Aging: Lecture Notes (BCH 301)
Document Details
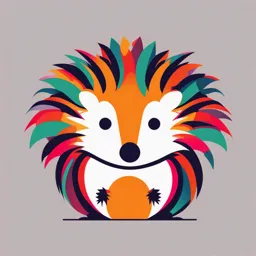
Uploaded by TroubleFreeThallium9798
Edo University Iyamho
Dr. L.O. Iserhienrhien
Tags
Summary
These lecture notes cover the topic of aging, exploring various aspects of the process, including the relationship between aging and disease, biological and chemical theories of aging, hallmarks of aging such as genomic instability and telomere shortening, and interventions to delay aging. The document addresses the impact of aging on several body tissues and organs.
Full Transcript
TOPIC: AGEING (BCH 301) LECTURER: Dr. L.O. Iserhienrhien OBJECTIVES At the end of this lecture, the students should be able to: describe the relationship between aging and disease. differentiate between biological and chemical theories of aging. e...
TOPIC: AGEING (BCH 301) LECTURER: Dr. L.O. Iserhienrhien OBJECTIVES At the end of this lecture, the students should be able to: describe the relationship between aging and disease. differentiate between biological and chemical theories of aging. explain the precepts of the free radical theory of aging, including identification of characteristic oxidation products that accumulate in long-lived proteins with age. outline the evidence that the rate of mutation of DNA is an important determinant of the rate of aging. describe the etiology and pathology characteristic of several diseases of accelerated aging. Overview Aging is a natural phenomenon. It is a is characteristic of all multicellular organisms. The medical branch dealing with age related diseases, is called Geriatrics or Gerontology. Aging may be defined as the time-dependent deterioration in function of an organism. It is seen as a gradual decline in adaptation of the organism to its normal environment, following the onset of reproductive maturity. Ageing is associated with accumulated damage to cells that, over time, weakens the immune system, diminishes the body’s capacity to repair itself and increases the risk of developing a host of different diseases. Excluding genetic defects, childhood disease and accidents, complex systems like humans survive until about age 50 with limited maintenance requirements or risk of death, then they become increasingly frail and their death rate increases, reaching a maximum at about age 76. Our lifespan is affected by our genetics and our environmental exposure, and our death is usually attributable to failure HALLMARKS OF AGING 1. Genomic Instability: DNA Damage: Our DNA, the blueprint of life, faces constant assaults from free radicals, radiation, and errors during replication. These damages accumulate over time, leading to mutations, miscoding, and ultimately, cellular dysfunction. Epigenetic Alterations: Chemical modifications on DNA and histones, known as epigenetic changes, regulate gene expression. Age-related alterations in these patterns can lead to dysregulated gene expression and contribute to diverse diseases. 2. Telomere Shortening: Protective Caps: Telomeres, repetitive sequences at chromosome ends, act as protective caps. With each cell division, they shorten, eventually triggering cellular senescence (replicative arrest) or apoptosis (programmed cell death). Telomerase Activity: The enzyme telomerase can replenish telomeres, but its activity declines with age, further accelerating this process. 3. Epigenetic Dysregulation: DNA Methylation: Methylation patterns on DNA control gene expression. Age-related changes in these patterns can lead to silencing of important genes and activation of detrimental ones, impacting cellular function and contributing to disease. Histone Modifications: Alterations in histone modifications, proteins that package DNA, can also affect gene expression and contribute to age-related cellular dysfunction. 4. Protein Misfolding and Aggregation: Protein Stability: Over time, proteins can misfold or aggregate, losing their function and accumulating as harmful clumps. This can impair cellular processes and contribute to neurodegenerative diseases like Alzheimer's and Parkinson's. Chaperone Dysfunction: Chaperones are proteins that assist with proper protein folding. With age, their function can decline, leading to increased protein misfolding and aggregation. 5. Deregulated Nutrient Sensing: AMPK and Sirtuins: These signaling pathways play crucial roles in regulating metabolism and stress response. Age-related dysregulation of these pathways can lead to metabolic imbalances, inflammation, and increased susceptibility to age-related diseases. Insulin/IGF-1 Signaling: This pathway regulates growth, metabolism, and aging. With age, its sensitivity can decline, contributing to metabolic disorders and increased disease risk. 6. Mitochondrial Dysfunction: Energy Powerhouses: Mitochondria are the energy-producing organelles of the cell. Age-related damage to mitochondrial DNA and impaired function can lead to decreased energy production, increased oxidative stress, and contribute to various age-related diseases. 7. Cellular Senescence: Senescent Cells: These are cells that have permanently stopped dividing but remain metabolically active. They secrete inflammatory signals that can damage nearby cells and contribute to tissue aging and age-related diseases. Senolytic Therapies: Therapies targeting the removal of senescent cells are a promising avenue for combating age-related decline and disease The Hayflick Limit - Replicative Senescence Dr Leonard Hayflick discovered that cultured human neonatal fibroblasts will divide about 60 times before entering a non-dividing state. He noted that differentiated cells from higher animals undergo only a limited number of cell divisions (population doublings) in tissue culture, unless they become transformed or are infected with certain viruses. Cells from younger donors have greater replicative capacity, a greater number of cell divisions in cell culture, but with each generation, the number of dividing cells decreases. This limited doubling capacity is known as the Hayflick limit. The relevance of the Hayflick limit to human aging is still debated because there is a variation in the replicative capacity of some human cells, even at advanced age. Also, major tissues such as muscle and nerve, are largely post-mitotic, i.e. not actively dividing. However, changes in the metabolism and morphology of senescent cells, their responsiveness to hormones and their synthetic and degradative capacity, e.g. in the immunological and endothelial systems, may affect humans’ adaptability and susceptibility to age-related diseases, placing limits on our lifespan. Decline in Biochemical and Physiological Systems with Age. Ageing has broad-based physiological effects. It is fundamentally the result of changes in cellular structure and function, biochemistry and metabolism. The result of aging, even healthy aging, is increased susceptibility to disease and increased probability of death, which can be regarded as the end-point of aging. However, aging is not a disease. Diseases affect a fraction of the population; aging affects all of us, whether it is programmed or stochastic. Changes in Biochemistry and Physiology During Biochemical Changes Aging Physiological changes 1 Basal metabolic rate Lung expansion volume 2 Protein turnover Renal filtration capacity (glomerular) 3 Glucose tolerance Renal concentration capacity 4 Reproductive capacity Cardiovascular performance 5 Telomere shortening Musculoskeletal system 6 Oxidative phosphorylation Nerve conduction velocity 7 Endocrine and exocrine systems 8 Reproductive and immunological systems 9 Sensory systems (Vision, audition) Theories of Ageing 1. Biological theories: It treats aging as a genetically controlled event, determined by the programmed expression or repression of genetic information. Ageing and death are seen as the orchestrated end-stage of birth, growth, maturation and reproduction. Apoptosis (programmed cell death) and thymic involution are examples of genetically programmed events at the level of cells and organs. The decline in the immunological, neuroendocrine and reproductive systems may be seen, in a broader context, as evidence for action of a biological clock affecting the integrated functions of an organism. Biological theories attribute differences in lifespan to interspecies differences in genetics, but also provide an explanation for the observation that there is a genetic component to longevity within a species, e.g. in families with a history of longevity. Differences in lifespan among species are also closely correlated with the efficiency of DNA repair mechanisms. Longer-lived species have more efficient DNA repair processes. Numerous diseases of accelerated aging (progeria) also illustrate the importance of genetics and maintenance of the integrity of the genome during aging. Some biological or programmed theories of ageing include Endocrine theory: Biological clock acts through hormones to control the pace of ageing. Immunological theory: This is a programmed decline on immune functions leading to increased vulnerability to infectious diseases, ageing and death. Aging is caused in part by progressive breakdown in the immunological system. At present, evidence strongly indicates that the age-related decline in the immunological functions is mainly due to the decrease in activity of the T cells. Auto-antibodies produced by the body attack not only foreign substances but also to the natural proteins of the body. They are speculated to cause age- associated diseases such as rheumatoid arthritis, systemic lupus erythematosus, etc. As age advances, immunological system becomes less able to distinguish “self” from “non-self” and as a result, proceeds to destroy normal and desirable proteins of the body. Chemical Theories Chemical theories of ageing treat aging as a somatic process resulting from cumulative damage to biomolecules. One of the chemical theories is the error-catastrophe theory, which proposes that ageing is the result of cumulative errors in the machinery for replication, repair, transcription, and translation of genetic information. Eventually, errors in critical enzymes, such as DNA and RNA polymerases or enzymes involved in the synthesis and turnover of proteins, gradually affect the fidelity of expression of genetic information and permit the accumulation of altered proteins. The propagation of errors and resultant accumulation of dysfunctional macromolecules leads eventually to the collapse of the system. Wear and tear Theory: Through a series of experiments and mathematical models, Gompertz in the early 19 th century observed that shorter-lived mammals have a greater age-adjusted rate of death, while the death rate for poikilotherms varies with ambient temperature – flies live longer when grown at lower temperatures. This observation has been interpreted as evidence for 'rate of living' or 'wear and tear' theories of aging. Flies, being more active at higher temperature, consume more energy and die more rapidly. Flies that are restrained, e.g. in a matchbox, also live longer; wingless flies live longer; and male flies, segregated from females, also live longer. In each case, in small enclosures, without wings and in the absence of the opposite sex, male flies are less active, have lower basal metabolic rates, and have longer mean and maximum lifespans. One of the most distinguishable changes is the appearance and accumulation of lipofuscin granules in the cytoplasm of neurons, skeletal muscles, cardiac muscles and connective tissues. This pigment consists of a heterogeneous mixture of cross-linked polymerized lipids and protein formed by reactions between amino acid residues and lipid peroxidation products, such as malondialdehyde. These cross-linked products are probably derived from peroxidatively damaged cell organelles that were auto-phagocytized by lysosomes but could not be digested. It is acid insoluble and is rich in peroxidation products of polyunsaturated fatty acids and has high concentration of neutral and acidic polymerases. The cause and origin of this pigment is not clear but if large quantity of such inert substances will accumulate then it will adversely affect the metabolism and functions of the cells. Crosslinking It has been proposed that ageing is due to cross linking of macromolecules, nucleic acids and proteins, which are vital for the functioning of the cell. Several compounds like pyruvic acid, succinic acid, acetaldehyde, silicon, lead, manganese, calcium, and zinc are found in our body and are potential cross linkers. These accumulate with age and may not only cross link with two or more enzymes and inactivate them but also act at the level of genes. Increased amount of calcium is known to crosslink as it is a divalent ion and can bind with proteins and nucleic acid having negative charge. The physical plasticity of young embryonic tissues results largely from the presence of large amounts of hyaluronate and relatively small amounts of collagen that are not highly cross-linked to other related proteins. Thus, the tissues are very flexible and elastic. Many of these proteins produced during development are permanent body components; as the person ages, more and more cross-links form between these protein molecules, thereby rendering the tissues more rigid and less elastic. Somatic mutations: Ageing may also be due to accumulated random mutations, which destroys genes and causes loss of chromosomes of somatic cells like that of cardiac muscles, skeletal muscles and brain. These cells do not divide after certain stage of growth due to mutations and if they do, the genes either do not produce any protein or produce defective proteins. After reaching certain level, they may inactivate the cell its death. The number of functional somatic cells decreases with age. The organism dies when number of functional cells decreases below certain level. The Free Radical Theory of Aging The most widely accepted chemical theory of aging is the free radical theory of aging (FRTA). This theory treats aging as the result of cumulative oxidative damage to biomolecules: DNA, RNA, protein, lipids, and glycoconjugates. From the viewpoint of the FRTA, longer-lived organisms have lower rates of production of reactive oxygen species (ROS), better antioxidant defenses, and more efficient repair or turnover processes. The FRTA focuses on ROS as the primary source of damage and the fundamental cause of aging. Radicals are compounds that contain a single electron, usually in an outside orbital. Oxygen is a biradical, a molecule that has two unpaired electrons in separate orbitals. Through a number of enzymatic and nonenzymatic processes that routinely occur in cells, O2 accepts single electrons to form highly reactive oxygen species (ROS). The ROS formed by the reduction of O2 are the radical superoxide (O2−), the nonradical hydrogen peroxide (H2O2), and the hydroxyl radical (OH ). O2 is both essential for oxidation reactions in the Atoms and molecules pathways of adenosine triphosphate (ATP) generation, detoxification, and biosynthesis. However, when O2 having one unpaired accepts single electrons, it is transformed into highly electron can cross link reactive oxygen radicals that damage cellular lipids, proteins, and DNA. two molecules. These It also forms lipid peroxides and malondialdehyde compounds are produced from membrane lipids containing polyunsaturated during oxidation of fatty acids. Damage by reactive oxygen radicals contributes to organic compounds by cellular death and degeneration in a wide range of molecular oxygen as diseases. follows: In some cases, free-radical damage is the direct cause of a disease state (e.g., tissue damage initiated by RH + O2 → R. + H2O2 exposure to ionizing radiation). R– + O2 → RO2 In neurodegenerative diseases, such as Parkinson disease, or in ischemia-reperfusion injury, ROS may RO2 + RH → R + ROOH perpetuate the cellular damage caused by another process R– + R → R:R Mitochondrial Theories of Aging Mitochondria contain proteins specified by both nuclear and mitochondrial DNA. Those encoded by the mitochondrial DNA include essential subunits of the three proton pumps and ATP synthase. Mitochondria are the major site of ROS production in the cell, and the MtDNA is especially sensitive to mutations. mtDNA is not protected by a sheath of histones, and mitochondria have limited capacity for DNA repair. Mitochondrial diseases commonly involve defects of energy metabolism, including the pyruvate dehydrogenase complex, pyruvate carboxylase, complexes I, II, III, and IV, cytochrome c, ATP synthase (complex V), and biosynthesis of ubiquinone. These defects can be caused by mutations in both nuclear and mitochondrial DNA, but mtDNA suffers many more mutations than nuclear DNA. Such defects often result in the accumulation of lactic acid because of impaired oxidative phosphorylation, and may cause cell death, especially in skeletal (myopathies) and cardiac muscles (cardiomyopathies) and nerve (encephalopathies) tissues, which are heavily dependent on oxidative metabolism. A natural decline occurs in mitochondrial DNA function with age. If this decline reaches a threshold, which apparently differs from tissue to tissue, the normal mitochondrial function is lost, and the tissue or organ may exhibit disease. In a small number of people, this mitochondrial degeneration occurs very early in life, resulting in premature ageing Effects of Ageing on Some Body Tissues and Organs. Aging of Muscle - Damage to Mitochondrial DNA A general decrease in skeletal muscle mass and strength is observed in old age. This is due to a decrease in both the number of motor neurons and the number and size of myofibers. The fiber loss is accompanied by an increase in interstitial, fibrous connective tissue, and a reduction in capillary density, which limits the blood supply. The decrease in muscle mass and strength contributes to frailty and the increased risk of mortality. The loss in skeletal muscle mass may also contribute to glucose intolerance in the elderly as a result of the decreasing mass of tissue available to take up from blood. One of the major changes in muscle biochemistry with age is an increase in the number of muscle cells with mitochondria deficient in cytochrome oxidase, which limits the muscle's ability to do work. As mitochondria become less efficient in oxidizing NADH, they become more reduced, and the accumulation of partially reduced ubiquinone promotes the reduction of molecular oxygen, leading to increased superoxide production in older mitochondria. Under these conditions, when oxidative phosphorylation is impaired, cells appear to generate ATP primarily by glycolysis. NADH is oxidized extra-mitochondrially, primarily by NADH oxidases in the plasma membrane, which produce hydrogen peroxide, but no ATP. The Lens of the Eye The tissues with the highest content of collagen and other related proteins are most severely affected by the collagen cross-linking and tissue rigidity associated with aging. One of the first structures to exhibit pathological changes as a result of this increased rigidity is the lens of the eye. Seeing close objects becomes more difficult with advancing age until most middle-aged people require reading glasses. Loss of elasticity also affects other tissues, including the joints, blood vessels, kidneys, lungs, and heart, and greatly reduces their ability to function. The Arteries Gruel-like substances or compounds that contain lipids, cholesterol, calcium and other materials often get deposited in large or medium-sized arteries and become hardened. These deposits then become fibrotic and calcified, resulting in arteriosclerosis (hardening of the arteries). Arteriosclerosis interferes with normal blood flow and can result in a thrombus, which is a clot or plaque inside a vessel. A piece of the plaque, called an embolus can break loose, float through the circulation, and lodge in smaller arteries, where it may cause myocardial infarctions or strokes. Atherosclerosis affects all middle-aged and older people to some extent and may even occur in young people. People with high blood cholesterol appear to be at increased risk of developing atherosclerosis. In addition to dietary influences, this condition seems to have a heritable component, and blood tests are available to screen people for high blood cholesterol. INTERVENTIONS TO DELAY AGING Antioxidant Supplements Though there is an ongoing, vigorous research into establishing the fact that antioxidant supplements have any effect on maximum lifespan of humans or other vertebrates, it is still generally accepted that antioxidant supplements, most of which include vitamins, may improve health, particularly in those subjects with vitamin deficiencies. Thus, effects of antioxidant therapy on mean (and healthy) lifespan are not unexpected. Calorie Restriction Calorie restriction (CR) is one intervention that consistently extends maximum lifespan in a variety of species, including mammals, fish, flies, worms, and yeast. Reduction in total caloric intake appears to be the essential feature of this intervention, i.e. the beneficial, life-extending effects are observed whenever CR is applied and regardless of dietary composition, although early and prolonged intervention has more impressive effects. Genetic Models of Aging There is increasing interest in development of mammalian genetic models with increased lifespan. Some scientists are trying to translate the knowledge generated from genetically manipulating the lifespan to the treatment of human aging. Ames and Snell dwarf mice have separate, homozygous defects in growth hormone, thyroid hormone and prolactin production in the pituitary and a 40% increase in both mean and maximum lifespan, compared to long-lived progenitor strains. Their skin collagen is less crosslinked and they are less susceptible to some age-related diseases and declines in organ function, but they are infertile and have poor thermoregulation. In contrast to CR-mice, these mice are obese, although their body weight and food intake are decreased, compared to normal mice. Studies on these mice suggest that the onset of multiple, late-life functional declines and diseases may be decelerated in parallel by a few, underlying, unifying control mechanisms Apoptosis (Programmed Cell Death) Apoptosis is also known as programed cell death. It is a Greek word, meaning “falling of leaves”. The total number of cells in the body are regulated by controlling the rate of cell division and cell death. When cells are no longer needed or become a threat to the organism, they undergo a suicidal programmed cell death, or apoptosis. In normal organs, the number of cells newly produced will be equal to the number of dead cells. In those cells which are progressing to apoptosis, the process will involve a specific proteolytic cascade that will cause the cell to shrink and condense, to disassemble its cytoskeleton, and to alter its cell surface so that a neighboring phagocytic cell, such as a macrophage, can attach to the cell membrane and digest the cell. In contrast to programmed death, cells that die as a result of an acute injury usually swell and burst due to loss of cell membrane integrity, a process called cell necrosis. Necrotic cells may spill their contents, causing inflammation and injury to neighboring cells. Apoptosis, however, is an orderly cell death that results in disassembly and phagocytosis of the cell before any leakage of its contents occurs, and neighboring cells usually remain healthy. Initial Events of Apoptosis Normal Pathways to Apoptosis Apoptosis can be divided into three general phases: An initiation phase, A signal integration phase, An execution phase. At the initiation phase, apoptosis can be initiated by external signals that work through death receptors, such as tumor necrosis factor (TNF), or deprivation of growth hormones. intracellular events that affect mitochondrial integrity (e.g., oxygen deprivation, radiation), and irreparably damaged DNA. In the signal integration phase, these pro-apoptotic signals are balanced against antiapoptotic cell-survival signals by several pathways, including members of the Bcl-2 family of proteins. Apoptosis is only initiated when the signals from pro-apoptotic factors override the anti-apoptotic factors in the cell. The execution phase of apoptosis is carried out by proteolytic enzymes called caspases. Major components in apoptosis. The release of cytochrome c from mitochondria, or activation of death receptors, can both lead to the initiation of apoptosis. Normal Pathways to Apoptosis Apoptosis can be divided into three general phases: An initiation phase, A signal integration phase, An execution phase. At the initiation phase, apoptosis can be initiated by external signals that work through death receptors, such as tumor necrosis factor (TNF), or deprivation of growth hormones. The Death-Receptor Pathway to Apoptosis The Mitochondrial Integrity Pathway to Apoptosis: Progerias - Diseases of Accelerated Aging Caused by Defects in DNA Repair A number of genetic progeroid diseases are considered models of accelerated aging (progeria), result in premature aging. These monogenic diseases display many but not all, of the features of normal aging. Few progeric patients develop dementia or age-related pathologies, such as Alzheimer's disease. The progerias are sometimes described as caricatures of aging. They are useful models for understanding the ageing process. Several of them arise from deficiencies in repair of DNA. They also suggest that efficient repair of DNA is essential for prevention of cancer and for normal aging Werner's and Bloom's syndromes are autosomal recessive diseases caused by mutation of distinct DNA helicase genes which are thought to have a role in replication and repair of damaged DNA. Patients with Werner's syndrome appear normal during childhood, but stop growing in their teens. They gradually show many symptoms of premature aging, including graying and loss of hair, thinning of skin, development of early cataracts, impaired glucose tolerance and diabetes, atherosclerosis and osteoporosis, and increased rates of cancer. Death usually occurs in the mid-40s from cardiovascular disease. Fibroblasts from Werner's patients divide only about 20 times in cell culture and have higher levels of protein-bound carbonyl groups, an indicator of increased oxidative stress. Bloom's Syndrome is also characterized by increased frequency of chromosomal breaks, dwarfism, photosensitivity and increased frequency of cancer and leukemia; death occurs typically in the mid-20s. Ataxia-telangiectasia, or fragile chromosome syndrome, is associated with increased loss of telomeres with cell division and deficiency in repair of double-strand DNA breaks. It is caused by a defect in a protein kinase involved in signal transduction, cell cycle control and DNA repair. Hutchinson-Gilford syndrome is a severe, pediatric form of progeria. Patients have many of the symptoms of Werner's syndrome, but the symptoms appear at an earlier age and death usually occurs by the mid- 20s. This syndrome is caused by a defect in a gene for lamin, a component of the nuclear lamina, which together with nuclear membranes and pore complexes comprise the nuclear envelope. Hutchinson-Gilford is one of several distinct syndromes associated with lamin mutations. The progeric mutation appears to increase nuclear fragility and aberrant mRNA splicing; as in Werner's syndrome, cultured fibroblasts become prematurely senescent.