Campbell Essential Biology PDF
Document Details
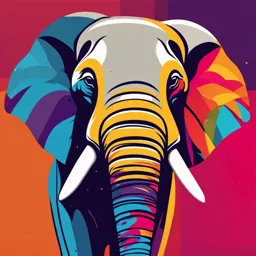
Uploaded by SmootherSacramento
KMUTNB
Tags
Summary
This textbook provides a foundational overview of biology. It covers topics from introduction to the scientific process, to chemistry and macromolecules. The text also connects biological concepts to real-world issues and applications.
Full Transcript
Chapter 1: Introduction: Biology Today This chapter introduces the fundamental concepts of biology, emphasizing its relevance to everyday life and establishing a framework for further exploration. **Why Biology Matters:** Biology, the scientific study of life, is interwoven into the fabric of our...
Chapter 1: Introduction: Biology Today This chapter introduces the fundamental concepts of biology, emphasizing its relevance to everyday life and establishing a framework for further exploration. **Why Biology Matters:** Biology, the scientific study of life, is interwoven into the fabric of our existence. From understanding the names of organisms (taxonomy) to making everyday decisions, we constantly engage with biological principles. The search for life beyond Earth, like the Mars rover mission, highlights our innate curiosity about the living world. **Biology and Society:** Biology\'s impact extends to diverse societal issues, including medicine, public health, and environmental concerns. Examples such as medical uses of radiation, flu vaccines, and the human microbiome illustrate this broad reach. Throughout the book, \"Biology and Society\" essays within each chapter will connect biological concepts to real-world applications. **The Scientific Study of Life:** - **The Process of Science:** Biology, as a science, relies on inquiry -- the search for information and explanations. Two main approaches characterize scientific inquiry: - **Discovery science:** Primarily focuses on describing nature through verifiable observations and measurements (data). Darwin\'s observations of diverse species and Goodall\'s study of chimpanzee behavior are examples. - **Hypothesis-driven science:** Seeks to explain nature. It employs the scientific method, a systematic process involving: 1. **Observation:** Noticing a phenomenon or problem. 2. **Question:** Formulating a testable inquiry about the observation. 3. **Hypothesis:** Proposing a tentative explanation (a testable prediction) for the observation. 4. **Prediction:** Stating the expected outcome if the hypothesis is correct (often using \"if\...then\" logic). 5. **Experiment:** Designing and conducting a test to evaluate the prediction. 6. **Results:** Analyzing the data collected from the experiment. 7. **Revise/Repeat:** Modifying the hypothesis or designing new experiments based on the results. - **Theories in Science:** In science, a theory is not a mere guess. It is a comprehensive explanation supported by substantial evidence, broader in scope than a hypothesis. Theories, like the theory of evolution by natural selection, provide a framework for understanding a wide range of phenomena and generating new hypotheses. **The Nature of Life:** - **Properties of Life:** Living things share several key properties: 1. **Order:** Exhibit complex but organized structures. 2. **Regulation:** Maintain internal environment within suitable limits. 3. **Growth and Development:** Increase in size and complexity controlled by DNA. 4. **Energy Processing:** Acquire and use energy to power life\'s activities. 5. **Response to the Environment:** React to changes in surroundings. 6. **Reproduction:** Produce offspring of the same kind. 7. **Evolution:** Change over time as a population. - **Life in Its Diverse Forms:** The 1.8 million identified species on Earth exhibit remarkable diversity. Taxonomy organizes species into progressively broader groups, forming a hierarchical classification. The broadest units are: 1. **Three Domains:** Bacteria, Archaea (both with prokaryotic cells), and Eukarya (with eukaryotic cells). 2. **Kingdoms within Eukarya:** Plantae, Fungi, Animalia (all multicellular), and Protists (mostly unicellular). **Major Themes in Biology:** Five unifying themes recur throughout the study of biology: 1. **Evolution:** The core theme of biology. The theory of evolution by natural selection explains the unity and diversity of life. Adaptations arise through natural selection, where organisms with traits better suited to their environment have greater reproductive success. Artificial selection, the selective breeding of domesticated plants and animals, provides evidence for this process. 2. **Structure/Function:** The form of an object, whether a molecule or body part, is closely related to its function. Examples include the branched structure of lungs facilitating gas exchange and the concave shape of red blood cells maximizing oxygen diffusion. 3. **Information Flow:** Life depends on the transmission and use of information. Within cells, genes (DNA sequences) store information that directs protein synthesis. Information flow also coordinates cellular activities during development and maintains internal conditions within organisms. 4. **Energy Transformations:** Life requires energy. Ecosystems are primarily powered by solar energy. Photosynthesis captures this energy and converts it into chemical energy (stored in sugars), which is then used by consumers. Energy flows through ecosystems, while matter is recycled. Cellular respiration, the breakdown of fuel molecules to release energy, powers cellular work. 5. **Interconnections within Systems:** Life exists at many levels of organization, from molecules to the biosphere. Emergent properties arise at each level due to the interactions of parts within a system. For example, the properties of a cell emerge from the interactions of its molecules. The interconnectedness of biological systems is evident in phenomena like global climate change affecting ecosystems and human health. Chapter 2: Essential Chemistry for Biology ------------------------------------------ This chapter explores the fundamental principles of chemistry that are crucial for understanding biological processes. It explains how the structure of atoms and molecules dictates their interactions and how these interactions underpin the properties of water, a substance essential for life. **Why Chemistry Matters:** Life at its core is an intricate dance of chemical reactions. Understanding basic chemistry is essential for grasping biological concepts, from the effects of radiation on cells to the importance of balanced diets. Simple substances can combine to form complex ones with emergent properties, like table salt from sodium and chlorine. The acidity of biological fluids, like stomach acid and lemon juice, illustrates chemistry\'s role in everyday life. **Some Basic Chemistry:** - **Matter: Elements and Compounds:** Matter, anything that occupies space and has mass, is made of elements. Elements are substances that cannot be broken down further by chemical reactions. Compounds are substances formed by the combination of two or more elements in fixed ratios. Essential elements are necessary for life. - **Atoms:** Atoms are the smallest units of matter that retain the properties of an element. Atoms are composed of subatomic particles: - **Protons:** Positively charged, determine the element (atomic number). - **Neutrons:** No charge, isotopes of an element differ in the number of neutrons. - **Electrons:** Negatively charged, participate in chemical reactions. - **Isotopes:** Isotopes are variants of an element with different numbers of neutrons and thus different mass numbers. Radioactive isotopes have unstable nuclei that decay spontaneously, emitting radiation. Radiometric dating utilizes the decay of radioactive isotopes to estimate the age of fossils. - **Chemical Bonding and Molecules:** Atoms are linked together by chemical bonds to form molecules. Three types of bonds are important in biology: - **Ionic bonds:** Formed by the attraction between oppositely charged ions (atoms that have gained or lost electrons). *Example:* Table salt (NaCl) is formed by an ionic bond between Na⁺ (positive) and Cl⁻ (negative). - **Covalent bonds:** Formed by the sharing of electrons between atoms. These are strong bonds and form molecules. A double bond involves sharing two pairs of electrons. *Example:* Water (H₂O) is formed by covalent bonds between two hydrogen atoms and one oxygen atom. - **Hydrogen bonds:** Weak attractions between polar molecules. In a water molecule, the oxygen atom attracts electrons more strongly than the hydrogen atoms, creating a polar molecule with slightly positive and negative regions. Hydrogen bonds form between the slightly positive hydrogen of one water molecule and the slightly negative oxygen of another. **Water and Life:** Water\'s unique life-supporting properties stem from its molecular structure and hydrogen bonding: - **Cohesion:** Water molecules stick together due to hydrogen bonds. This allows for surface tension (the resistance of the surface of a liquid to stretching or breaking) and the transport of water against gravity in plants. - **Moderation of Temperature:** Water can absorb and release a large amount of heat with only a slight change in its own temperature. This is due to water\'s high specific heat (the amount of heat needed to raise the temperature of 1 gram of a substance by 1°C). Evaporative cooling (as in sweating) also helps moderate temperature. - **Ice Floating:** Ice floats because it is less dense than liquid water. This is because hydrogen bonds in ice hold water molecules farther apart in a spacious crystal structure. This property insulates bodies of water, preventing them from freezing solid. - **Water as the Solvent of Life:** Water is a versatile solvent, dissolving many substances due to its polarity. A solution is a homogeneous mixture of two or more substances: - **Solvent:** The dissolving agent (e.g., water). - **Solute:** The substance being dissolved (e.g., sugar or salt). - **Aqueous solution:** A solution in which water is the solvent. - **Acids, Bases, and pH:** A small percentage of water molecules break apart into hydrogen ions (H⁺) and hydroxide ions (OH⁻). - **Acid:** A substance that releases H⁺ into a solution. - **Base:** A substance that removes H⁺ from a solution. - **pH scale:** Measures H⁺ concentration; ranges from 0 (most acidic) to 14 (most basic). Each pH unit represents a tenfold change in H⁺ concentration. - **Buffers:** Minimize changes in pH by accepting or donating H⁺. **Radioactivity (Evolution Connection):** - **Radioactivity as an Evolutionary Clock:** Radioactive decay, the breakdown of radioactive isotopes, can be used to determine the age of fossils through radiometric dating. The half-life of a radioactive isotope is the time it takes for 50% of the atoms in a sample to decay. This information helps establish the chronological order of fossils and provides insights into the evolutionary history of life. Chapter 3: The Molecules of Life -------------------------------- This chapter explores the large molecules essential for life, also known as macromolecules. It details their structure, function, and importance in various biological processes, connecting them to everyday examples like the food we eat. **Why Macromolecules Matter:** From the sugar in your coffee to the glycogen stored for a marathon, macromolecules play crucial roles in our lives. They determine the diversity of life, from mosquitos to elephants, even though their basic DNA structure is nearly identical. Even the melting of chocolate is dictated by the structure of its fat molecules. **Organic Compounds:** - **Carbon Chemistry:** Carbon\'s ability to form four covalent bonds with various atoms, including other carbon atoms, allows it to create diverse carbon skeletons. These skeletons can vary in length, branching, and the presence of double bonds, leading to an immense variety of organic molecules. Functional groups, specific groups of atoms attached to carbon skeletons, participate in chemical reactions and give organic molecules their unique properties. *Example:* Hydroxyl group (-OH) in alcohols, Carboxyl group (-COOH) in proteins. - **Giant Molecules from Smaller Building Blocks:** Large biological molecules (macromolecules) are often polymers, long chains of smaller molecular units called monomers. - **Dehydration reaction:** Monomers are linked together through dehydration reactions, where a water molecule is removed. - **Hydrolysis:** Polymers are broken down into monomers through hydrolysis, where a water molecule is added to break the bond between monomers. **Large Biological Molecules:** Four classes of macromolecules are essential for life: 1. **Carbohydrates:** Carbohydrates include sugars and polymers of sugars. - **Monosaccharides:** Simple sugars (monomers of carbohydrates). *Examples:* Glucose (C₆H₁₂O₆), fructose (an isomer of glucose). - **Disaccharides:** Double sugars formed by joining two monosaccharides through a dehydration reaction. *Examples:* Lactose (glucose + galactose), sucrose (glucose + fructose), maltose (glucose + glucose). - **Polysaccharides:** Long chains of monosaccharides. - **Storage polysaccharides:** Store sugar for later use. *Examples:* Starch (in plants), glycogen (in animals). - **Structural polysaccharides:** Provide structural support. *Examples:* Cellulose (in plant cell walls), chitin (in insect exoskeletons and fungal cell walls). 2. **Lipids:** Lipids are hydrophobic (water-fearing) molecules. - **Fats (triglycerides):** Composed of glycerol and three fatty acids joined by dehydration reactions. - **Saturated fatty acids:** Have no double bonds in their carbon skeleton; tend to be solid at room temperature (e.g., animal fats). - **Unsaturated fatty acids:** Have one or more double bonds; tend to be liquid at room temperature (e.g., plant and fish oils). - **Trans fats:** A type of unsaturated fat formed by hydrogenation; associated with health risks. - **Steroids:** Lipids with a carbon skeleton containing four fused rings. *Examples:* Cholesterol (component of cell membranes), testosterone, estrogen (sex hormones). 3. **Proteins:** Proteins are polymers of amino acids, linked by peptide bonds. - **Amino acids:** Monomers of proteins; consist of a central carbon atom bonded to an amino group (-NH₂), a carboxyl group (-COOH), a hydrogen atom, and a variable side chain (R group). - **Polypeptides:** Chains of amino acids linked by peptide bonds. - **Protein shape:** A protein\'s function depends on its specific three-dimensional shape, determined by the amino acid sequence and interactions between the side chains. Denaturation is the loss of a protein\'s shape and thus its function due to changes in temperature, pH, or other factors. 4. **Nucleic Acids:** Nucleic acids store and transmit genetic information. - **DNA (deoxyribonucleic acid):** Stores genetic information; a double helix made of two polynucleotide strands. Each nucleotide consists of a deoxyribose sugar, a phosphate group, and a nitrogenous base (adenine, guanine, cytosine, or thymine). Base pairing: A with T, G with C. - **RNA (ribonucleic acid):** Various types of RNA involved in protein synthesis; single-stranded. Each nucleotide consists of a ribose sugar, a phosphate group, and a nitrogenous base (adenine, guanine, cytosine, or uracil). - **Gene:** A specific segment of DNA that codes for the amino acid sequence of a polypeptide. Gene expression involves transcription (DNA to RNA) and translation (RNA to protein). Lactose intolerance is used as a running theme throughout the chapter to illustrate the interplay of different macromolecules (lactase enzyme, lactose sugar) and their impact on human health. The chapter also touches on the genetic basis for lactose intolerance, demonstrating the connection between genes (DNA) and proteins. Chapter 4: A Tour of the Cell ----------------------------- This chapter explores the microscopic world of cells, detailing their structure and function. It highlights the differences between prokaryotic and eukaryotic cells and examines the organelles that carry out the various functions of eukaryotic cells. The chapter also touches on the evolutionary connections related to cell structure and function. **Why Cells Matter:** Cells are the fundamental units of life. Everything an organism does, from moving to thinking, happens at the cellular level. Understanding cell structure is crucial for understanding how life works, from the effects of caffeine on plants to the devastating consequences of structural failures within cells, like a collapsing house. **The Microscopic World of Cells:** - **Microscopes:** Most cells are too small to be seen with the naked eye, requiring microscopes for visualization. - **Cell theory:** States that all living things are composed of cells, and all cells come from pre-existing cells. **The Two Major Categories of Cells:** 1. **Prokaryotic cells:** Found in bacteria and archaea. They are smaller and simpler than eukaryotic cells, lacking a nucleus and other membrane-bound organelles. They have a nucleoid region (where DNA is located), ribosomes, a plasma membrane, a cell wall, and sometimes a capsule, pili, and flagella. 2. **Eukaryotic cells:** Found in protists, plants, fungi, and animals. They are larger and more complex than prokaryotic cells, containing a nucleus and other membrane-bound organelles. Both prokaryotic and eukaryotic cells share features like a plasma membrane, cytoplasm, chromosomes (carrying genes made of DNA), and ribosomes. **An Overview of Eukaryotic Cells:** Eukaryotic cells are compartmentalized by membranes, with different organelles carrying out specialized functions. - **Cytoplasm:** The region between the plasma membrane and the nucleus. Contains the cytosol (a jellylike fluid) and various organelles. - **Plasma membrane:** The outer boundary of the cell; regulates the passage of materials into and out of the cell. **Organelles:** - **The Nucleus and Ribosomes: Genetic Control of the Cell:** - **Nucleus:** Contains most of the cell\'s DNA, organized into chromosomes. The nucleus is surrounded by the nuclear envelope, a double membrane with pores that regulate the passage of materials. The nucleolus, located within the nucleus, is the site of ribosome production. - **Ribosomes:** Responsible for protein synthesis. Ribosomes can be free in the cytoplasm or bound to the endoplasmic reticulum (ER). - **How DNA directs protein production:** DNA directs protein synthesis through a process involving messenger RNA (mRNA). mRNA carries genetic instructions from the DNA in the nucleus to the ribosomes in the cytoplasm. - **The Endomembrane System: Manufacturing and Distributing Cellular Products:** - **Endoplasmic reticulum (ER):** A network of interconnected membranes. - **Rough ER:** Studded with ribosomes; involved in protein synthesis and membrane production. - **Smooth ER:** Lacks ribosomes; involved in lipid synthesis, detoxification, and other metabolic processes. - **Golgi apparatus:** Modifies, sorts, and packages proteins and lipids for storage or transport. - **Lysosomes (animal cells):** Contain digestive enzymes; break down macromolecules and worn-out organelles. - **Vacuoles:** Large sacs of membrane. Types include: - **Food vacuoles:** Involved in ingestion and digestion. - **Contractile vacuoles:** Pump out excess water from cells. - **Central vacuoles (plant cells):** Store nutrients, pigments, and toxins; contribute to cell growth. - **Energy Transformations: Chloroplasts and Mitochondria:** - **Chloroplasts (plant cells):** Sites of photosynthesis, converting light energy into chemical energy. Chloroplasts have a double membrane, stroma (fluid), and grana (stacks of thylakoids). - **Mitochondria:** Sites of cellular respiration, generating ATP from the breakdown of organic molecules. Mitochondria have a double membrane, cristae (infoldings of the inner membrane), and matrix (fluid). - **The Cytoskeleton: Cell Shape and Movement:** - **Cytoskeleton:** A network of protein fibers that provides structural support, maintains cell shape, and aids in movement. Components include microtubules, microfilaments, and intermediate filaments. - **Cilia and Flagella:** Microtubule-containing extensions from some cells that aid in movement. Cilia are short and numerous, while flagella are longer and often occur singly. **Humans Versus Bacteria (Evolution Connection & Process of Science):** - **Antibiotics target bacterial cell structures:** Antibiotics exploit differences between prokaryotic and eukaryotic cells to selectively kill bacteria without harming human cells. Examples include antibiotics that target bacterial ribosomes or cell walls. - **What Makes a Superbug?** (Process of Science) The development of antibiotic resistance in bacteria, like MRSA, highlights the importance of cell structure in disease and treatment. - **The Evolution of Bacterial Resistance:** (Evolution Connection) The evolution of antibiotic resistance illustrates how natural selection acts on variations within a population (bacteria) in response to environmental pressures (antibiotics). - **The Origin of Membranes:** (Evolution Connection) Membranes are fundamental to life. Experiments suggest that phospholipids, the key components of membranes, could have self-assembled into simple membranes in the early Earth environment, paving the way for the first cells. Chapter 5: The Working Cell --------------------------- the dynamic processes within cells, focusing on energy transformations, enzyme activity, and the movement of materials across cell membranes. It connects these cellular functions to everyday experiences like exercise and food preservation. It also touches on the application of these principles in nanotechnology. **Why Cellular Functions Matter:** Cellular functions are essential for life. Understanding how cells work explains everything from the effects of nerve gas (which cripples a vital enzyme) to how we preserve food using osmosis. Even everyday activities like eating pizza and then needing to exercise to burn off the calories involve cellular processes. **Some Basic Energy Concepts:** - **Conservation of Energy:** Energy is the capacity to cause change. It cannot be created or destroyed, only converted from one form to another. - **Kinetic energy:** The energy of motion. - **Potential energy:** Stored energy due to an object\'s location or structure. - **Chemical energy:** Potential energy stored in the arrangement of atoms within molecules. - **Heat:** A type of kinetic energy contained in the random motion of atoms and molecules; a byproduct of all energy conversions. - **Entropy:** A measure of disorder or randomness. Energy conversions increase entropy. - **Chemical Energy:** The energy stored in the arrangement of atoms in molecules, released during chemical reactions. Cellular respiration breaks down fuel molecules, releasing their chemical energy to produce ATP. - **Food Calories:** A calorie (cal) is the amount of energy needed to raise the temperature of 1 gram of water by 1°C. Kilocalories (kcal, or Calories) are used to measure the energy content of food. **ATP and Cellular Work:** - **ATP (adenosine triphosphate):** The cell\'s energy currency. ATP powers cellular work by transferring a phosphate group to other molecules, energizing them. ATP consists of adenosine and a tail of three phosphate groups. The crowding of negative charges in the phosphate tail contributes to ATP\'s potential energy. - **ADP (adenosine diphosphate):** Formed when ATP loses a phosphate group, releasing energy. - **The ATP Cycle:** ATP is continuously used and regenerated in cells. Cellular respiration provides the energy to convert ADP back to ATP. **Enzymes:** - **Enzymes:** Biological catalysts that speed up chemical reactions by lowering the activation energy (the energy needed to start a reaction). Enzymes are usually proteins. - **Enzyme Activity:** Enzymes are highly specific. The active site of an enzyme binds to its substrate (the reactant molecule). Induced fit: The active site changes shape slightly to embrace the substrate. - **Enzyme Inhibitors:** Molecules that inhibit enzyme activity by binding to the enzyme, either at the active site (competitive inhibition) or elsewhere (noncompetitive inhibition). Some inhibitors are reversible, others are irreversible. **Membrane Function:** - **Membrane Structure:** The plasma membrane is selectively permeable, allowing only certain substances to pass through. It consists primarily of a phospholipid bilayer with embedded proteins (fluid mosaic model). - **Passive Transport:** The movement of substances across a membrane without energy input. - **Diffusion:** The movement of molecules from an area of high concentration to an area of low concentration. - **Facilitated diffusion:** The diffusion of substances across a membrane with the help of transport proteins. - **Osmosis:** The diffusion of water across a selectively permeable membrane. - **Hypertonic:** Higher solute concentration. - **Hypotonic:** Lower solute concentration. - **Isotonic:** Equal solute concentration. - **Active Transport:** The movement of substances against their concentration gradient, requiring energy input (usually from ATP). - **Exocytosis:** The export of large molecules from the cell by fusion of vesicles with the plasma membrane. - **Endocytosis:** The import of large molecules into the cell by formation of vesicles from the plasma membrane. Types include phagocytosis (\"cellular eating\") and receptor-mediated endocytosis. **Nanotechnology & Evolution:** - **Harnessing Cellular Structures:** Nanotechnology draws inspiration from cellular structures and processes. *Example:* Researchers are attempting to harness the energy-producing capacity of sperm cells to power microscopic robots. - **Can Enzymes Be Engineered?** (Process of Science) Directed evolution is a laboratory technique used to create artificial enzymes with desired properties by mimicking natural selection. - **The Origin of Membranes:** (Evolution Connection) Experiments suggest that membranes could have formed spontaneously from phospholipids in the early Earth environment, a critical step in the origin of life. Chapter 6: Cellular Respiration: Obtaining Energy from Food ----------------------------------------------------------- This chapter explores how organisms harvest energy from food through the process of cellular respiration, emphasizing the crucial role of oxygen. It also touches upon the related processes of fermentation and the broader context of energy flow and chemical cycling in ecosystems. **Energy Flow and Chemical Cycling in the Biosphere:** Life depends on energy transformations. Almost all ecosystems rely on the sun as the ultimate energy source. Plants, algae, and certain bacteria, collectively known as *autotrophs* or *producers*, capture solar energy through *photosynthesis*. They convert light energy into chemical energy stored in sugars and other organic molecules, using inorganic carbon dioxide and water as starting materials and releasing oxygen as a byproduct. *Heterotrophs* or *consumers*, like animals and fungi, obtain their energy by consuming these organic molecules produced by autotrophs. Photosynthesis and cellular respiration are linked in a cycle. The products of photosynthesis (glucose and oxygen) are the reactants for cellular respiration, and the products of cellular respiration (carbon dioxide and water) are the reactants for photosynthesis. While chemicals are recycled, energy flows through ecosystems in a one-way path: entering as sunlight and exiting as heat. **Cellular Respiration: Aerobic Harvest of Food Energy:** Cellular respiration is the process by which cells harvest the chemical energy stored in organic fuel molecules, primarily glucose. It\'s an *aerobic* process, meaning it requires oxygen. This process occurs primarily in the mitochondria of eukaryotic cells. The overall equation for cellular respiration is: C₆H₁₂O₆ (glucose) + 6O₂ (oxygen) → 6CO₂ (carbon dioxide) + 6H₂O (water) + \~32 ATP (energy) The process can be broken down into three main stages: 1. **Glycolysis:** Occurs in the cytoplasm. Glucose is broken down into two molecules of pyruvic acid. A small amount of ATP is produced directly, and electrons are transferred to the electron carrier NAD⁺, forming NADH. 2. **Citric Acid Cycle (Krebs Cycle):** Occurs in the mitochondrial matrix. Pyruvic acid is further broken down, releasing carbon dioxide. A small amount of ATP is produced directly, and more electrons are captured by NAD⁺ and another electron carrier, FADH₂. 3. **Electron Transport Chain:** Occurs in the inner mitochondrial membrane. Electrons carried by NADH and FADH₂ are passed down a chain of protein molecules. This \"fall\" of electrons releases energy used to pump H⁺ ions across the membrane, creating a concentration gradient. The flow of H⁺ back across the membrane through ATP synthase drives the production of a large amount of ATP. Oxygen acts as the final electron acceptor at the end of the chain, forming water. **Fermentation: Anaerobic Harvest of Food Energy:** When oxygen is unavailable, some cells can still harvest energy through *fermentation*. This *anaerobic* process relies on glycolysis to produce ATP, but it yields much less ATP than cellular respiration. In human muscle cells, fermentation produces lactic acid as a byproduct. In other organisms, such as yeast, fermentation produces ethyl alcohol and carbon dioxide. **Key Concepts to Remember:** - Cellular respiration is the aerobic harvesting of energy from food. - The process occurs in three stages: glycolysis, the citric acid cycle, and the electron transport chain. - The electron transport chain produces the most ATP. - Oxygen is the final electron acceptor in cellular respiration. - Fermentation is an anaerobic process that yields much less ATP than cellular respiration. - Photosynthesis and cellular respiration are complementary processes that cycle chemicals and transfer energy in ecosystems. Chapter 7: Photosynthesis: Using Light to Make Food --------------------------------------------------- This chapter delves into the remarkable process of photosynthesis, where light energy is converted into chemical energy in the form of sugars. It explores the structures involved, the intricate steps of the process, and the implications for global energy. **The Basics of Photosynthesis:** Photosynthesis is the process used by plants, algae, and certain bacteria (called *photoautotrophs*) to convert light energy into chemical energy. It uses carbon dioxide and water as starting materials, producing sugars and releasing oxygen as a byproduct. The overall simplified equation is: 6CO₂ (carbon dioxide) + 6H₂O (water) \--Light Energy\--\> C₆H₁₂O₆ (glucose) + 6O₂ (oxygen) **Chloroplasts: Sites of Photosynthesis:** Photosynthesis occurs within specialized organelles called *chloroplasts*. These organelles contain a green pigment called *chlorophyll* which absorbs light energy. Chloroplasts are primarily found in the leaves of plants. Within the chloroplast, internal membranes called *thylakoids* are arranged in stacks called *grana*. The fluid-filled space surrounding the thylakoids is called the *stroma*. **An Overview of Photosynthesis:** Photosynthesis can be divided into two main stages: 1. **Light Reactions:** These reactions occur in the thylakoid membranes. Chlorophyll absorbs light energy, which is then used to split water molecules, releasing oxygen. The light energy is also converted into chemical energy in the form of ATP and NADPH, two energy-carrying molecules. 2. **Calvin Cycle:** These reactions take place in the stroma. The ATP and NADPH produced during the light reactions are used to convert carbon dioxide into glucose through a series of enzyme-catalyzed reactions. This process is also called *carbon fixation*. **The Light Reactions: Converting Solar Energy to Chemical Energy:** Sunlight is a form of electromagnetic radiation that travels in waves. *Visible light* is the portion of the electromagnetic spectrum that we can see. Chlorophyll absorbs light primarily in the blue-violet and red-orange regions of the spectrum, reflecting green light. Within the thylakoid membranes, chlorophyll and other pigment molecules are organized into *photosystems*. Each photosystem consists of a cluster of pigments that gather light energy and a *reaction center* where the light energy is converted into chemical energy. Two photosystems work together in a series of electron transfers to produce ATP and NADPH. **The Calvin Cycle: Making Sugar from Carbon Dioxide:** The Calvin cycle uses the ATP and NADPH generated during the light reactions to convert carbon dioxide into G3P (glyceraldehyde-3-phosphate), a three-carbon sugar. The plant then uses G3P to synthesize glucose and other organic molecules. The Calvin cycle is a cyclical process because the starting molecule is regenerated with each turn. **Key Concepts to Remember:** - Photosynthesis converts light energy into chemical energy stored in sugars. - It occurs in chloroplasts, specifically in the thylakoids (light reactions) and stroma (Calvin cycle). - Chlorophyll absorbs light energy to drive the process. - The light reactions produce ATP and NADPH, which are used in the Calvin cycle. - The Calvin cycle fixes carbon dioxide into sugars. - Photosynthesis is crucial for all ecosystems because it provides the base of the food chain and produces the oxygen we breathe. Chapter 8: Cellular Reproduction: Cells from Cells -------------------------------------------------- This chapter examines the fundamental process of cell division, detailing the mechanisms of both mitosis and meiosis and exploring the significance of these processes in growth, repair, and reproduction. **What Cell Reproduction Accomplishes:** Cell reproduction, also known as cell division, is essential for life. It serves three main functions: - **Cell replacement:** Damaged or worn-out cells are continuously replaced by new cells. - **Growth:** Multicellular organisms grow by increasing the number of cells through repeated cell divisions. - **Reproduction:** Many single-celled organisms reproduce asexually by dividing in half, and cell division is also crucial for the production of gametes (sperm and egg) for sexual reproduction. **The Cell Cycle and Mitosis:** The cell cycle is the ordered sequence of events that extends from the time a cell is first formed from a dividing parent cell until its own division into two cells. It consists of two main stages: - **Interphase:** This is the longest part of the cell cycle, where the cell grows, performs its normal functions, and duplicates its chromosomes in preparation for cell division. Interphase is divided into three subphases: G₁ (first gap), S (DNA synthesis), and G₂ (second gap). - **Mitotic (M) phase:** This phase encompasses the actual division of the nucleus (mitosis) and the division of the cytoplasm (cytokinesis). **Eukaryotic Chromosomes:** Eukaryotic chromosomes consist of *chromatin*, a complex of DNA and protein. Before cell division, the chromatin condenses into visible chromosomes. Each duplicated chromosome consists of two identical *sister chromatids* joined at the *centromere*. **Mitosis:** Mitosis is a continuous process with four main stages: 1. **Prophase:** The chromosomes condense, the nuclear envelope breaks down, and the *mitotic spindle* begins to form. 2. **Metaphase:** The chromosomes line up in the middle of the cell. 3. **Anaphase:** The sister chromatids separate and move to opposite poles of the cell. 4. **Telophase:** Two new nuclear envelopes form, the chromosomes uncoil, and the spindle disappears. **Cytokinesis:** Cytokinesis is the division of the cytoplasm. In animal cells, a *cleavage furrow* pinches the cell in two. In plant cells, a *cell plate* forms between the two new nuclei, eventually developing into a new cell wall. **Cancer Cells: Dividing Out of Control:** Cancer is a disease of the cell cycle. Cancer cells divide excessively and can invade other tissues, forming *malignant tumors*. This uncontrolled growth is caused by mutations in genes that regulate the cell cycle. **Meiosis, the Basis of Sexual Reproduction:** Meiosis is a type of cell division that produces *haploid* gametes (sperm and egg) in diploid organisms. Unlike mitosis, meiosis involves two rounds of cell division: meiosis I and meiosis II. **Homologous Chromosomes:** Diploid cells have pairs of *homologous chromosomes*, one from each parent. Homologous chromosomes carry genes for the same characteristics at the same loci. **The Process of Meiosis:** - **Meiosis I:** Homologous chromosomes pair up and exchange segments (*crossing over*) during prophase I. Homologous chromosomes then separate during anaphase I, resulting in two haploid cells. - **Meiosis II:** Sister chromatids separate during anaphase II, producing four haploid daughter cells. **The Origins of Genetic Variation:** Meiosis and fertilization contribute to genetic variation in offspring through three main mechanisms: - **Independent assortment of chromosomes:** Homologous chromosomes can line up in different ways during metaphase I, leading to different combinations of chromosomes in gametes. - **Crossing over:** The exchange of genetic material between homologous chromosomes during prophase I creates new combinations of alleles on chromosomes. - **Random fertilization:** Any sperm can fertilize any egg, resulting in a vast number of possible combinations of genes in the zygote. **When Meiosis Goes Away:** Errors during meiosis can lead to abnormal chromosome numbers. *Nondisjunction* is the failure of chromosomes or chromatids to separate properly during meiosis, resulting in gametes with extra or missing chromosomes. This can lead to disorders like Down syndrome (trisomy 21). Chapter 9: Patterns of Inheritance - A Summary ---------------------------------------------- This chapter explores the fundamental principles of heredity and how traits are passed from one generation to the next. It builds upon Mendel\'s foundational work and delves into more complex inheritance patterns. **Genetics and Heredity:** - **Heredity:** The transmission of traits from one generation to the next. - **Genetics:** The scientific study of heredity. - **Gregor Mendel:** A monk whose experiments with pea plants revealed basic patterns of inheritance. He used purebred varieties (genetically uniform) and controlled their reproduction by cross-fertilization (fertilizing one plant with pollen from another) and self-fertilization. - **Character:** A heritable feature that varies among individuals (e.g., flower color). - **Trait:** Each variant for a character (e.g., purple or white flowers). - **Hybrids:** Offspring of two different purebred varieties. - **P generation:** Parental plants. - **F₁ generation:** Hybrid offspring of the P generation. - **F₂ generation:** Offspring of the F₁ generation. **Mendel\'s Laws:** - **Law of Segregation:** Allele pairs separate (segregate) during gamete formation (meiosis), and fertilization restores the paired condition in the offspring. This explains the reappearance of recessive traits in the F₂ generation. - **Law of Independent Assortment:** The inheritance of one character has no effect on the inheritance of another. This means that alleles for different genes assort independently of each other during gamete formation. A dihybrid cross (tracking two characters) demonstrates this law, resulting in a 9:3:3:1 phenotypic ratio in the F₂ generation. **Genetic Terminology:** - **Alleles:** Different versions of a gene (like different flavors of ice cream). A gene for flower color might have alleles for purple and white. - **Homozygous:** An organism with two identical alleles for a gene (e.g., PP for purple flowers or pp for white flowers). Think \"same\". - **Heterozygous:** An organism with two different alleles for a gene (e.g., Pp for purple flowers). Think \"different\". - **Dominant Allele:** In heterozygotes, the allele that determines the organism\'s appearance (phenotype). It \"masks\" the recessive allele. - **Recessive Allele:** In heterozygotes, the allele that has no noticeable effect on the organism\'s appearance. It is only expressed when paired with another recessive allele. - **Phenotype:** An organism\'s observable traits (e.g., purple flowers, brown hair). What you *see*. - **Genotype:** An organism\'s genetic makeup, the alleles it carries (e.g., PP, Pp, pp). The underlying genetic code. - **Wild-type trait:** The trait most commonly found in nature. Note: This isn\'t necessarily the dominant trait. **Tools for Predicting Inheritance:** - **Punnett Square:** A diagram used to predict the genotypes and phenotypes of offspring from a genetic cross. - **Testcross:** A mating between an individual of unknown genotype and a homozygous recessive individual. It helps determine the unknown genotype. - **Probability:** Inheritance patterns follow the rules of probability. - **Rule of Multiplication:** The probability of a compound event is the product of the probabilities of each independent event. Example: The probability of inheriting two recessive alleles (one from each parent) is ½ \* ½ = ¼. - **Pedigree:** A family tree that traces the inheritance of a trait over several generations. It helps determine inheritance patterns and individual genotypes. **Variations on Mendel\'s Laws:** - **Incomplete Dominance:** The heterozygote has an intermediate phenotype between the two homozygotes (e.g., red flower x white flower = pink flower). - **Codominance:** Both alleles are expressed in the heterozygote. (e.g., human AB blood type -- both A and B carbohydrates are present on red blood cells). - **Multiple Alleles:** More than two alleles exist for a gene in the population (e.g., human ABO blood group -- alleles IA, IB, and i). - **Pleiotropy:** One gene affects multiple phenotypic characters (e.g., sickle-cell disease -- one mutation causes various symptoms). - **Polygenic Inheritance:** Multiple genes affect a single phenotypic character (e.g., human height, skin color). **Epigenetics and Environmental Influence:** - **Epigenetic Inheritance:** Inheritance of traits transmitted by mechanisms not directly involving the DNA sequence itself (e.g., chemical modifications to DNA or proteins). These modifications can be influenced by the environment. This explains how identical twins with the same genotype can have different phenotypes. **The Chromosomal Basis of Inheritance:** - **Chromosome Theory of Inheritance:** Genes are located on chromosomes, and their behavior during meiosis and fertilization accounts for inheritance patterns. - **Linked Genes:** Genes located near each other on the same chromosome tend to be inherited together. They don\'t always follow the law of independent assortment. - **Sex-Linked Genes:** Genes located on the sex chromosomes (usually the X chromosome). Their inheritance patterns differ between males and females because males have only one X chromosome (XY) while females have two (XX). (e.g., red-green colorblindness, hemophilia). **Human Genetic Disorders:** Many genetic disorders are inherited as recessive traits (e.g., cystic fibrosis, albinism). Others, like Huntington\'s disease and achondroplasia, are caused by dominant alleles. **Genetic Testing:** Tests can detect disease-causing alleles in an individual\'s genome, often during pregnancy (e.g., amniocentesis, chorionic villus sampling). Chapter 10: The Structure and Function of DNA - A Summary --------------------------------------------------------- This chapter explores the intricate world of DNA, its structure, how it replicates, the flow of genetic information from DNA to protein, and the impact of mutations and viruses. **DNA and RNA Structure:** - Both DNA (deoxyribonucleic acid) and RNA (ribonucleic acid) are nucleic acids, polymers composed of nucleotide monomers. - Each nucleotide consists of a nitrogenous base, a five-carbon sugar, and a phosphate group. - Nucleotides link together via covalent bonds between the sugar of one and the phosphate of the next, forming a sugar-phosphate backbone. Nitrogenous bases extend from this backbone. - DNA uses the sugar deoxyribose, while RNA uses ribose. - DNA contains the bases adenine (A), thymine (T), guanine (G), and cytosine (C). RNA substitutes uracil (U) for thymine. - DNA is typically double-stranded, while RNA is single-stranded. **DNA\'s Double Helix:** - James Watson and Francis Crick, with crucial data from Rosalind Franklin, elucidated DNA\'s three-dimensional structure: a double helix. - Two polynucleotide strands wrap around each other, held together by hydrogen bonds between complementary bases. - A always pairs with T (two hydrogen bonds), and G always pairs with C (three hydrogen bonds). - The specific base pairing is crucial for DNA replication and the accurate transmission of genetic information. **DNA Replication:** - DNA replicates in a semi-conservative manner, meaning each new DNA molecule consists of one parental strand and one newly synthesized strand. - The process begins at origins of replication, where the double helix unwinds. - DNA polymerases add nucleotides to the growing daughter strands, following base-pairing rules. - DNA replication is incredibly accurate due to the specificity of base pairing and the proofreading capabilities of DNA polymerases. **Information Flow: From DNA to RNA to Protein:** The central dogma of molecular biology describes the flow of genetic information: DNA → RNA → Protein. This involves two key steps: - **Transcription:** The synthesis of RNA using a DNA template. RNA polymerase binds to a gene\'s promoter region and adds RNA nucleotides complementary to the DNA template strand. In eukaryotes, the resulting RNA transcript is processed by adding a cap and tail, and removing non-coding regions called introns via RNA splicing. - **Translation:** The synthesis of a polypeptide using an mRNA template. Ribosomes in the cytoplasm coordinate the interaction between mRNA codons and tRNA anticodons. Each tRNA carries a specific amino acid, which is added to the growing polypeptide chain based on the mRNA codon sequence. The process continues until a stop codon is reached. **The Genetic Code:** - The genetic code is a set of rules that dictates the correspondence between mRNA codons and amino acids. - It\'s nearly universal, shared by most organisms, highlighting the evolutionary connectedness of life. - 61 codons specify amino acids, while 3 are stop codons. AUG codes for methionine and serves as the start codon. **Mutations:** - Mutations are changes in the DNA base sequence, arising from errors during DNA replication or from exposure to mutagens (e.g., radiation, certain chemicals). - **Types of mutations:** - **Substitution:** One base pair is replaced by another. Can be silent (no amino acid change), missense (different amino acid), or nonsense (introduces a stop codon). - **Insertion/Deletion:** One or more nucleotides are added or removed, causing frameshift mutations that alter the reading frame downstream. **Viruses:** - Viruses are infectious particles consisting of genetic material (DNA or RNA) enclosed in a protein coat (capsid), and sometimes a membranous envelope. - They are not considered living organisms because they cannot reproduce on their own. - Viruses infect host cells and hijack the cellular machinery to replicate their genetic material and produce new viral particles. - **Bacteriophages:** Viruses that infect bacteria. Can undergo a lytic cycle (destroying the host cell) or a lysogenic cycle (integrating their DNA into the host chromosome). - **Plant Viruses:** Often have RNA genomes and require damage to the plant\'s outer layers for infection. - **Animal Viruses:** Can have DNA or RNA genomes. Some have envelopes derived from the host cell membrane. Retroviruses, like HIV, use reverse transcriptase to convert RNA to DNA. **Viroids and Prions:** - **Viroids:** Small, circular RNA molecules that infect plants. - **Prions:** Infectious proteins that cause brain diseases in animals, including mad cow disease in cattle and Creutzfeldt-Jakob disease in humans. They propagate by converting normal proteins into misfolded prion versions. **Chapter 11: How Genes Are Controlled** This chapter explores gene regulation, the intricate process that dictates which genes are active at any given time, influencing cell specialization and organismal development. **Why Gene Regulation Matters:** Gene regulation is crucial for cell specialization and organismal complexity. Every cell in a sexually reproducing organism originates from the zygote and inherits identical DNA. Yet, cells differentiate into diverse types with distinct structures and functions due to gene regulation. This process is analogous to restaurants using the same cookbook but selecting different recipes to create unique menus. **Gene Regulation in Bacteria:** Bacteria utilize operons for gene regulation. An operon is a cluster of related genes along with their control sequences. The *lac* operon in *E. coli* serves as an example: - The *promoter* is the site where RNA polymerase binds to initiate transcription. - The *operator* acts as a switch, controlling RNA polymerase access to the promoter. - A *repressor* protein can bind to the operator, preventing transcription. - The presence of lactose inactivates the repressor, allowing transcription of genes for lactose metabolism. **Gene Regulation in Eukaryotic Cells:** Eukaryotes have more complex gene regulation mechanisms than bacteria. The \"pipeline\" from gene to protein offers multiple control points: 1. **DNA Packing:** Coiling and folding of DNA can influence gene expression. X chromosome inactivation in female mammals is a prime example. 2. **Transcription Initiation:** This is the most important control point. Transcription factors (proteins) interact with enhancers and the promoter to influence RNA polymerase binding. Silencers are DNA sequences where repressor proteins bind, inhibiting transcription. 3. **RNA Processing:** Alternative RNA splicing creates different mRNAs from the same gene. 4. **mRNA Breakdown:** mRNA lifespan varies, affecting protein production. 5. **microRNAs (miRNAs):** Small RNAs can bind to mRNAs, either degrading them or blocking translation. 6. **Translation Initiation:** Regulatory proteins can control translation start. 7. **Protein Activation and Breakdown:** Proteins may require modification for activation, and their breakdown rates can be regulated. **Cell Signaling:** In multicellular organisms, gene regulation can be influenced by signals from other cells. Signal transduction pathways convey external signals into the cell, triggering cellular responses, including changes in gene expression. **Homeotic Genes:** These master control genes regulate groups of other genes during embryonic development, determining body part placement. Mutations in homeotic genes can lead to dramatic developmental abnormalities. **DNA Microarrays:** This technology enables visualization of gene expression by comparing mRNA from different cells or tissues. Fluorescently labeled cDNA from a sample is hybridized to a microarray containing DNA fragments from thousands of genes. Glowing spots indicate active genes. **Cloning Plants and Animals:** - **Genetic Potential of Cells:** Differentiated cells retain all genes, even if not expressed. Plant cloning demonstrates this by growing a whole plant from a single cell. - **Reproductive Cloning:** Nuclear transplantation involves replacing the nucleus of an egg or zygote with a nucleus from an adult body cell. The resulting embryo can develop into a clone of the donor. Dolly the sheep was the first mammal cloned from an adult cell. - **Therapeutic Cloning and Stem Cells:** This technique aims to produce embryonic stem cells (ES cells), which have the potential to differentiate into various cell types for medical applications. Umbilical cord blood and adult stem cells are also potential sources. **The Genetic Basis of Cancer:** Cancer involves uncontrolled cell division. Several mutations are typically required to produce a fully cancerous cell. - **Oncogenes:** These cancer-causing genes arise from mutations in proto-oncogenes, which normally code for proteins involved in cell growth and division. - **Tumor-suppressor genes:** These genes normally inhibit cell division. Mutations that deactivate tumor-suppressor proteins can lead to cancer. **Cancer Risk and Prevention:** Most cancers are caused by carcinogens, such as UV radiation and tobacco. Lifestyle choices, including diet, can influence cancer risk. **Chapter 12: DNA Technology** This chapter delves into the revolutionary field of DNA technology, exploring its diverse applications and the ethical considerations it raises. **DNA Profiling:** DNA profiling analyzes DNA samples to determine if they come from the same individual, revolutionizing forensic science. The process involves: 1. **DNA Isolation:** Obtaining DNA samples from crime scenes, suspects, victims, etc. 2. **DNA Amplification:** Using PCR to create many copies of specific DNA sequences. 3. **DNA Comparison:** Comparing amplified fragments to determine matches. **Key DNA Technologies:** - **Recombinant DNA:** Combining DNA from two sources, often different species, to create a single DNA molecule. - **Gene Cloning:** Producing multiple identical copies of a gene-carrying piece of DNA, typically using bacterial plasmids as vectors. - **Restriction Enzymes:** Bacterial enzymes that cut DNA at specific sequences (restriction sites), creating restriction fragments with \"sticky ends\" for joining DNA from different sources. - **DNA Ligase:** Enzyme that joins DNA fragments together. - **Gel Electrophoresis:** Separates DNA fragments by size and charge. DNA, negatively charged due to phosphate groups, moves towards the positive electrode. Shorter fragments move faster through the gel. - **Polymerase Chain Reaction (PCR):** Amplifies specific DNA segments rapidly. It uses heat-stable DNA polymerase, primers (short DNA sequences that flank the target region), and cycles of heating and cooling to replicate the target DNA exponentially. - **Short Tandem Repeat (STR) Analysis:** Compares the lengths of STR sequences (short, repeated DNA sequences) at specific sites in the genome, allowing for individual identification. **Applications of DNA Technology:** - **Pharmaceutical Products:** Genetically modified organisms (GMOs), especially bacteria, are used to produce large quantities of human proteins like insulin (Humulin), human growth hormone (HGH), and tissue plasminogen activator (tPA). - **Genetically Modified Organisms (GMOs) in Agriculture:** Genes are introduced into crops to enhance insect resistance (e.g., Bt corn), herbicide resistance, or nutritional value (e.g., golden rice). Similar efforts are underway with farm animals. - **Human Gene Therapy:** Introducing genes into an afflicted person to treat disease. One approach uses a virus carrying a normal human gene to infect a patient\'s bone marrow cells, aiming to correct genetic disorders. **Bioinformatics:** Bioinformatics uses computational tools to store and analyze biological data, particularly DNA sequences. - **DNA Sequencing:** Determining the complete nucleotide sequence of a DNA molecule. Next-generation sequencing techniques have greatly accelerated this process. - **Genomics:** Study of whole sets of genes (genomes). The Human Genome Project sequenced the entire human genome. - **Genome-Mapping Techniques:** The whole-genome shotgun method is commonly used, involving fragmenting the genome, cloning and sequencing fragments, and then reassembling the sequences using computer software. - **Proteomics:** Study of whole sets of proteins (proteomes) encoded by genomes. - **Systems Biology:** Aims to model the behavior of whole biological systems based on the study of interactions among system parts, integrating data from genomics, proteomics, and other fields. **Safety and Ethical Issues:** - **GMO Concerns:** Potential environmental and health risks of GMOs are debated, including gene flow to wild relatives, reduced genetic diversity, and introduction of allergens. - **Ethical Concerns of Human DNA Technologies:** Privacy issues related to DNA profiling and the ethical implications of human gene therapy, including germline gene editing, are actively discussed. **Chapter 13: How Populations Evolve** This chapter explores the mechanisms of evolution, focusing on how populations change over time and how new species arise. **Evolution Today:** Evolution is an ongoing process, observable in both natural and human-influenced environments. Examples include pesticide resistance in insects, adaptation to human-altered landscapes, and the evolution of drug-resistant microbes. **The Diversity of Life:** - **Taxonomy:** The branch of biology concerned with identifying, naming, and classifying species. - **Binomial Nomenclature:** The two-part Latinized naming system (Genus species) developed by Carolus Linnaeus. - **Hierarchical Classification:** Organisms are grouped into increasingly broad categories: Domain, Kingdom, Phylum, Class, Order, Family, Genus, Species. **Explaining the Diversity of Life:** - **The Idea of Fixed Species:** The historical view that species are unchanging, contrasting with the evolutionary perspective. - **Lamarck and Evolutionary Adaptations:** Lamarck proposed that organisms evolve by inheriting acquired characteristics, a concept later disproven. However, his idea that organisms change over time paved the way for Darwin. **Charles Darwin and *The Origin of Species*:** - **Darwin\'s Journey:** Observations from his voyage on the HMS Beagle, especially in the Galápagos Islands, heavily influenced his thinking about evolution. He observed that species vary globally, locally, and over time. - **Darwin\'s Theory:** Darwin proposed *descent with modification* (evolution) by *natural selection*, where individuals with advantageous traits are more likely to survive and reproduce. **Evidence of Evolution:** - **Fossils:** The fossil record documents the history of life, showing changes in organisms over time and the existence of extinct species. Fossils of transitional forms link different groups of organisms, supporting evolutionary relationships. - **Homologies:** Similarities resulting from common ancestry. - *Homologous Structures:* Anatomical similarities in different organisms due to common ancestry, even if the structures have different functions. - *Molecular Homologies:* Similarities in DNA and RNA sequences or the genetic code, indicating common ancestry. The more similar the sequences, the more recent the common ancestor. - **Evolutionary Trees:** Diagrams representing evolutionary relationships among groups of organisms. Branch points represent common ancestors. **Natural Selection as the Mechanism for Evolution:** - **Artificial Selection:** Selective breeding of domesticated plants and animals to promote the occurrence of desirable traits, demonstrating the power of selection to cause change. - **Key Points about Natural Selection:** - Acts on individuals but populations evolve. - Affects only heritable traits. - Is an editing process, not a creative mechanism. - Is not goal-directed. **The Evolution of Populations:** - **Sources of Genetic Variation:** Mutation (changes in DNA sequences) is the ultimate source of new alleles. Sexual reproduction shuffles existing alleles to create new combinations. - **Populations as Units of Evolution:** A *population* is a group of individuals of the same species that live in the same area and interbreed. The *gene pool* consists of all copies of every type of allele at every locus in all members of the population. - **Analyzing Gene Pools:** - *Allele Frequency:* The proportion of a particular allele in the population. - *Hardy-Weinberg Equation:* Describes the genetic makeup of a population that is *not* evolving (at genetic equilibrium). The equation is: *p*² + 2*pq* + *q*² = 1, where *p* is the frequency of one allele, *q* is the frequency of the other allele, *p*² and *q*² are the frequencies of homozygotes, and 2*pq* is the frequency of heterozygotes. - **Microevolution:** Generation-to-generation change in allele frequencies in a population. **Mechanisms that Alter Allele Frequencies:** - **Genetic Drift:** Random change in allele frequencies, most pronounced in small populations. - *Bottleneck Effect:* Drastic reduction in population size, reducing genetic diversity. - *Founder Effect:* A few individuals colonize a new habitat, with a gene pool different from the original population. - **Gene Flow:** Genetic exchange with another population, tending to reduce differences between populations. - **Natural Selection (Three Outcomes):** - *Directional Selection:* Favors one extreme phenotype. - *Disruptive Selection:* Favors both extreme phenotypes. - *Stabilizing Selection:* Favors intermediate phenotypes. - **Sexual Selection:** Natural selection in which individuals with certain traits are more likely to obtain mates. This can lead to *sexual dimorphism*, differences in appearance between males and females. Chapter 14: How Biological Diversity Evolves -------------------------------------------- This chapter explores the fascinating question of how the incredible diversity of life on Earth arose. It delves into the mechanisms of speciation, the birth of new species, and how evolutionary relationships are traced and classified. **Why Evolution Matters:** Evolution is not just a historical event, it\'s an ongoing process that shapes the biological world around us, affecting everything from the food we eat to the medicines we use. **What is a Species?** The most commonly used definition relies on the **biological species concept**, which defines a species as a group of populations whose members can potentially interbreed in nature and produce fertile offspring. However, this definition has limitations. It cannot be applied to organisms that reproduce asexually (like many prokaryotes) or to fossils. Other species concepts exist, including those based on measurable physical traits, shared ancestry, and molecular data (like a species-specific \"barcode\"). **Reproductive Barriers Between Species:** Even closely related species are prevented from interbreeding by **reproductive barriers**, which can be categorized as either *prezygotic* or *postzygotic*. - **Prezygotic Barriers:** These barriers prevent mating or fertilization from occurring. Examples include: - **Temporal isolation:** Species breed during different times of day or year. - **Habitat isolation:** Species live in different habitats within the same area. - **Behavioral isolation:** Courtship rituals and other behaviors unique to a species are effective barriers. - **Mechanical isolation:** Morphological differences prevent successful mating. - **Gametic isolation:** Sperm of one species may not be able to fertilize eggs of another species. - **Postzygotic Barriers:** These barriers operate after hybrid zygotes have formed. Examples include: - **Reduced hybrid viability:** Interaction of parental genes may result in a hybrid offspring that cannot survive. - **Reduced hybrid fertility:** Even if a hybrid offspring survives, it may be infertile. - **Hybrid breakdown:** First-generation hybrids may be fertile, but subsequent generations lose fertility. **Mechanisms of Speciation:** Speciation occurs when a population becomes reproductively isolated from other populations of the parent species, allowing it to follow its own unique evolutionary path. This can happen in two main ways: - **Allopatric Speciation:** Gene flow is interrupted when a population is divided into geographically isolated subpopulations. For example, a new mountain range may split a population that inhabits lowlands. Over time, the separate populations may diverge genetically to the point where they can no longer interbreed, even if they come into contact again. The likelihood of allopatric speciation increases when the isolated population is small, as genetic drift and natural selection can have a greater impact on allele frequencies. - **Sympatric Speciation:** Speciation takes place in geographically overlapping populations. This can occur through several mechanisms, the most common of which is **polyploidy**, a condition where an organism has extra sets of chromosomes. Polyploidy is particularly common in plants. It can occur within a single species if an error in cell division results in offspring with extra sets of chromosomes or through hybridization between two different species. In both cases, the polyploid offspring are reproductively isolated from their parent species. Other mechanisms of sympatric speciation include habitat differentiation and sexual selection. - **Observing Speciation in Progress:** While speciation is often a slow process, taking thousands or even millions of years, biologists *can* observe speciation occurring in present times. This is because the environment is constantly changing, and populations are continually adapting through natural selection. Studying diverging populations provides valuable insights into the speciation process. Researchers have documented instances of populations diverging due to factors like different food sources or habitat preferences, as well as through sexual selection. Though these populations may still be considered subspecies, these studies provide snapshots of speciation in action. **Earth History and Macroevolution:** **Macroevolution** encompasses the grand sweep of evolutionary change above the species level. This includes the origin of new groups of organisms, the evolution of novel traits, and the impact of mass extinctions. The study of macroevolution relies heavily on the **fossil record**. - **The Fossil Record:** Fossils are preserved remnants or impressions of organisms that lived in the past. The sequence of fossils in rock strata, known as the **fossil record**, reveals the order in which organisms evolved. Deeper strata contain older fossils. **Radiometric dating**, based on the decay of radioactive isotopes, is used to determine the ages of fossils and rocks, providing absolute dates for events in evolutionary history. The **geologic time scale** divides Earth\'s history into a series of eons, eras, periods, and epochs, based on fossil evidence and radiometric dating. Mass extinctions mark the boundaries between eras. - **Plate Tectonics and Biogeography:** The theory of **plate tectonics** explains the movement of Earth\'s continents over geologic time. **Continental drift** has profoundly impacted the evolution of life by reshaping the physical features of the planet, altering habitats, and influencing the geographic distribution of organisms. The formation of **Pangaea**, a supercontinent that existed about 250 million years ago, and its subsequent breakup have played major roles in the evolution of biodiversity. **Biogeography**, the study of the past and present distribution of organisms, provides evidence for the impact of continental drift on speciation patterns. - **Mass Extinctions and Explosive Diversifications:** The fossil record reveals five major **mass extinctions**, periods when a large percentage of Earth\'s species went extinct. These events have been followed by periods of **explosive diversification**, where surviving lineages rapidly adapt and radiate into new ecological roles. The extinction of the dinosaurs, for example, paved the way for the diversification of mammals. **Mechanisms of Macroevolution:** While speciation occurs through microevolutionary processes like natural selection and genetic drift, macroevolutionary changes often involve larger-scale events and modifications in development: - **Large Effects From Small Genetic Changes:** Relatively minor changes in genes that control development can have significant impacts on an organism\'s morphology. **Homeotic genes**, which regulate the timing and spatial pattern of development, play a crucial role in shaping body form. Changes in the timing of developmental events can also lead to dramatic changes, such as **paedomorphosis**, the retention of juvenile features in the adult. - **The Evolution of Biological Novelty:** **Exaptations** are structures that originally evolved for one function but later became co-opted for a different function. For example, feathers likely first evolved for insulation or display in dinosaurs and were later co-opted for flight in birds. Complex structures typically evolve gradually, through a series of incremental modifications of simpler structures. The evolution of the eye is a classic example of this process. **Classifying the Diversity of Life:** - **Classification and Phylogeny:** **Systematics** is the discipline of biology that focuses on classifying organisms and determining their evolutionary relationships. Biologists use **phylogenetic trees** to represent evolutionary history. **Cladistics** is a method of classifying organisms based on shared, derived characters, organizing species into **clades**, which consist of an ancestral species and all its descendants. Homologous characters are used to infer evolutionary relationships, while analogous characters, which result from convergent evolution, are not. - **Classification: A Work in Progress:** Biological classification is continually being revised based on new evidence from molecular data and other sources. The **three-domain system** (Bacteria, Archaea, and Eukarya) is the most widely accepted classification scheme today. Within the domain Eukarya, there is still ongoing debate about the precise number and boundaries of kingdoms, particularly within the protists. The Evolution of Microbial Life ------------------------------- This chapter explores the vast and often overlooked world of microorganisms, focusing on prokaryotes (bacteria and archaea) and protists. It covers their evolution, diversity, ecological roles, and their impact on human life. **Why Microorganisms Matter:** Microorganisms are not just germs; they are fundamental to life on Earth, playing crucial roles in ecosystems and even within our own bodies. **Human Microbiota:** The human body is home to trillions of microorganisms, collectively known as the **human microbiota**. These microbes, mostly bacteria, archaea, and protists, outnumber our own cells. They inhabit various parts of our body, including the skin, mouth, nasal passages, and digestive tract. The microbiota plays essential roles in human health, including nutrient absorption, vitamin synthesis, and protection against disease. However, disruptions to the microbiota can have negative consequences, potentially increasing susceptibility to infections and other health problems. **Major Episodes in the History of Life:** Life on Earth began with prokaryotes, which dominated for billions of years before eukaryotes evolved. Key events in the history of life include: 1. **Origin of Earth:** \~4.6 billion years ago. 2. **Oldest known rocks:** \~3.85 billion years ago. 3. **First prokaryotes:** \~3.5 billion years ago. 4. **Increasing atmospheric O₂ (due to photosynthesis):** \~2.7 billion years ago. 5. **First eukaryotes:** \~1.8 billion years ago. 6. **Oldest multicellular fossils:** \~1.2 billion years ago. 7. **Large, complex multicellular organisms:** \~600 million years ago. 8. **Colonization of land by plants, fungi, and animals:** \~500 million years ago. **The Origin of Life:** The leading hypothesis for the origin of life proposes a four-stage process: 1. **Abiotic Synthesis of Organic Monomers:** Small organic molecules, the building blocks of life, formed from inorganic precursors. The Miller-Urey experiment demonstrated that this was possible under simulated early-Earth conditions. Other potential sources of organic monomers include hydrothermal vents and meteorites. 2. **Abiotic Synthesis of Polymers:** Organic monomers joined to form larger polymers, such as proteins and nucleic acids. This could have occurred on hot surfaces like clay or rock, where water evaporated and concentrated the monomers, facilitating their polymerization. 3. **Formation of Pre-Cells:** Polymers became enclosed in membranes, forming **pre-cells**, which maintained an internal environment distinct from their surroundings. Pre-cells exhibited some properties of life, such as the ability to grow and divide. 4. **Origin of Self-Replicating Molecules:** The emergence of self-replicating molecules, likely RNA, made inheritance possible. **Ribozymes**, RNA molecules that can catalyze reactions, may have played a crucial role in early self-replication. Natural selection then acted on pre-cells containing self-replicating RNA, favoring those that were more efficient at replicating and growing, eventually leading to the first true cells. **Prokaryotes:** - **They\'re Everywhere:** Prokaryotes inhabit virtually every environment on Earth, including extreme habitats that are too hot, cold, salty, or acidic for eukaryotes. - **Structure/Function:** Prokaryotic cells are simpler than eukaryotic cells, lacking a nucleus and other membrane-bound organelles. Most prokaryotes have cell walls, and many have flagella for movement. Common prokaryotic shapes include spherical (cocci), rod-shaped (bacilli), and spiral (spirochetes). Some prokaryotes form colonies or biofilms. - **Prokaryotic Reproduction:** Prokaryotes reproduce rapidly by **binary fission**, a type of asexual reproduction. High reproductive rates and frequent mutations generate considerable genetic variation, allowing for rapid adaptation to changing environments. Some prokaryotes form resistant **endospores** to survive harsh conditions. - **Prokaryotic Nutrition:** Prokaryotes exhibit diverse metabolic pathways. Some are **autotrophs**, like plants, obtaining energy from sunlight through photosynthesis. Others are **heterotrophs**, obtaining energy and carbon from organic molecules. Some prokaryotes, called **chemotrophs**, obtain energy from inorganic substances. - **The Ecological Impact of Prokaryotes:** Prokaryotes play essential roles in **chemical recycling**, decomposing organic matter and returning nutrients to the environment. They are also crucial for **nitrogen fixation**, converting atmospheric nitrogen into forms usable by plants. **Bioremediation** uses prokaryotes to remove pollutants from the environment. - **The Two Main Branches of Prokaryotic Evolution:** Prokaryotes are divided into two domains: **Bacteria** and **Archaea**. Archaea often inhabit extreme environments and have unique structural and biochemical characteristics. Some bacteria are **pathogens**, causing disease by producing toxins. **Protists:** - **Protozoans:** Unicellular eukaryotes that ingest their food, including amoebas, flagellates, apicomplexans, and ciliates. - **Slime Molds:** Multicellular protists that resemble fungi in appearance and lifestyle, but are not closely related. - **Unicellular and Colonial Algae:** Photosynthetic protists that support food chains in aquatic ecosystems, including dinoflagellates, diatoms, and green algae. - **Seaweeds:** Large, multicellular marine algae that are not closely related to plants but share some similarities due to convergent evolution. Chapter 16: The Evolution of Plants and Fungi - A Summary --------------------------------------------------------- This chapter explores the evolutionary journey of plants and fungi, highlighting their adaptations to terrestrial life and their profound ecological impact. **Colonizing Land:** The transition from aquatic to terrestrial life presented challenges for plants, demanding new adaptations. Unlike algae, which absorb resources directly from the water, land plants require specialized structures to obtain resources from two distinct environments: air and soil. - **Roots:** Anchor plants, absorb water and minerals from the soil, and often form symbiotic associations with fungi called mycorrhizae. Mycorrhizae greatly enhance the root\'s absorptive surface area, a key adaptation for terrestrial life. - **Shoots:** Consist of photosynthetic leaves supported by stems. Leaves are coated with a waxy cuticle to reduce water loss, and stomata regulate gas exchange. Stems provide structural support, often reinforced by lignin, a key component of wood. - **Vascular Tissue:** A network of tube-shaped cells transports water and minerals from roots to leaves and distributes sugars throughout the plant. Lignin in vascular tissue also provides structural support, allowing plants to grow tall. - **Reproductive Adaptations:** Plants evolved mechanisms to keep their gametes and developing offspring from drying out. Gametes are produced in protective structures, and the fertilized egg develops into an embryo while still contained within the female parent. Some plants evolved adaptations for airborne sperm (pollen) and improved dispersal of offspring via seeds. **The Origin of Plants from Green Algae:** Plants evolved from charophytes, a group of green algae that inhabited shallow waters prone to occasional drying. Natural selection favored algae that could survive periods of drought, leading to the evolution of terrestrial adaptations in plants. **Plant Diversity:** Plant evolution is marked by four major periods: 1. **Origin of Terrestrial Adaptations (Bryophytes):** Bryophytes, such as mosses, were the first land plants. They lack true roots and leaves and have weak upright support because they lack lignin. They require water for reproduction because their sperm swim to the eggs. In bryophytes, the gametophyte generation is dominant. 2. **Origin of Vascular Tissue (Ferns):** Ferns and other seedless vascular plants evolved vascular tissue hardened by lignin, allowing them to grow taller and colonize a wider range of habitats than bryophytes. They still require water for reproduction. The sporophyte generation is dominant in ferns. 3. **Origin of Seeds (Gymnosperms):** Gymnosperms, such as conifers, evolved seeds, which further protected plant embryos from drying and other hazards. A seed consists of an embryo packaged with a food supply within a protective covering. Gymnosperms also evolved pollen, allowing for wind-mediated transfer of sperm to egg, eliminating the need for water. The sporophyte generation is dominant in gymnosperms. 4. **Origin of Flowers (Angiosperms):** Angiosperms, or flowering plants, evolved flowers, complex reproductive structures that bear seeds within protective chambers called ovaries. The flower\'s showiness attracts pollinators, facilitating efficient transfer of pollen. Refinements in vascular tissue further enhanced water transport. Angiosperms produce fruits, which develop from the ovary and aid in seed dispersal. The sporophyte generation is dominant in angiosperms. **Fungi:** Fungi are eukaryotes more closely related to animals than to plants. They are heterotrophs that obtain nutrients by absorption after externally digesting food. - **Fungal Structure:** Most fungi are constructed of threadlike filaments called hyphae, which form an interwoven network called a mycelium. The cell walls of fungi are mainly composed of chitin. The mycelium is the feeding structure of the fungus. - **Fungal Reproduction:** Fungi typically reproduce by releasing haploid spores that are produced either sexually or asexually. Spores germinate to produce mycelia. **The Ecological Impact of Fungi:** - **Decomposers:** Fungi and bacteria are the principal decomposers in ecosystems, breaking down organic matter and recycling vital chemical elements. - **Parasites:** Some fungi absorb nutrients from the cells or body fluids of living hosts, causing diseases in plants and animals. - **Commercial Uses:** Fungi are used in food production (mushrooms, cheese, bread, beer, wine) and in the manufacture of antibiotics. **Plant-Fungus Interactions:** Symbiotic relationships between fungi and plants are crucial for the success of both kingdoms. Mycorrhizae are symbiotic associations between fungi and plant roots that enhance nutrient and water uptake by plants. Lichens are symbiotic associations between fungi and algae or photosynthetic bacteria. The fungus provides habitat, and the algae or bacteria provide food. **Plant Diversity as a Nonrenewable Resource:** The ever-increasing human population is causing the extinction of plant species at an alarming rate, especially in forest ecosystems. Deforestation, driven by demands for timber, land for housing, and agriculture, is a major threat to plant diversity, particularly in the tropics. Chapter 17: The Evolution of Animals - A Summary ------------------------------------------------ This chapter explores the vast diversity of the animal kingdom, tracing its evolutionary history and highlighting key adaptations that have led to the success of various animal groups. Special attention is given to the vertebrates and the fascinating story of human evolution. **The Origins of Animal Diversity** - **What is an Animal?** Animals are defined as multicellular, heterotrophic eukaryotes that ingest food. Unlike fungi, which absorb nutrients after external digestion, animals digest food internally. Most animals reproduce sexually, with a life cycle including haploid gametes (sperm and egg), a diploid zygote, a blastula stage (hollow ball of cells), a gastrula stage (embryo with tissue layers), and potentially a larval stage before reaching adulthood. - **Early Animals and the Cambrian Explosion:** Animals likely evolved from a colonial, flagellated protist ancestor. The earliest animal fossils, dating back 550-575 million years, are impressions of soft-bodied organisms. The Cambrian explosion (535-525 million years ago) marks a rapid diversification of animal body plans, with many new phyla appearing. This burst of evolution may be attributed to factors like evolving predator-prey relationships and increased atmospheric oxygen. Also, the genetic framework for complex bodies was likely already established, with subsequent evolution primarily involving variations on existing themes. - **Animal Phylogeny:** Animal phylogeny, the evolutionary history of animals, is often categorized by body plan. Key distinctions include the presence or absence of true tissues (groups of similar cells performing a function) and body symmetry (radial or bilateral). Sponges lack true tissues. Bilateral symmetry, with a distinct head end, is associated with mobility and a concentration of sense organs and nervous tissue at the head. Body cavities, fluid-filled spaces separating the digestive tract from the outer body wall, also contributed to animal complexity by allowing internal organs to grow and move independently. **Major Invertebrate Phyla** Invertebrates, animals without backbones, comprise 95% of all animal species. Here are some key invertebrate phyla: - **Sponges (Porifera):** Stationary, porous bodies, no true tissues. Specialized cells filter food particles from water drawn through pores. - **Cnidarians (Cnidaria):** Radial symmetry, tentacles with stinging cells (cnidocytes), gastrovascular cavity with one opening. Include jellyfish, corals, and sea anemones. Exist as polyps (stationary) or medusae (floating). - **Molluscs (Mollusca):** Soft-bodied, often with a shell. Body plan includes a muscular foot, visceral mass (containing internal organs), and mantle (tissue that secretes the shell). Include snails, clams, and octopuses. - **Flatworms (Platyhelminthes):** Simplest bilateral animals. May be free-living (planarians) or parasitic (tapeworms, flukes). - **Annelids (Annelida):** Segmented worms, complete digestive tract, body cavity. Include earthworms and leeches. - **Roundworms (Nematoda):** Cylindrical, unsegmented bodies, tapered at both ends. Important decomposers and some are parasitic. - **Arthropods (Arthropoda):** Segmented bodies, jointed appendages, exoskeleton. Includes arachnids (spiders, scorpions), crustaceans (crabs, lobsters), millipedes, centipedes, and insects. Insects are the most diverse animal group, with success attributed to flight and metamorphosis. - **Echinoderms (Echinodermata):** Spiny skin, water vascular system, endoskeleton, radial symmetry as adults (bilateral larvae). Include sea stars and sea urchins. Share an evolutionary branch with chordates. **Vertebrate Evolution and Diversity** Vertebrates are chordates with a backbone. Chordates share four key embryonic features: a dorsal, hollow nerve cord; a notochord; pharyngeal slits; and a post-anal tail. - **Fishes:** The earliest vertebrates were jawless fishes (e.g., hagfishes and lampreys). Jawed fishes evolved later, with cartilaginous fishes (sharks, rays) having a flexible cartilage skeleton and bony fishes having a calcium-reinforced skeleton. Bony fishes include ray-finned fishes and lobe-finned fishes. Lobe-finned fishes have muscular fins that gave rise to amphibian limbs. - **Amphibians:** Tetrapods (four-limbed vertebrates) that typically lay their unshelled eggs in water. Aquatic larvae metamorphose into terrestrial adults. Moist skin supplements lung function. - **Reptiles:** Amniotes (vertebrates with a shelled egg). Scaly skin and amniotic eggs are key adaptations for land. Include snakes, lizards, turtles, crocodiles, and birds. Nonbird reptiles are ectotherms, regulating body temperature through behavior. - **Birds:** Endothermic reptiles with wings and feathers adapted for flight. Honeycombed bones, toothless beaks, and a single ovary reduce weight. - **Mammals:** Endothermic vertebrates with mammary glands and hair. Include monotremes (egg-laying), marsupials (pouched), and eutherians (placental mammals). **The Human Ancestry** Primates likely evolved from small, arboreal, insect-eating mammals. Adaptations for tree-dwelling include limber shoulder joints, agile hands, sensitive fingertips, forward-facing eyes, and extensive parental care. - **The Emergence of Humankind:** Humans and chimpanzees share a common ancestor but diverged about 6-7 million years ago. Bipedalism (walking upright) preceded the evolution of a large brain. *Australopithecus* were small-brained bipeds. *Homo habilis* had a larger brain and used tools. *Homo erectus* was the first hominin to migrate out of Africa. - **Homo neanderthalensis:** Large-brained, tool-using hominins that coexisted with *Homo sapiens* and interbred with some populations. - **Origin and Dispersal of Homo sapiens:** *Homo sapiens* originated in Africa and migrated to other continents. Unique human traits like extended childhood and language contributed to the development of culture. - **Are We Still Evolving?:** While the human body hasn\'t changed much in the last 100,000 years, human populations have continued to adapt to different environments. Examples include adaptations to high altitude, malaria resistance, lactose tolerance, and variations in skin pigmentation. Human cultural evolution continues to be a powerful force. Chapter 18: An Introduction to Ecology and the Biosphere This chapter introduces the study of ecology, the scientific exploration of how organisms interact with each other and their environment. It emphasizes the interconnectedness of life and how human activities are impacting these delicate balances, particularly through global climate change. **Why Ecology Matters:** Ecology helps us understand interconnected processes like the water cycle and pollution, resource availability, and even the shared evolutionary origins of seemingly disparate traits like goosebumps in humans and feather fluffing in birds. **An Overview of Ecology:** Ecology studies interactions from the individual level outwards, encompassing organismal, population, community, and ecosystem ecology. While observation in natural environments is crucial, ecologists also employ controlled experiments and theoretical modeling. Ecology informs environmentalism, but ultimately, solutions require ethical and societal decisions beyond scientific data. **Ecology and Environmentalism:** Human technology has allowed us to inhabit almost every corner of Earth, but our survival remains dependent on finite resources. Human activities have significantly altered the environment, causing pollution, species endangerment, and extinctions. Ecology provides the knowledge base for addressing these issues, but solutions require addressing economic and ethical considerations as well. **Living in Earth's Diverse Environments:** - **Abiotic Factors:** Nonliving components like energy, temperature, water, nutrients, and other factors (wind, fire, salinity, currents, etc.) greatly influence where organisms can survive and thrive. Sunlight powers most ecosystems via photosynthesis, but life also exists in dark environments like deep-sea vents, relying on chemosynthesis. - **Evolutionary Adaptations:** Organisms evolve adaptations to meet the challenges posed by their environment. These adaptations can be physiological (e.g., adjusting blood cell count at high altitude), anatomical (e.g., growing thicker fur in winter), or behavioral (e.g., migration). - **Adjusting to Environmental Variability:** Acclimation is a gradual, reversible physiological adjustment to environmental change. **Biomes:** Biomes are major life zones characterized by vegetation type (terrestrial) or physical environment (aquatic). - **Aquatic Biomes:** Categorized by salinity (freshwater vs. marine) and other factors like water movement and depth. Include lakes/ponds, rivers/streams, wetlands, oceans, intertidal zones, and coral reefs. Key concepts include: photic/aphotic zones, benthic realm, pelagic realm. - **Freshwater Biomes:** Critical for drinking water, irrigation, and industry. Face threats from pollution and damming. - **Marine Biomes:** Vast and diverse, from sunlit coral reefs to dark hydrothermal vents. Threats include overfishing, pollution, and habitat destruction. - **Wetlands:** Transitional biomes between aquatic and terrestrial environments. High biodiversity and important for flood control and water purification. - **Terrestrial Biomes:** Primarily determined by climate (temperature and precipitation). Include tropical forests, savannas, deserts, chaparral, temperate grasslands, temperate broadleaf forests, coniferous forests, tundra, and polar ice. These biomes are influenced by latitude, proximity to water, and landforms like mountains (rain shadow effect). **How Climate Affects Terrestrial Biome Distribution:** Earth's curvature causes uneven heating by the sun, driving global climate patterns. Warm, moist air rises at the equator, causing rainfall, explaining the location of tropical rain forests. Dry air descends at 30° N/S latitude, creating deserts. Temperate zones lie between the tropics and the poles. Oceans and mountains influence local climate. **Human Impact on Biomes:** Human activities have altered ¾ of Earth\'s land surface, primarily for agriculture and development. Deforestation, especially in tropical rain forests, is driven by agriculture, logging, and unsustainable practices. Freshwater resources are increasingly stressed by pollution, overuse, and drought. **Forests:** Human activity has dramatically impacted forest ecosystems, with deforestation being a key concern. Tropical rain forests are being cleared for agriculture and resources like palm oil. Other forests face threats from logging, mining, and pollution. **Fresh Water:** Freshwater ecosystems are highly vulnerable to pollution from agriculture, industry, and sewage. Overuse of groundwater and climate change contribute to water shortages. **Global Climate Change:** - **The Greenhouse Effect and Global Warming:** Greenhouse gases (CO₂, water vapor, methane, etc.) trap heat in the atmosphere. Increasing concentrations of these gases, primarily due to the burning of fossil fuels, are causing a warming trend (global warming). - **The Accumulation of Greenhouse Gases:** Atmospheric CO₂ levels have increased dramatically since the Industrial Revolution, primarily due to human activities. Deforestation contributes by reducing CO₂ uptake. Oceans absorb CO₂, but this is causing ocean acidification. **How Does Climate Change Affect Species Distribution?** Climate change is altering species distribution, with ranges shifting toward the poles or higher elevations. Some species may adapt, but others face extinction. Species in polar regions and on mountaintops are particularly vulnerable. **Effects of Climate Change on Ecosystems:** Climate change disrupts ecological interactions and is shifting biome boundaries. Warmer temperatures and altered precipitation patterns contribute to more frequent and intense wildfires. **Looking to Our Future:** Individuals can reduce their carbon footprint by reducing energy consumption, transportation emissions, and waste generation. Sustainable resource management and international cooperation are crucial for addressing global climate change. Chapter 19: Population Ecology ------------------------------ This chapter explores population ecology, the study of how and why populations change over time. It examines factors that influence population size, density, and growth, and how these principles apply to managing natural resources and understanding human population trends. **Why Population Ecology Matters:** Understanding population ecology is essential for addressing issues such as overfishing, waste generation, resource management, and the impact of growing human populations. **An Overview of Population Ecology:** Population ecology examines the factors that influence population size and dynamics. A population is defined as a group of individuals of the same species living in the same general area. Key features of a population include size, age structure, density, and dynamics (changes in population features over time). This field plays a vital role in applied research for conservation, resource management, and pest control. **Population Density:** Population density is the number of individuals per unit area or volume. It can be