Nanofertilizers for Plant Growth: A Review (PDF)
Document Details
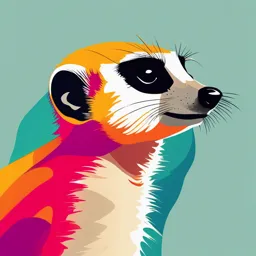
Uploaded by pepinos
Brock University
2024
Natalia A. Semenova, Dmitriy E. Burmistrov, Sergey A. Shumeyko, Sergey V. Gudkov
Tags
Summary
This review examines the use of nanofertilizers as an alternative to traditional mineral fertilizers for improving plant growth. The authors analyze the efficacy of nanoformulations for macro- and micro-elements in a variety of crops. The potential benefits of nanofertilizers, such as targeted delivery and reduced environmental impact, are discussed, alongside potential drawbacks and conflicts in existing data.
Full Transcript
agronomy Review Fertilizers Based on Nanoparticles as Sources of Macro- and Microelements for Plant Crop Growth: A Review Natalia A. Semenova , Dmitriy E. Burmistrov , Sergey A. Shumeyko and Sergey V. Gudkov * Prokhorov Gene...
agronomy Review Fertilizers Based on Nanoparticles as Sources of Macro- and Microelements for Plant Crop Growth: A Review Natalia A. Semenova , Dmitriy E. Burmistrov , Sergey A. Shumeyko and Sergey V. Gudkov * Prokhorov General Physics Institute of the Russian Academy of Sciences, Vavilov St., 38, 119991 Moscow, Russia; [email protected] (N.A.S.); [email protected] (D.E.B.); [email protected] (S.A.S.) * Correspondence: [email protected] Abstract: The necessity for efficacious, sophisticated methodologies to facilitate agricultural intensifi- cation in the context of global population growth is widely accepted. One of the principal methods for enhancing the yield of plant agricultural products is the application of fertilizers. In light of the rapid advancement of nanotechnology over recent decades, the potential of utilizing fertilizing systems based on nanoparticles and nanomaterials—termed “nanofertilizers”—as an alternative to classical mineral fertilizers is increasingly being explored. Due to their unique properties, nanofertilizers demonstrate a number of qualities useful for agriculture. These include high activity, more accurate dosing, targeted delivery of fertilizers to plants, reduced accumulation in soils and groundwater, high durability, and so forth. This review presents a synthesis of data on the efficacy of nanofertilizers over the last decade, focusing on macro-based (N, P, K, Ca, Mg, S) and micro-based (Fe, Zn, Mn, B, Cu, Mo) nanoformulations for agricultural crops. We analyzed over 200 publications, published mainly over the last decade, on the topic of “nanofertilizers”. An analysis of published data on the effectiveness of using nanoparticles as applied fertilizers was carried out, and the effectiveness of using nanofertilizers was compared with traditional chemical fertilizers for a number of elements. Keywords: nanofertilizers; nanoparticles; nanomaterials; macronutrients; micronutrients; plant nutrition; sustainable agriculture Citation: Semenova, N.A.; Burmistrov, D.E.; Shumeyko, S.A.; Gudkov, S.V. Fertilizers Based on Nanoparticles as 1. Introduction Sources of Macro- and Microelements The rapid growth of the global population, the reduction of cultivable land due to for Plant Crop Growth: A Review. environmental problems resulting from the improper exploitation of agricultural areas and Agronomy 2024, 14, 1646. https:// introduction of excess fertilizer, climate change, environmental degradation, the irrational doi.org/10.3390/agronomy14081646 use of nonrenewable resources, and high urbanization rates have collectively led to food Academic Editors: Wenhai Mi, Meng shortages. A significant increase in the total population of the Earth, which is projected Xu and Baskaran Stephen Inbaraj to reach 9.7 billion by 2050 , will lead to an increase in food demand , with the need to increase global grain production by at least 70%. Ways to solve the problems of food Received: 7 June 2024 shortages for agricultural products can be either extensive (through the expansion of arable Revised: 10 July 2024 land), typical for developing countries, or intensive (through the introduction of modern Accepted: 24 July 2024 technologies). Thus, for the global sustainable development of the agro-industrial complex, Published: 27 July 2024 it is necessary to develop an effective system of nutrition and plant protection with minimal damage to the environment. Advances in science and technology could be a potential solution for increasing added Copyright: © 2024 by the authors. value in current production systems. A significant increase in agricultural production Licensee MDPI, Basel, Switzerland. is possible through the use of modern knowledge in the field of nanotechnology to create This article is an open access article effective nutrition and plant protection systems; apply innovative phenotyping methods; distributed under the terms and implement water management and nanopurification systems and systems for the efficient conditions of the Creative Commons use of solar energy by plants, which can be used in precision farming systems; and much Attribution (CC BY) license (https:// more [6–14]. creativecommons.org/licenses/by/ Until recently, it was believed that applying more fertilizer could increase crop yields 4.0/). around the world. However, this approach to agriculture can cause serious threats to Agronomy 2024, 14, 1646. https://doi.org/10.3390/agronomy14081646 https://www.mdpi.com/journal/agronomy Agronomy 2024, 14, 1646 2 of 48 the environment in the form of eutrophication and the pollution of fresh water sources, which, in turn, can negatively affect aquatic flora and fauna and can also be dangerous for the local population. Moreover, the intensive application of fertilizers increases the concentration of nitrates in groundwater to toxic levels. It is known that most of the bulk fertilizers applied directly to the soil and irrigation system enter the atmosphere and are washed away by wastewater, adversely affecting ecosystems. In addition, the low efficiency of traditional fertilizers (20–50%) and the costly increase in their application rates have prompted researchers around the world to develop and promote the use of nanofertilizers [12,18], which can reduce nutrient deficiencies due to their conversion into biologically inaccessible forms. Nanomaterials differ from their bulk counterparts in a number of physical and chemi- cal properties that give them unique properties and high reactivity due to their high surface area-to-volume ratio. Nanofertilizers are nanoparticles (1–100 nm in size) or emulsions containing one or more macro- and/or microelements. However, this definition is not entirely accurate. Nanofertilizers also include materials larger than 100 nm, modified with nanosized particles, also called nanostructured bulk fertilizers. Nanofertilizers can reduce the loss of nutrients and reduce the load on ecosystems. Depending on the chemical composition, nanofertilizers can be monocomponent [23,24] or multicomponent (multin- utrient) [25–27]. Chemical composition determines the basic optical, magnetic, electrical, and catalytic properties of nanoparticles. The size and shape of nanoparticles also influences the physical and chemical properties and determines their unique action [29–31]. Nanofertilizers have shown their effectiveness on many crops, including grains [32–34], vegetables [31,35,36], and industrial crops [37–40], helping to increase growth rates, the concentration of photosynthetic pigments, and productivity. Some nanofertilizers allow the dosed release of nutritional components into the substrate, providing a prolonged effect [41–43]. Nanotechnologies are effective as stress protectors, allowing the mitigation of harmful effects of negative factors (salinization, heavy metal pollution) [25,33,44]. For example, under salinity conditions, nanoparticles accelerate the metabolism of sugars by increasing α-amylase activity, restore redox imbalance and the content of reactive oxygen species (ROS) by reducing lipoxygenase activity, influence the ion profile of plants, improve nutri- ent uptake and nitric oxide production, limit Na accumulation; optimize the K to Na ratio, and increase plant resistance to stress [45–47]. These beneficial effects may be explained by changes in the relevant genes. The reduction of the toxic effects of heavy metals by the use of nanoparticles has similar mechanisms: the reduction of oxidative stress (reduction of ROS synthesis); improvements in nutrient absorption, the expression of relevant genes, chlorophyll synthesis, and photosynthetic efficiency; and increases in membrane stabil- ity [48,49]. In addition, nanoparticles of essential minerals help enhance the development of beneficial microflora by stimulating enzyme activity and the synthesis of exopolysaccha- rides and secondary metabolites. Beneficial microorganisms, in turn, participate in the transformation of nanoparticles in the rhizosphere, reducing toxic effects. However, it should be noted that the simultaneous development of pathogenic microflora also occurs. NPs can also exhibit a negative, inhibitory effect on beneficial microflora and other factors affecting cell metabolism and the stability of cell membranes due to their chemical nature or high concentrations. The use of biodegradable nanomaterials with a polymer coating can prevent the negative consequences of using NPs. Nanotechnology has already revolutionized agriculture. According to forecasts, by 2030, the average annual growth of the nanotechnology market will be 15%. Many new studies are devoted to the development of nanoparticles and nanomaterials that can be used to increase the rate of seed germination [53,54], as a source of nutrients , for the biofortification of plants [11,56], and to control growth, yield, and other physiological parameters. However, there are conflicting data on the effect of nanofertilizers on crop yields. Thus, some studies confirm the effectiveness of using nanofertilizers, which allow Agronomy 2024, 14, 1646 3 of 48 one to obtain a 30% increase in yield compared to bulk analogs. Others, on the contrary, show the absence of clear advantages of their use. The use of nano-sized fertilizers, despite the obvious positive effects obtained on many types of plants, can also have negative consequences for the environment, including the toxicity of some types of nano-fertilizers for soil microflora, nano-pollution in the soil, danger to beneficial insects, etc. [23,60], which must be taken into account when developing timing, methods, and application rates. The main purpose of this review is to summarize and analyze recent developments in the field of using nanoparticles as fertilizers to increase the productivity of agricultural crops. Particular attention is paid to quantitative effects that increase the growth and productivity of cultivated plants, taking into account side effects; factors influencing their effectiveness are also considered. Possible directions for further research and prospects for the successful implementation of nanofertilizers in sustainable agriculture are identified. A comprehensive literature search was conducted using the following scientific search engines: Google Scholar, PubMed, and Scopus. This review is primarily based on a critical analysis of scientific publications since 2012. The primary search terms employed in the lit- erature review were “nanofertilizers”, “use of nanoparticles as fertilizers”, “nanoparticles”, “nanoparticles as sources of micronutrients”, “nanoparticles as sources of macronutrients”, and so forth, as well as various combinations thereof. In total, the analysis encompassed more than 200 sources. It should be noted that the selection of specific publications was not a criterion for analysis; only those works that were suggested by the search engines were included. 2. Nanoparticles in Agriculture Nanoparticles (NPs) are an innovative tool to solve many emerging global problems, including in the agricultural industry. A nanoparticle is any particle whose one characteris- tic size is in the range of 1–100 nm. The small size of nanoparticles makes it possible to more effectively exhibit properties such as ion exchange, diffusion, ion adsorption, and complexation. This is primarily a consequence of the high proportion of atoms present on the surface with an increased proportion of centers and operating with higher reactivity towards adsorption and electrochemical interactions. Due to their small size, nanopar- ticles can easily pass through cellular barriers directly (5–20 nm). However, research does not exclude the absorption of dissolved nanoparticles from the soil solution in the form of ions. Like the ions of conventional bulk fertilizers, they are not absorbed selectively, but their dissolution rate and quantity per unit volume are much higher. Nanoparticles, as well as nanomaterials (NMs), are potential candidates for imple- mentation in agriculture. Nanomaterials perform the functions of delivering forms of ions available to plants in the required volume and order, ensure the expression of plant genes on nanomatrices, and provide targeted delivery of molecules and genes to living cells ; these features of nanomaterials open up wide possibilities for their use in point farming and preventing the accumulation of excess nutrients in soils to reduce environmental load. The main modern direction of development of nanotechnology in the agricultural sector is the development of environmentally friendly application technologies of nanofer- tilizers that can provide effective delivery of ions (for soluble NPs) and nutrients to plant cells, as well as for genome transformation and the stimulation of temporary or stable gene expression to produce plants with the desired properties both in vitro and in vivo (resistance to drought, phytopathogens, accelerated growth cycles, etc.) [62,65,66]. 3. Nanofertilizers Nanofertilizers (NFs) are nanomaterials that contain macro- and/or micronutrients essential to plants or are used as nanocarriers for conventional chemical fertilizers, thereby facilitating the effective delivery of nutrients. Nanofertilizers have been demonstrated to be more effective than conventional chem- ical fertilizers due to their unique mechanisms of action, which enhance their efficacy, 3. Nanofertilizers Nanofertilizers (NFs) are nanomaterials that contain macro- and/or micronutrients essential to plants or are used as nanocarriers for conventional chemical fertilizers, thereby Agronomy 2024, 14, 1646 facilitating the effective delivery of nutrients. 4 of 48 Nanofertilizers have been demonstrated to be more effective than conventional chemical fertilizers due to their unique mechanisms of action, which enhance their effi- reduce nutrient cacy, reduce losses,losses, nutrient and minimize environmental and minimize degradation environmental (Figure degradation 1) [67,68]. (Figure For 1) [67,68]. example, on sandy For example, soils,soils, on sandy nanocomposite fertilizers nanocomposite release fertilizers 78% more release nitrogen 78% more than bulk nitrogen than fertilizers.. bulk fertilizers Figure1.1.Advantages Figure Advantagesof ofNPs NPsallowing allowingthem themtotopenetrate penetrateplants plantsmore moreeasily. easily.The Thefigure figurewas wascreated cre- ated using BioRender web application https://app.biorender.com/ (accessed on 5 February using BioRender web application https://app.biorender.com/ (accessed on 5 February 2024). 2024) Regarding Regarding the the mechanisms mechanisms of of penetration penetration of of nanofertilizers nanofertilizers intointo plant plant cells, cells, small small particle particle sizes sizes and and significant significant increases increases in in their their surface surface area area contribute contribute to to more moreefficient efficient absorption absorption.. Moreover, Moreover, nanofertilizers nanofertilizers are are able able to topenetrate penetratedirectly directlyinto intocells, cells,which which makes it possible to reduce energy costs for their absorption and delivery, makes it possible to reduce energy costs for their absorption and delivery, or even avoid or even avoid them themcompletely completely[71,72]. [71,72]. Like Likeconventional conventionalfertilizers, fertilizers,nanofertilizers nanofertilizers areare dissolved dissolved in the soilsoil in the solution for for solution direct di- absorption by plants. rect absorption However, by plants. However,due due to their small to their particle small size,size, particle theirtheir solubility is usually solubility is usu- higher than than ally higher that of traditional that fertilizer of traditional counterparts. fertilizer counterparts. Nanofertilizers Nanofertilizers are more are moreeffective effec- compared tive compared to conventional ones; they can reduce nitrogen losses due to processessuch to conventional ones; they can reduce nitrogen losses due to processes such as asleaching, leaching,emissions, emissions, andand long-term long-term uptake uptake by by soil soil microorganisms microorganisms [66,73].[66,73]. Moreover, Moreover, controlled-release controlled-releasenanofertilizers nanofertilizerscan canimprove improvefertilizer fertilizeruse useefficiency efficiency and and reduce reduce soil degra- soil deg- dation by reducing the toxic effects that are associated with the radation by reducing the toxic effects that are associated with the overuse of traditionaloveruse of traditional chemical chemicalfertilizers fertilizers..Some Somestudies studieshavehavelooked lookedatatthe use the useofof time-release time-release nanoencapsu- nanoencap- lated sulated fertilizers. For example, biodegradable polymeric chitosan nanoparticles(~78 fertilizers. For example, biodegradable polymeric chitosan nanoparticles (~78nm)nm) have have been used for the controlled release of NPK fertilizer sources such as urea,calcium been used for the controlled release of NPK fertilizer sources such as urea, calcium phosphate, phosphate,and andpotassium potassium chloride chloride. Other. Other nanomaterials nanomaterials suchsuch as kaolin and polymeric as kaolin and poly- biocompatible nanoparticles can also be used for this purpose meric biocompatible nanoparticles can also be used for this purpose.. According to the classification of Liu and Lal, 2015, depending on the composition, According to the classification of Liu and Lal, 2015, depending on the composition, nanofertilizers are divided into four categories: macronutrient nanofertilizers, micronutri- nanofertilizers are divided into four categories: macronutrient nanofertilizers, micronu- ent nanofertilizers, nutrient-loaded nanofertilizers, and plant growth-enhancing nanomate- trient nanofertilizers, nutrient-loaded nanofertilizers, and plant growth-enhancing nano- rials. We consider the first two categories, the mechanism of action of which can be materials. We consider the first two categories, the mechanism of action of which can comparable to the use of bulk analogs. be comparable to the use of bulk analogs. 3.1. Nanofertilizers: Sources of Macronutrients Nanofertilizers aimed at delivering macroelements chemically consist of one or more nano-sized macroelements necessary for crops for various metabolic processes of growth and development, as well as for mitigating stress reactions. Traditionally, plant macronutrients include nitrogen (N), phosphorus (P), potassium (K), calcium (Ca), mag- nesium (Mg), and sulfur (S). The most common agrochemical chemical forms for re- 3.1. Nanofertilizers: Sources of Macronutrients Nanofertilizers aimed at delivering macroelements chemically consist of one or more nano-sized macroelements necessary for crops for various metabolic processes of growth and development, as well as for mitigating stress reactions. Traditionally, plant mac- Agronomy 2024, 14, 1646 5 of 48 ronutrients include nitrogen (N), phosphorus (P), potassium (K), calcium (Ca), magne- sium (Mg), and sulfur (S). The most common agrochemical chemical forms for replen- ishing the listed elements are NH4NO3, (NH4)2SO4, (NH4)2CO3, NH4NO3, Ca(NO3)2, and plenishing KNO the listed elements are NH4 NO 3 (nitrogen); Ca(H2PO4)2·H2O, Ca(H 2PO3 ,4(NH )2·H24O, )2 SO (NH 4 , 4(NH )2HPO 4 )24CO , and3 , NH KH42NO , Ca(NO3 )2 , PO43(phospho- and KNO (nitrogen); Ca(H PO ) · H O, Ca(H rus); KCl, KNO3, K2SO4, KH2PO4, and K-MgSO4 (potassium); CaCO3, Ca(NO 3 2 4 2 2 2 PO 4 ) 2 · H 2 O, (NH 4 ) 2 HPO 4 , and KH2 PO 3)2,4 (phosphorus); KCl, KNO , K SO , KH PO , and Ca(H2PO4)2·H2O, and CaSO4·2H2O (calcium); MgSO4, MgO, MgCl2, Mg(NO3)2, and 3 2 4 2 4 K-MgSO 4 (potassium); CaCO 3 , Ca(NO 3 )2 , Ca(H CaMg(CO 2 PO ) · H O, and 34)22(magnesium); 2 CaSO · and4 MgSO2H O (calcium); MgSO 2 4, NH4)2SO4, (NH4)42SO4, FeSO4, and , MgO, MgCl , Mg(NO ) 2 K2SO4 (sulfur). 3 2 , and CaMg(CO 3 )2 (magnesium); Scientific interest in the and MgSO4of possibility , NH using4 )2 SO 4 , (NH4 )2 SOas nanoparticles 4 , FeSO 4 , andto fertilizers Kdeliver 2 SO4 (sulfur). mac- Scientific interest in the possibility of using nanoparticles roelements to plants has grown several times over the past 10 years (Figure 2). An analysis as fertilizers to deliver ofmacroelements published research to plants resultshasshowed grown that several timesofover the bulk the work the past 10 yearsto(Figure is devoted 2). An nanofertiliz- analysis of published research results showed that the bulk of the work is devoted to ers containing nitrogen, which is a key element necessary for plant growth. Table 1 shows nanofertilizers containing nitrogen, which is a key element necessary for plant growth. shortened results of a number of modern works demonstrating the production and effec- Table 1 shows shortened results of a number of modern works demonstrating the produc- tiveness of using nanofertilizers as sources of macroelements. A more detailed description tion and effectiveness of using nanofertilizers as sources of macroelements. A more detailed of the effectiveness of using nanofertilizers for the delivery of each of the main macroele- description of the effectiveness of using nanofertilizers for the delivery of each of the main ments (N, P, K, Ca, Mg, S) is presented below in the corresponding subsections. macroelements (N, P, K, Ca, Mg, S) is presented below in the corresponding subsections. Figure 2. The dynamics of the number of publications containing the keywords “Nitro- Figure 2. The dynamics of the number of publications containing the keywords “Nitrogen/phos- gen/phosphorus/potassium/calcium/magnesium/sulfur” phorus/potassium/calcium/magnesium/sulfur” and “nanoparticles and “nanoparticles plantData plant fertilizers”. fertilizers”. taken DataPubMed from taken from PubMed database database https://pubmed.ncbi.nlm.nih.gov/ https://pubmed.ncbi.nlm.nih.gov/ (accessed (accessed on 29 on 29 April 2024). April 2024). Agronomy 2024, 14, 1646 6 of 48 Table 1. The application of nanoparticles as sources of macronutrients and their effect on the growth, photosynthesis, and yield of crops. Experimental Optimal Fertilizer Composition Method Particle Size, nm Object/Plant Culture Experiment Results Refs. Conditions Concentration Wet chemical method with encapsulation of The nanoparticles showed slow release of urea-modified HA nitrogen even at day 60 compared to the Three types of soils with nanoparticles into control. The control released nitrogen earlier U-modified HA NPs 100 × 150 Nitrogen release different pH (4.2, 5.2, - micro/nanoporous and in large quantities until about day 30, and 7.0) cavities of Glyricidia but this was followed by the release of sepium (Jacg.) Kunth nitrogen in low and patchy quantities. Walp. under pressure ↑ chlorophyll content (+13–19% depending on the variety) ↑ number of shoots (+5–11%) Biosynthesis from ↑ spike length (+8–28%) shrimp waste by Triticum aestivum L. var. Foliar spraying in a Chitosan NPs 10–20 14 L·ha−1 ↑ number of spikelets (+7–8%) Penicillium oxalicum Misr-1 and Gemaiza-11 field experiment ↑ spike weight (+5–13%) Currie and Thom ↑ 1000-grain weight (+7–16%) ↑ grain yield (+27–31%) Plant height did not change ↑ number of shoots per plant (+11%) Cultivation in a growth ↑ FW (+30%) chamber in a substrate ↑ number of spikelets (+30%) consisting of a 1:1 Triticum durum Desf. 15 kg·ha−1 ↑ spike weight (+36%) mixture of soil and sand ↑ 1000-grain weight (+23%) under simulated ↑ Grain quantity (+27%) sunlight ↑ protein content (+13%) ↑ total root length (2.5 times), number Cultivation in an (2.4 times), and surface area (2.3 times) U-doped amorphous aerated hydroponic Batch method 13.5 ↑ Ca, P, and S Ca3 (PO4 )2 -NPs Cucumis sativus L. solution followed by 3.7 mM ↑ N uptake (1.7 times) provocation of nitrogen ↑ gene expression CsDUR3 (5 times) starvation for 7 days SPAD level did not change ↑ N content in berries (+28.6%) ↑ amino acid concentrations in plants ↑ arginine concentrations in the musts Vitis vinifera L. Field experiment 0.4 kg·ha−1 (+21%) ↓ proline concentration compared to commercial urea treatment (6 kg·ha−1 ) Agronomy 2024, 14, 1646 7 of 48 Table 1. Cont. Experimental Optimal Fertilizer Composition Method Particle Size, nm Object/Plant Culture Experiment Results Refs. Conditions Concentration ↑ FW (+42%) ↑ nutrient content ↑ chlorophyll content (+12.7%) ↑ ETR (+39%) Nitrogen-doped CDs Hydrothermal method 2.6 Lactuca sativa L. Hydroponic culture 100 mg·L−1 ↑ light energy conversion efficiency (Y (II)) (+31%) ↑ photosynthesis rates—Rubisco activity (+61%) ↑ plant height (+13%) ↑ spike length (+24%) ↑ spike weight (+79%) ↑ number of spikelets (3.6 times) Cultivation in pots ↑ Grain quantity (+2.8) Chemical method with Triticum aestivum L. HA-U-NPs 38.7 under controlled 75 kg·ha−1 ↑ Mg (2.4 times), N (2.4 times), P (2.6 times), centrifugation Pusa HD 3086 conditions K (1.8 times), Ca (5.5 times), and Fe (3 times) in grains ↑ protein content (2 times) ↑ phospholipid concentration (+51%) ↑ proline concentration (+12%) ↑ plant height (+13%) ↑ spike length (+24%) ↑ spike weight (+79%) ↑ stem weight (+56%) ↑ number of spikelets (3.2 times) Cultivation in pots Chemical method with Triticum aestivum L. ↑ Grain quantity (+2.5) Mg-doped HA-U-NPs 28.3 under controlled 75 kg·ha−1 centrifugation Pusa HD 3086 ↑ Mg (3 times), N (2.7 times), P (3.8 times), K conditions (1.9 times), Ca (5.4 times), and Fe (3.7 times) in grains ↑ protein content (2 times) ↑ phospholipid concentration (+28%) ↑ proline concentration (+20%) Agronomy 2024, 14, 1646 8 of 48 Table 1. Cont. Experimental Optimal Fertilizer Composition Method Particle Size, nm Object/Plant Culture Experiment Results Refs. Conditions Concentration ↑ plant height (+10%) ↑ spike length (+18%) ↑ spike weight (+61.5%) ↑ stem weight (+40%) Cultivation in pots Chemical method with Triticum aestivum L. ↑ number of spikelets (2.8 times) Zn-doped HA-U-NPs 20.8 under controlled 37.5 kg·ha−1 centrifugation Pusa HD 3086 ↑ Grain quantity (+2.5) conditions ↑ N (2.2 times), P (2.3 times), Mg (2.1 times), K (1.8 times), Fe (2.8 times), and Ca (4.2 times) in grains ↑ protein content (+50%) ↑ growth rate (+32.6%) One-step wet chemical ↑ seed yield (+20.4%) HA-NPs 8–22 Glycine max L. Greenhouse conditions 21.8 mg·L−1 P method ↑ shoot DW (+18.2%) ↑ root DW (+41.2%) ↑ height (+12.6%) ↑ leaf surface area (+27.7%) ↑ FW (+53%) ↑ green cob yield, t/ha (+29.4%) Zea mays L. yellow 50 mg·L−1 ↑ chlorophyll concentration (+52%) cultivar ↑ protein content (+22.1%) ↑ TPC (+12.9%) ↑ total flavonoids (+33.3%) Chemical method with Foliar application in ↑ total indoles (+32.4%) HA-NPs 100 [38,78] centrifugation field conditions ↑ height (+17.0%) ↑ leaf surface area (+18.6%) ↑ FW (+58.8%) Zea mays L. white ↑ green cob yield, t/ha (+45.2%) 100 mg·L−1 cultivar ↑ chlorophyll concentration (+53%) ↑ TPC (+28.0%) ↑ total flavonoids (+10.0%) ↑ total indoles (+25.5%) Wet chemical deposition In Ultisol (pH 4.7): and subsequent surface Cultivation in pots for ↑ FW (6.4–11.6 times) functionalization with 35 days using two ↑ P in shoots (1.5 times) and Ca (2 times) HA-NPs with different glycine or dibasic 25.7 Helianthus annuus L. phosphorus-deficient 150 mg·kg−1 P ↓ Fe (8 times) and Zn (2 times) [79,80] surface charges ammonium citrate to soils (Ultisol and In Vertisol (pH 8.2): obtain positively or Vertisol) NPS did not significantly affect biomass negatively surface ↓ Ca (3 times) charged-nano-apatite Agronomy 2024, 14, 1646 9 of 48 Table 1. Cont. Experimental Optimal Fertilizer Composition Method Particle Size, nm Object/Plant Culture Experiment Results Refs. Conditions Concentration All K-NP concentrations ↑ number of spikes (1.5–2 times) Field conditions, sandy ↑ yield per hectare (2–3 times) Green synthesis using Triticum aestivum L. 20, 40, and K-NPs 21–30 loam soil, and foliar ↑ protein content (30–50%) Morus alba L. extract (var. HD2967) 60 mg·L−1 treatment ↑ photosynthetic pigments (+50–60%) compared to the bulk analog (K2 SO4 ) and control (without added potassium) Clayey and Provides controlled release of P and N over a clayey-loamy soils; longer period compared to traditional DAP. DAP with KFeO2 -NP Chemical (sol–gel) Incubation studies on 7–18 assessment of the 10% nano-coating ↑ release pattern of K (+8–12.5%), P nano-coating method clay and loamy soils dynamics of the release (7–10 times), N (3 times), and Fe (2.5–3 times) of N, P, K, and Fe in 60 days depending on soil type ↑ Ca (+0.4%), N (+0.9%), P (+0.04%), and K (0.5%) ↑ total branches (+10%) Greenhouse study, sand ↑ leaf surface area (+21%) Arachis hypogaea L. var. substrate, 80 days of 160 mg·L−1 Ca ↑ DW (+19%) Luhua No. 4 cultivation ↑ soluble sugar content (+33%) Heterogeneous phase ↑ protein content (+90%) precipitation of compared to negative control and results are CaCO3 -NPs Ca(OH)2 after 20–80 equivalent to Ca(NO3 )2 treatments hydration of CaO and subsequent calcination ↑ plant height (+10%) ↑ branching number (+9.5%) ↑ growth rate (+16%) Two-year experiment in ↑ seed yield (1.6 times) Arachis hypogaea L. field conditions, foliar 200 mg·L−1 ↑ 100-seed weight (1.6 times) spraying ↑ protein content (+10.5%) ↑ oil (+6%) ↑ P (+12%) and K (2.5 times) Agronomy 2024, 14, 1646 10 of 48 Table 1. Cont. Experimental Optimal Fertilizer Composition Method Particle Size, nm Object/Plant Culture Experiment Results Refs. Conditions Concentration ↑ fruit firmness, TA, TSS, TPC, TAA, and fiber content in fruits ↓ internal browning ↓ FW, total soluble solids compared Khazra nano-chelated Spraying 70 days after to control calcium (Khazra Sodour Malus domestica Borkh. full flowering, a month ↓ polygalacturonase activity, pectin - - 25 mg·L−1 Ahrar Shargh Co., cv. Red Delicious before harvest, and methylesterase, and β-galactosidase in fruits Tehran, Iran) during storage that were treated with both CaCO3 -NPs and CaCl2 With increasing shelf life, the quality of fruits treated with CaCO3 -NPs was higher than that of fruits treated with CaCl2 ↑ germination rate (+20%) ↑ plant height (+38%) ↑ FW (+20%) ↑ protein soluble content (+41%) Cultivation in the field, 125–250 mg·L−1 Sr0.96 Mg0.02 Ca0.02 Fe12 O19 , Sol–gel autoignition treatment at the stages ↑ chlorophyll concentration (+33–42%) 42.4 Hordeum vulgare L. compared to untreated control SrMgCa nano-HF method of germination and growth separately Higher doses ↓ growth parameters ↑ Fe (20 times), Ca (18 times), Mg (3 times), 500 mg·L−1 and Sr (60 times) in leaves ↑ yield (+14%) Chemical method with Mg-NPs+ FeSO4 20 85 days of cultivation in 0.5 + 0.5 g·L−1 ↑ Fe (+27%) and Mg (+7%) in leaves centrifugation Vigna unguiculata ssp. field, application of Mg ↑ plasma membrane stability unguiculata and/or Fe 56 and ↑ Fe (+17%) and Mg (+9%) in leaves Chemical method with 72 days after sowing Mg-NPs 100 0.5 g·L−1 ↑ SPAD (+5%) centrifugation ↓ yield (2.7 times) ↑ stem FW (+23%) 60 days of cultivation ↑ root FW (+20%) after planting in a ↑ pod production (+18%) greenhouse; foliar plant ↑ total polyphenols (+21%) Green bean Phaseolus treatments every ↑ flavonoid content (970–1703 mg Mg-NPs - - 0.05–0.1 g·L−1 vulgaris L. cv. ‘Stike’ 10 days (5 in total); a catechin/100 g FW, not determined without mixture of vermiculite treatment) and perlite in a 2:1 ratio ↑ DPPH (+35%) was used as a substrate when using 50 mg·L−1 Mg-NPs compared to MgSO4 at the same concentration Agronomy 2024, 14, 1646 11 of 48 Table 1. Cont. Experimental Optimal Fertilizer Composition Method Particle Size, nm Object/Plant Culture Experiment Results Refs. Conditions Concentration ↑ seed germination (3 times) ↑ early ripeness (3 weeks) Research in the field ↑ number of productive shoots S-NPs Chemical method Triticum aestivum L. and greenhouse, seed 3.4 g·L−1 ↑ Grain quantity treatment time 15 min ↑ grain weight and yield (+30–100% depending on the variety) ↑ pathogen resistance ↑ root FW (+73%) ↑ shoot FW (+35%) S-NPs 20–40 200 mg·L−1 ↑ linear electron flow ↑ quantum yield of photosystem II Application to soil as a ↑ relative chlorophyll content Solanum lycopersicum L. multifunctional Chemical method ↑ root FW (+81%) fertilizer ↑ shoot FW (+50%) ↑ linear electron flow S-NPs modified with 200 mg·L−1 ↑ quantum yield of photosystem II stearic acid (cS) ↑ relative chlorophyll content ↑ content of tryptophan, tomatidine, and scopoletin in leaves ↑ content of available S in the soil ↑ DW (+11–12%) ↑ gain yield (+15%) Aqueous precipitation Helianthus annuus L. var. S-NPs 35–45 Field experiment 40 kg·ha−1 S ↑ oil content (+14,7%), compared to plants method KBSH 42 fertilized with gypsum ↑ sulfur release (+7 days compared with gypsum) HA—hydroxyapatite; FW—fresh weight; DW—dry weight; U—urea; ETR—electron transfer rate; TPC—total phenolic content; TA—titrable acidity; TAA—total antioxidant activity; TSS—total soluble solids; HF—hexaferrites; DAP—diammonium phosphate; CDs—carbon quantum dots; DPPH—2.2-diphenyl-1-picrylhydrazyl; SPAD—soil plant analysis development. ↑: indicator increase; ↓: indicator decrease. Agronomy 2024, 14, 1646 12 of 48 The macronutrient requirements of crop plants are increasing as the demand for more food supplies increases for the ever-growing world population. The demand for macronu- trients is expected to increase to 263 million tons by 2050. To reduce the amount of fertilizer applied that harms the environment, nanofertilizers have been proposed that have increased application efficiency compared to conventional chemical fertilizers. Developed nanofertilizers containing macronutrients are constantly being improved by scientists and technicians around the world, and their use allows for greater growth and productivity of agricultural crops. Thus, nanofertilizers with macroelements are designed to not only increase the efficiency of agricultural production but also reduce production costs and, as a result, are an economical alternative to existing traditional fertilizers. A detailed description of nanofertilizers in the context of each key plant macronutrient (N, P, K, Ca, Mg, S) is provided in the relevant sections below. 3.1.1. NFs: Nitrogen Sources Nitrogen is a crucial nutrient involved in numerous life processes of cultivated plants. Nevertheless, the widespread use of mineral nitrogen fertilizers is associated with a signifi- cant drawback: more than half of the nitrogen applied is lost to the environment. Part of the applied nitrogen enters the atmosphere in the form of nitrogen oxide, which is one of the harmful greenhouse gases that contribute to global warming. In order to enhance the efficacy of nitrogen utilization, a range of strategies have been implemented, with nitrogen nanofertilizers occupying a distinctive position [41,91,92]. For example, the slow release of nitrogen was observed when urea (ammonium) was applied to zeolite chips. Similarly, urea-modified hydroxyapatite nanoparticles were pressure-encapsulated in the cavity of softwood Gliricidia sepium (Jacq.) Steud. and tested to release nitrogen slowly and steadily into the soil. Interestingly, nitrogen supply with this strategy was found to be optimal for up to 60 days compared to conventional nitrogen fertilizers, which provided a greater nitrogen supply to the plants initially and a very low supply at a later stage, up to 30 days. Replacing the traditional use of nitrogen mineral fertilizers with nanosubstitutes has recently shown effectiveness in obtaining high crop yields. For example, the use of chitosan-based nanoparticles as a replacement for mineral nitrogen fertilizers has proven its effectiveness in growing soft wheat (Triticum aestivum L.); the yield indicators when using these nanofertilizers at a concentration of 14 L·ha−1 were not inferior to the application of mineral fertilizers (240 kg·ha−1 ). Thus, nanofertilizers make it possible, if not to completely replace mineral ones, then to reduce their application, which would reduce the environ- mental load on the environment and make a certain contribution to the development of sustainable agriculture. Also, in studies on the effect of nanoparticles of amorphous calcium phosphate en- riched with urea (U-ACP) on the growth and yield of durum wheat (Triticum durum Desf.), it was found that the yield and quality of plants treated with nanofertilizers (15 kg·ha−1 U-ACP + 60 kg·ha−1 diammonium hydrogen phosphate) were similar to those obtained using a conventional mineral fertilizer application protocol (diammonium hy- drogen phosphate 150 kg·ha−1 ). However, the nitrogen content was slightly reduced (by 40%), and there were more grains in a spike, but they were smaller than small grains compared to the standard growing protocol, which was due to the greater availability of nitrogen in the nanofertilizer, which affected the number of inflorescences formed and grains set. The treatment of cucumber plants (Cucumis sativus L.) with U-ACP at a nitrogen concentration equivalent to half the concentration of urea applied in the control treatment showed better results in nitrogen assimilation, while no significant changes were observed in the accumulation of biomass and chlorophyll. In experiments, an increased accumulation of calcium and phosphorus in plants was noted, since this nanofertilizer also contains Ca and P, and there is evidence of the possibility of using its base (hydroxyap- atite nanoparticles) as a source of phosphorus. Grapes harvested from plants treated with U-ACP provided levels of yeast-available nitrogen similar to increased doses of urea Agronomy 2024, 14, 1646 13 of 48 (6 kg·ha−1 ), despite a decrease in the dose of nitrogen applied. The concentration of amino acids was higher than in the control and when 3 kg·ha−1 of urea was added. Nanofertilizers provided a high concentration of arginine in the wort, but a decrease in proline compared to the application of 6 kg·ha−1 of urea. U-ACP is essentially a multi-component fertilizer, although the majority of researchers consider it to be a nitrogen fertilizer. Based on ACP, nanoU-NPKs have also been developed to provide a slow, gradual release of macronutrients. The use of nanoU-NPK has been demonstrated to reduce the amount of nitrogen supplied to plants by 40% compared to the application of mineral fertilizers while maintaining the weight of the yield obtained. The efficacy of the application of urea nanohybrids (hydroxyapatite-urea HAU, hydroxyapatite-urea with added Mg-MgHAU, hydroxyapatite-urea with added Zn-ZnHAU) in doses equal to 25 and 50% of nitrogen compared to a control (urea 150 kg·ha−1 ) was also demonstrated. The application of such doses to wheat (Triticum aestivum L.) resulted in an increase in growth and yield, improved plant uptake of nutrient elements (N, P, K, Ca, Mg, Fe, etc.), and an increase in protein and phospholipid content in the grain. Consequently, the application of nanohybrids can result in a reduction of up to 75% in the requirement for nitrogen fertilizer, without affecting the growth or quality of the plants. The urea in nanohybrid fertilizers is released at a gradual pace, providing a prolonged period of action. In terms of agronomic outcomes, plants treated with suspension nanohybrids demonstrated superior performance compared to those treated with granular mineral fertilizers. The incorporation of NPK fertilizer into a hydrogel nanocomposite network results in a delayed release of nutrient elements, offering a promising avenue for the optimization of fertilizer use and water conservation in sustainable agriculture. A comprehensive assessment of the use of nitrogen nanofertilizers on pastures was also carried out and optimal application parameters and problems associated with their use were established. It was found that the most effective way to apply nitrogen- containing nanofertilizers is foliar treatment. However, the use of foliar treatments requires special conditions for their use (absence of direct sunlight, precipitation during the treatment period, strict adherence to the concentration) and, if not observed, the treatments can cause a number of undesirable consequences, such as leaf burns and growth inhibition. In addition, the costs of production and multiple applications of fertilizers may not be recouped, and the mechanisms for converting nanoparticles into ionic forms are not fully understood, which poses a potential threat to ecosystems. Therefore, large-scale field experiments and the standardization of nanofertilizer application methods are necessary. When assessing the impact of six different foliar treatments (50 kg·ha−1 ) with bulk and nano-nitrogen-based fertilizers (granular and dissolved urea, ammonium nitrate solution, and nanoformulations based on nitrates, urea, and ammonium) on the dynamics of ammonia and nitrous oxide emissions from pastures, it was found that NH3 volatilization was the main pathway of N loss (2–51% of N applied). Higher emissions were observed when using NH4 foliar formulations and lower emissions were observed