubility) ○ The amount of a gas that dissolves on water depends on: The gas pressure above water – if pressure of gas increases, more will dissolve The gas ability to dissolve in water (solubility) ○ Solubility coefficient – number that tells how easily a gas dissolves in water Higher solubility coefficient will dissolve more easily ○ Solubility coefficient of co2 and o2 in water are different from each other Solubility of o2 in water is lower than of co2 Both decrease with increasing temp At same partial pressure, [o2] more exchange) ○ The Krogh’s diffusion constant Depends on gas + type of surface Shows how easily air exchanges via blood, air, muscles ○ Thickness of exchange surface – thinner = faster Ficks Law of Diffusion describes relationship between 4 factors: MO2=KO2*[A(P1O2-P2O2)/d] ○ MO2 – rate of O2 transfer or consumption (in some cases) ○ KO2 – Krogh’s diffusion constant for O2 Different for different gases/medias ○ A – area of barrier ○ P1O2-P2O2 – PO2 differences across the barrier ○ D – thickness Skin breathers – increases area available for gas exchange (A) to increase oxygen diffusion SA/V Ratio (challenge for respiration) ○ Ratio of surface area (for gas exchange) to volume (consumes O2) gets smaller as animal gets larger ○ Issue for gas exchange across body surface – can influence shape of animal ○ For any given volume SA of flat object > than for a sphere – explains the shape of many animals that rely on gas exchange across skin Forced convection (def^n): bulk of movement of a fluid such as water, air, blood, usually involves the energy expenditure by pumping mechanisms (lungs, heart etc) ○ In many animals present on both sides of gas exchange surface ○ Larger, more active animals it is provided by respiratory/circulatory systems Fick principle can be applied to both respiratory system and cardiovascular system ○ Respiratory – MO2 = Vm (C1O2-CeO2) Mo2 – rate of o2 consumption Vm – minute ventilation volume (air going in/out) C1O2-CeO2 – difference in O2 concentration between inspired water or air and expired water or air ○ Cardiovascular – MO2 = Vb (CAO2-CVO2) Vb – cardiac output (amount of blood heart pumping) (CAO2-CVO2) – difference in O2 concentration between arterial blood leaving the gas exchange organ and mixed venous blood from body Lectures 2A/B – Respiratory Systems (CH12) 4 basic type of gas exchangers Infinite pool gas exchanger (skin breather) ○ No pumping mechanism ○ Least effective, less control Countercurrent gas exchanger ○ Flow of water and blood are opposite directions ○ Most effective Ventilated pool gas exchanger (inside lungs) ○ Air is pumped in and out of sac-like lungs Cross Current Gas Exchanger ○ Air flows in one direction via sacs and tubes (parabronchi which are at right angles to blood vessels) ○ Blood flows at different angle ○ More effective than 1 direction or ventilated pool Gas Exchange in Water Water (medium where O2 tends to be eliminated) can be: ○ Hypoxic – low in O2 ○ Hyperoxic – high in O2 The rate of O2 transport across the skin of aquatic (skin) breathers is diffusion limited ○ PO2 of arterial blood passing through skin never catches up to PO2 of surrounding water Boundary layers limit gas exchange across skin of aquatic skin breathers ○ Different on outside of animal where there's a gradient until in water ○ A little water next to skin where PO2 is in middle ○ If animal moves, thickness of boundary layer can change The relative importance of gas exchange across the skin is affected by: ○ Motion of animal (impacts boundary layers) ○ Blood flow to the skin (shunting) ○ Species (eg. surface area of the skin may differ) ○ Developmental stage (eg. tadpole vs frog) – some aquatic animals metamorph into animals that breathe air Gas exchange across gills – countercurrent gas exchangers ○ Gill arch, buccal cavity, afferent and efferent blood vessels, lamellae, gill filaments, opercular cavity, water flow ○ Lamellae in fish gill shows flow of water and blood Water one direction, blood opposite Blood flow, water flood, efferent and afferent blood vessels, filament cut across If water and blood flowed in the same direction (concurrent) ○ Water starts with high Po2 magnitude, blood starts with low, both flow same direction, po2 meets in middle, gradient gets smaller, blood and water leaving gill If water and blood flowed in opposite directions (countercurrent) ○ Final PO2 of blood gets higher, maintains gradient and doesn't disappear Gas Exchange in Air More oxygen in air Most species are most exotic and live in areas where water becomes warm and hypoxic Sacropterygii (lobe finned fish) contains 3 species of (unique) lungfish – land vertebraes evolved from this group African lungfish survive the dry season by burrowing underground and air breathing until the rain comes Fick equation principals ○ If animal wants to breath air in hypoxic environment, need an exchange surface (like network of capillaries) ○ Common principals - Increased surface area, reduced diffusion distance, a lot of blood flow Amphibians redesign processes during metamorphosis ○ Aq water breathing when tadpoles --> air breathing when adults ○ Use multiple exchange surface areas Birds: breath in and out - goes in one direction around a circuit with air sacs and lungs Bats - thin membrane for gas exchange, high metabolic rate, light General Strategies for Air breathers: Organ for gas exchange – modified gills, regions of body like lining of gill chamber, mouth cavity, lungs Amphibians – loss gills, dependence on air Physical Properties between air and water – helpful to explain characteristics of air breathers At any given partial pressure of O2 (PO2) there's a greater concentration of oxygen in air vs water At any given rate of o2 consumption, air breathing animals ventilate their gas exchangers at a lower rate than water breathers ○ Not the only issue that affects ventilation but important Air less dense/viscous than water, energetic cost of ventilation for air breathers is less than that for water Capacitance coefficient of CO2 in water is 28x greater than that for O2, same in air ○ Results with partial pressure of CO2 lower in blood of water breathing animals compared to air breathing No significant variation of PO2 in air other than at altitude/poorly ventilated burrows Hypoxia (low environmental Po2) is more common in water Challenge of respiration in water is getting enough O2 Air does not provide structural support for gills Many terrestrial animals have evolved internal gas exchange structures (eg lungs) ○ Lungs - good surface, doesn’t collapse, retains water (good for hot/dry not using a lot of water across respiratory surface) Internal location is important for reducing water loss across respiratory surfaces Air Breathing Organs Fish ○ Related to 2 factors – aquatic hypoxia, increased demand for O2 cause by temp/activity ○ Convergent evoultion ○ Air breathing fish using air for supplemental o2 ○ Using gills to get rid of co2 ○ Obligate - need breath air all time, breath air even when in well oxygenated water ○ Facultative - breath under certain circumstances for o2, only breathe air when water is hypoxic, when temp is high, when active ○ 3 regions of body that evolved to form air breathing organs in modern air breathing fish Branchial (gill), opercular and pharyngeal cavities Gastrointestinal tract Air bladder ○ Climbing perch – includes lining of gill chambers and the labyrinthine organs (layered bony structures) attached to the gills arches Important fish groups ○ Ray-finned fish/actinopterygii (modern fish) ○ Lobe-finned fish/sarcopterygii (ancient fish) Land vertebrates evolved Lungfish ○ Important species in lobe finned fish group (Sarcopterygii) – Neoceratodus forsteri (Australia) Protopterus aethiopicus (Africa) Lepidosiren paradoxa (South America) ○ Unique double circulation where oxygen rich blood from the lungs and oxygen poor blood from the body are partially separated within the heart Amphibians and reptiles ○ Greater dependence on air as the source of O2 ○ Trend – loss of gills, development of more complex lungs General trend as we go from amphibians to reptiles to birds & mammals where important variables within the Fick equation are altered to improve gas exchange ○ General increase in surface area of the gas exchanger ○ Reduction in diffusion distances Mammalian Lungs – Ventilated Pool Gas Exchangers ○ A lot of branching in airways (bronchi and bronchioles) before the regions where gas exchange occurs ○ Areas where gas exchange occurs are acinar airways – includes respiratory bronchioles, alveolar ducts, alveolar sacs ○ Cross sectional area of human lung increases as inspired air reaches the respiratory zone ○ Alveolar capillary – thickness of barrier to diffusion of gases between the blood cells and air space ○ Terms Alveoli = blind ending sacs in the lungs where gas exchange actually takes place Anatomical dead space = the airways in the lungs that are just used to transport air (no real gas exchange). Tidal volume Functional residual capacity Vital capacity Residual volume Total lung capacity Bird Lungs – cross current gas exchangers ○ Number of air sacs associated with the lungs in birds ○ Air sacs are involved in the pattern of airflow, not directly involved in gas exchange ○ Gas exchange takes place in tissue surrounding the tertiary (para) bronchi ○ Most birds have an additional network of parabronchi (neopulmo) ○ Gas exchange region of the bird lung Secondary (dorso) bronchi Lumen of parabronchus Secondary (ventro) bronchi ○ Diffusion of oxygen occurs from lumen of parabronchi into the atria from which a network of fine air capillaries radiate ○ In birds, flow of air through respiratory system is in one direction ○ Breathing First breath in – air moves into air sac First breath out – air moves into lungs Second breath in – air moves into front air sac Second breath out – air leaves body Lecture 3A/B – Transport in Respiratory Systems (CH13) Blood has two main components: plasma and red blood cells RBC contain respiratory pigment, haemoglobin (Hb) ○ Increases the amount of O2 carried in the blood Oxygen equilibrium curve - plots the concentration of o2 at different partial pressures of o2, (PO2’s) ○ Haemoglobin increases the amount of O2 carried in blood Oxygen carrying capacity - The max amount of o2, that can be carried by a given volume of blood. Haematocrit - the concentration of red blood cells per unit volume of blood. Myoglobin (Mb) – respiratory protein (pigment) found in (aerobic) muscle ○ Some species found in over tissues – liver, gill, brain ○ Each molecule of Mb can bind with one molecule of O2 whereas a molecule of Hb can bind with 4 molecules of O2 The affinity of a respiratory pigment describes how easily it binds o2 ○ The affinity of Mb is much greater than that of Hb. ○ This makes sense when we consider the different functions of these respiratory pigments. Functions of Hb and Mb ○ Hb – bind o2 as blood passess through the gas exchange organ (PO2 is high), the release of O2 when blood passes through the tissues (Po2 lower) ○ Mb – provides a source of O2 in tissues for times when O2 is scare (eg exercise), helps facilitate diffusion of O2 within tissues ○ Additional O2 bound to myoglobin is one of the adaptations dividing mammals use to stay underwater so long Structure of respiratory pigments – haemoglobin (tetramer), myoglobin (monomer) Unique (cooperative binding) properties of Hb related to structure ○ Binding of the first molecule of O2 results in a conformational change that makes it easier to bind the next molecules of O2 and so on Large variation in sizes of vertebrae rbcs – mammalian rbcs lack a nucleus whereas all other vertebrates rbcs are nucleated Factors affecting the binding of O2 to respiratory pigments ○ important to understand cooperative binding of o2, to Hb, tense (T) and relaxed (R) states of Hb, why the O2, equilibrium curve for Hb a is sigmoid shape. ○ don't worry about Hill plots & invertebrate info Oxygen binding characteristics of Mb and Hb ○ O2 equilibrium curves – Myoglobin, haemoglobin, partial pressure of O2 in venous blood, partial pressure of o2 in arterial blood ○ Cooperative binding of O2 to Hb explains the sigmoid shape of the O2 equilibrium curve P50=the partial pressure of O2 where Hb is 50% saturated Bohr effect – reduction in O2 affinity of Hb caused by a reduction in pH or increase in CO2 ○ Reduction in O2 affinity is the same thing as an increase in P50 ○ The bohr effect helps with the unloading of O2 from Hb when blood flows through tissue ○ At any given Po2, a decrease in blood pH will reduce the amount of O2 bound to Hb (and o2 concentration in blood) ○ As o2 binds to Hb affinity reduces and shifts to the right Root effect – in some fish species, an increase in blood Co2 or decrease in blood pH will reduce the total O2 carrying capacity of the blood Hb in fish have a root effect (occurs in some fish species, not mammals/most other animals) ○ Root effect is important in O2 delivery to the retina and the swimbladder in some species Teleost fish have a swim bladder to stay buoyant in water column by putting gas in salt water to adjust buoyancy ○ In these tissues production of lactic acid (decrease in pH) and Co2 initiates the root effect, and a increase in blood Po2 (as a result of Hb releasing O2) ○ A countercurrent arrangement of capillaries in these tissues helps magnify the increase in Po2 and aids in O2 diffusion into these tissues Other factors that affect Hb:O2 affinity ○ Increasing temp reduces Hb:o2 affinity Environmental issue for fish ○ A challenge for respiring fish is getting enough O2 out of water ○ Aquatic hypoxia (lower than normal O2) is also a common environmental situation for many fish species Increasing environmental temperatures with climate change creates additional problems ○ Increase the O2 requirements of fish – fish are ectotherms so their body temp and metabolic rate increase with temp increases ○ O2 solubility in water decreases with increasing temperature ○ Hb:O2 affinity is reduced at higher temperatures, making it hard to extract o2 from environment (loads of O2 in blood makes it hard to extract O2) ○ Fish that cope with environments that can become hypoxic, increasing temperatures due to climate change will compound the problem Organic phosphates with RBC (eg BPG) also reduce Hb:O2 affinity ○ RBCs have external environment that has different pH’s that affect Hb:O2 affinity Embryonic/Foetal Hbs – have higher Hb:O2 affinity (lower P50) than adults Hbs, favouring O2 delivery to the embryo/foetus ○ Hb in embryo/fetus have higher affinity than adults, takes mothers blood Transport of Carbon Dioxide CO2 is transported in blood in 3 forms ○ A small amount is carried in solution (dissolved Co2) ○ Some is also carried in the form of carbamino compounds attached to haemoglobin ○ Most CO2 in the blood is in the form of bicarbonate (HCO3-) ions in plasma Reaction that hydrates Co2 with water and turns into bicarbonate CO2 is highly soluble in water and quickly forms carbonic acid (H2CO3-) that dissociates into a proton (H+) and bicarbonate (HCO3-) ○ Add co2 to blood will react with water making bicarbonate ○ Rate of reaction is slow and not catalyzed Forms of co2 transported in blood ○ Total carbon dioxide (all combined forms) , bicarbonate (most common), carbaminohemoglobin, dissolved in plasma Haldane Effect: deoxygenated Hb carries more Co2 than oxygenated Hb (Hb is bound to O2) Carbonic anhydrase (CA) is an enzyme found in RBCs and other tissues that catalyzes this reaction ○ In RBCs of almost every vertebrae Combined reactions involved in CO2 transport at the tissues and at the gas exchanger ○ Pick up O2 at exchange surface, get rid of Co2 or vice versa Reaction steps involved in co2 and o2 gas exchange surfaces of vertebrates – co2 transport in blood at tissues ○ CO2 diffuses into the plasma and red blood cells ○ Inside the rbcs, CO2, combines with H20 and forms HCO3- and H+. The enzyme, carbonic anhydrase (CA) inside rbcs catalyzes this reaction. ○ Most of the HCO3- created by this reaction moves to the plasma via an exchange protein that moves chloride (Cl-) in the opposite direction. ○ This anion exchange protein that exchanges HCO3- for Cl is also known as the Band 3 protein. ○ Most CO2 is transported in the plasma as HCO3- until the blood reaches the gas exchanger. ○ Some of the protons (H+) created by the CO2 hydration reaction (CO2+ H2o) contribute to the Bohr effect and combine with Hb, causing it to release o2 that diffuses into the tissues. ○ Additional o2 bound to Hb also diffuses into the tissues without requiring the involvement of the Bohr effect. Steps in co2 transport at the gas exchanger ○ When the blood reaches the exchanger, CO2 diffuses down its concentration gradient from the rbcs and plasma and into the water (gills). ○ Some of the Hb releases (Bohr) protons (H+) when the Hb combines with o2 ○ HCO3- moves into the rbc via the anion exchange protein, combines with H+ to form CO2, which continues to diffuse down its concentration gradient to the water. ○ The reaction in step 3 is catalyzed by CA within the rbcs. Lecture 4 – Cardiovascular Systems (CH14) Invertebrates (except cephalopods) have “open” circulatory systems Vertebrates have “closed” circulatory systems Important features of the mammalian heart ○ Completely divided with 2 atria and 2 ventricles ○ Left side (atria and ventricles) receives oxygenated blood from the lungs and pumps around the body ○ Right side (atria and ventricles) receives deoxygenated blood from the body and pumps it to the lungs ○ Valves between the atria and ventricles, and ventricles and aorta prevent backflow and keep blood moving in one direction ○ Changes in pressure open and close the valves (like a one way door system) as the blood is moved around the heart. ○ Blood leaving the heart to the body (left side) is under high pressure. ○ Blood leaving the heart to the lungs (right side) is under low pressure Systole – contraction phase of the ventricle(s) when blood is pumped out of the heart Diastole – relaxation phase of the ventricle when blood returns to the heart Structure of blood vessels in vertebrates – structure of arteries and veins reflect the differences in blood pressure in these vessels The compliance and elasticity of the major arteries maintain an energy efficient relationship between the pressure generated by the heart and the flow of blood around the body. Difference in structure of vessels in different sections of the circulatory system make sense when their function & the pressures they are exposed to are considered Pressure will decrease as the distance from the heart increases on the arterial side Pressure lowers overall on the venous side Properties change as vessels get further from the heart and pressures decrease More muscle and elasticity and larger diameter vessels on arterial vs venous side Capillaries only consist of endothelial cells inside a basement membrane Where exchange takes place (gases, ions etc) between organs/tissues and the circulating blood permeability (& structure) of capillaries varies depending on tissues & exchange requirements Capillary beds have sphincters to control blood flow ○ Vasodilation vs vasoconstriction Blood pressure falls along the circulatory system of mammals Evolution of the vertebrae heart and circulatory system Animals began to tap into the air supply that was rich in oxygen they developed hearts and circulatory systems that could best exploit that Trend toward being able to separate blood flow into a circuit with high pressure and well oxygenated blood to the body and another circuit with low pressure for deoxygenated blood to go through gas exchange organs – maximizes gas exchange across thin surfaces At a finer level, many strategies to shunt blood to gas exchange surfaces and then shunt blood away from these areas when not needed – skin of amphibs, air breathing organ in air breathing fish Heart and circulatory systems in entirely aquatic fish (eg trout) Completely aquatic fish have a heart with 1 atrium and 1 ventricle & a "single circulation” ○ 2 other chambers in series (sinus venosus and bulbus arteriosus) Blood goes through heart before going to gas exchange organ Amphibians 2 artia, 1 ventricle, additional anatomical features that help to partially separate blood between left and right sides Deoxygenated blood can be directed to gas exchange surfaces Oxygenated blood can go to other parts of the body that consume o2 Shunts can redirect blood to the most appropriate gas exchange surface (lungs vs skin) Reptiles Most reptiles (except crocodiles) have a heart with 2 atria and a single ventricle Ventricle muscular ridges help to partially separate o2 rich and low o2 blood Crocodiles Exception in reptiles because they have a completely divided ventricle Foramen of panizza – connection between the arterial and venous side of the aortas leaving the heart that likely allows shunting when needed Lungfish Atrium and ventricle are partially divided allowing some separation of blood into 2 sides of the heart Similar to amphibians the partially separated heart of lungfish creates a functional separation of blood into a deoxygenated circuit going to the gas exchange organs and a o2 rich circuit going to the other parts of the body Shunts in lungfish circulation can direct blood towards the most appropriate gas exchange surface ○ Both lungfish and amphibians ventilate their lungs intermittently and the perfusion of the lungs with blood matches their ventilation in both groups Lungfish, other air breathing fish, and amphibians are all "bimodal" breathers, with more than one gas exchange surface. ○ In these animals, it makes sense that they have strategies (eg shunting) to send blood to areas that make the most sense at any given time. (eg just after an air breath, during diving etc) Lecture 5A/B – Temperature and the Principles of Heat Exchange (CH8/9) Fish solubility in water ○ O2 solubility higher, fish metabolism lower ○ Choice of hot/cold hypoxic environment, fish would choose cold water Q10– the extent to which a reaction rate changes with a 10C change in temperature LT50 – the lethal temperature at which half the animals die Eurythermal – animals with a wide temperature range Stenothermal – animals that can only tolerate a narrow temperature range Homeothermy – animals that seem to maintain their body temperatures at constant levels Poikilothermy – animals that show considerable variability in their body temperature often matching the environmental temperature (fish, amphibians, reptiles) Heterothermy – animals that go through daily or seasonal variations in body temperature Regional heterothermy – animals that allow the temperature of parts of their body to change with respect to core temperature Temperature limits to life – possible reasons ○ Negative impact of temperature on protein structure ○ Different Q10 values along a sequence of different reactions ○ Negative impact of temperature on the lipid bilayer Temperature regulation in ectotherms Animals that need to obtain heat from outside their body for body temperatures to be raised are ectotherms Behavioral thermoregulation – many ectothermic animals change their behavior to influence their body temperatures ○ When conditions in environment are unfavorable they can move or adjust their behavior to adjust to temp Terrestrial ectotherms can adjust their rate of heat uptake and heat loss by the way they position themselves in their environment ○ Behavioral – position themselves to get heat from sun at right angle Many aquatic ectotherms move to places in their environment with temperatures they prefer ○ Sometimes to get enough o2 Climate change – changes fish distribution to find temperatures that suit them ○ Movements of radio-tagged skipjack tuna follow warm water (28-29C) ○ Always try to move to maintain the same temp or preferred temp range Terrestrial ectotherms – behavioral thermoregulation often involves shuttling back and forth between sunlight and shade ○ Move in/out of sun/burrows to get desired temp ○ Temp of the substrate can result in additional heat gain/loss by conduction across the body surface in contact with the substrate Body size influences behavioral thermoregulation of some reptiles ○ Larger animals have lower SA/V traditions which reduces rate of heat loss and gain Nocturnal lifestyle avoids high temperatures of hot deserts ○ A lot of reptiles ○ Some skinks forage at night during the hottest times of the year ○ Many small ectotherms adopt nocturnal lifestyle and avoid high temps by burrowing during the day (ex. ants) Peripheral (external) thermoreceptors – pass on information to an animal about the thermal condition of their body surface ○ Can block receptor with a physical block, drugs, or remove to see how they behave ○ Best described in invertebrates but fish, amphibians and reptiles have them too Internal thermoreceptors – convey information about tissue temperature ○ Associated with thermosensitive neurons in the base of the hypothalamus ○ Hypothalamic lesions disrupt behavioral thermoregulation in ectotherms (eg goldfish, desert iguanas) Physiological regulation of heat exchange in ectotherms Changing albedo affects heat uptake of some ectotherms ○ Albedo – brightness of animal ○ Ectotherms that sit in the sun can change color ○ When other things (size, shape and posture) are similar, animals with high albedo (lightness of color) or reflectance, heat up more slowly than those with low albedo (darker) ○ Ex. chameleons become lighter at higher body temperatures and this reduces the rate of further warming during basking ○ Reflectance increases in a chameleon at 35C compared to 20C Camo and temperature regulation The change in albedo can be seen as dramatic color differences when temperature changes ○ Dark – trying to get heat, light – doesn't need to gain heat Some ectotherms control heat uptake and loss by regulating blood flow to surface tissues ○ Ex. iguanas spending the day foraging in the ocean and then basking in the sun They heat up faster then they cool down Adjust mostly through heart rate Heat loss is reduced by the fact that heart rate and blood flow to surface tissues is lower than expected when the animal is cooling Control over heart rate and circulation advantageous for what it's trying to achieve – heating or cooling The hysteresis of heart rate seems to be under control of the autonomic nervous systems Basking Reptiles basking – able to increase brain temp faster than body by adjusting circulation During basking in lizards the head temperature initially increases faster than the core body temp ○ Brain temp can be 2–4C higher than body temps during basking ○ When basking, heat retention in the head occurs by a countercurrent arrangement of blood vessels Countercurrent arrangement – multiples heat cause blood vessel arrangement that's going to the brain and disables itself so it doesn't go beyond ○ At higher temperatures, the countercurrent mechanism is uncoupled to prevent overheating of the brain Respiratory cooling Occurs by evaporative water loss from upper airways, mouth and tongue during normal breathing, panting or by gaping with an open mouth with the tongue hanging out Numerous reptiles pant to reduce body temperature once brain/body temps rise above certain point Main priority of respiratory cooling (panting) appears to regulate brain temperature Surviving the cold Many amphibians and reptiles spend the cold winter months in a state of hibernation During inactivity period they let their body temperatures follow that of the environment Many species of frogs and turtles over winter under the ice in cold but unfrozen water (cutaneous respiration) Key factors to survive winter ○ Sufficient energy stores for the period of starvation ○ Enough oxygen Energy stores they have going into winter determines if they will survive the winter Amphibians can obtain sufficient o2 across their skin in aquatic environments in winter ○ Some turtles can also obtain enough o2 via cutaneous routes in winter The relationship between metabolic rate and temperature indicates that there is a metabolic suppression at very low temperatures in some reptiles ○ Metabolic rate decreases even if the temp is cold, do things that turn off processes, energy saved = metabolic supression Freeze Tolerance Many invertebrates and some vertebrate species can tolerate freezing of their body fluids at cold temperatures Formation of ice crystals inside cells would rupture cell membranes and be catastrophic In freeze-tolerant species, freezing only occurs in extracellular fluids Restricting ice formation to the extracellular fluid protects the cells in freeze tolerant ectotherms ○ Ice starts in extracellular fluid, osmotic concentration increases inside cell and lowers freezing point Freeze tolerant animals can survive with 65% of their total body water in a frozen state Some species enhance extracellular freezing by synthesizing ice nucleating agents Ice nucleating agents also control the growth of ice in the extracellular fluid Cryoprotectants Synthesis of “cryoprotectants” also helps animals tolerate freezing These compounds reduce the freezing point of the extracellular fluid (slowing the rate of ice formation) Addition of cryoprotectants to the intracellular fluids also limits cell shrinkage by reducing water loss (increased intracellular osmotic concentration) Freeze Avoidance Many species have antifreeze compounds that help them avoid freezing Colligative antifreezes – lower the freezing point of body fluids due to the increase in total concentration of solutes ○ By adding lots of solutes, decreases freezing temperature Non- colligative antifreeze compounds – have chemical properties that suppress the growth of ice crystals ○ Control and suppress formation of ice crystals Rainbow smelt synthesize glycerol as a colligative antifreeze wehn temperature drops In animals that synthesize non-colligative antifreeze compounds there is a thermal hysteresis (difference) between the freezing and melting point of their plasma Hysteresis results from adsorption of molecules of antifreeze to tiny ice crystals which inhibit their growth (adsorption-inhibition effect – control way ice crystals grow) Antifreeze compounds are an example of convergent evolution – different compounds play a role in different groups of fish and insects ○ Arctic and subarctic fish produce antifreeze seasonally Prepared by synthesizing compounds during coldest winter months Triggered by ambient temperature and photoperiod