Adjuncts to Anesthesia PDF
Document Details
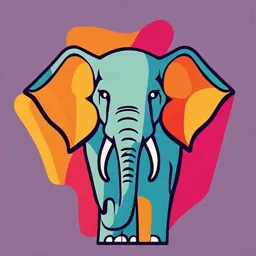
Uploaded by ImpeccableSeattle5651
Universidad de los Andes
Tags
Related
- Anesthesia for Ophthalmic Procedures PDF
- Anesthesia for Ear, Nose, Throat, and Maxillofacial Surgery Part 1 PDF
- Anesthesia for Ear, Nose, Throat, and Maxillofacial Surgery Part 2 PDF
- Anesthesia For Ear, Nose, Throat, And Maxillofacial Surgery Part 2 PDF
- Anesthesia For Ear, Nose, Throat & Maxillofacial Surgery (PDF)
- Anesthesia for Geriatrics PDF
Summary
This document provides an overview of adjunctive medications in anesthesia. It covers various drug classes, their mechanisms of action, and clinical uses. The summary highlights the importance of adjunctive agents in managing patients undergoing anesthesia or surgery. It is a professional medical document.
Full Transcript
CHAPTER 17 Adjuncts to Anesthesia KEY CONCEPTS Diphenhydramine is one of a diverse group of drugs that competitively blocks H1 receptors. Many drugs with H1-receptor antagonist properties have considerable antimuscarinic, or...
CHAPTER 17 Adjuncts to Anesthesia KEY CONCEPTS Diphenhydramine is one of a diverse group of drugs that competitively blocks H1 receptors. Many drugs with H1-receptor antagonist properties have considerable antimuscarinic, or atropine-like, activity (eg, dry mouth), or antiserotonergic activity (antiemetic). H2 blockers reduce the perioperative risk of aspiration pneumonia by decreasing gastric fluid volume and raising the pH of gastric contents. Metoclopramide increases lower esophageal sphincter tone, speeds gastric emptying, and lowers gastric fluid volume by enhancing the stimulatory effects of acetylcholine on intestinal smooth muscle. Ondansetron, granisetron, tropisetron, and dolasetron selectively block serotonin 5-HT3 receptors, with little or no effect on dopamine receptors. Located peripherally and centrally, 5-HT3 receptors appear to play an important role in the initiation of the vomiting reflex. Ketorolac is a parenteral nonsteroidal antiinflammatory drug that provides analgesia by inhibiting prostaglandin synthesis. Clonidine is a commonly used antihypertensive agent but in anesthesia it is used as an adjunct for epidural, caudal, and peripheral nerve block anesthesia and analgesia. It is often used in the management of patients with chronic neuropathic pain to increase the efficacy of epidural opioid infusions. Dexmedetomidine is a parenteral selective α2-agonist with sedative properties. It appears to be more selective for the α2-receptor than clonidine. Selective activation of carotid chemoreceptors by low doses of doxapram stimulates hypoxic drive, producing an increase in tidal volume and a slight increase in respiratory rate. Doxapram is not a specific reversal agent and should not replace standard supportive therapy (ie, mechanical ventilation). Naloxone reverses the agonist activity associated with endogenous or exogenous opioid compounds. Flumazenil is useful in the reversal of benzodiazepine sedation and the treatment of benzodiazepine overdose. Aspiration does not necessarily result in aspiration pneumonia. The seriousness of the lung damage depends on the volume and composition of the aspirate. Patients are at risk if their gastric volume is greater than 25 mL (0.4 mL/kg) and their gastric pH is less than 2.5. Many drugs are routinely administered perioperatively to protect against aspiration pneumonitis, to prevent or reduce the incidence of perianesthetic nausea and vomiting, or to reverse respiratory depression secondary to narcotics or benzodiazepines. This chapter discusses these agents along with other unique classes of drugs that are often administered as adjuvants during anesthesia or analgesia. Additionally, many nonanesthetic agents are increasingly prescribed perioperatively to provide for enhanced recovery following surgery (see Chapter 48). Aspiration Aspiration of gastric contents is a rare and potentially fatal event that can complicate anesthesia. Based on an animal study, it is often stated that aspiration of 25 mL of volume at a pH of less than 2.5 will be sufficient to produce aspiration pneumonia. Many factors place patients at risk for aspiration, including “full” stomach, intestinal obstruction, hiatal hernia, obesity, pregnancy, reflux disease, emergency surgery, and inadequate depth of anesthesia. Many approaches are employed to reduce the potential for aspiration perioperatively. Many of these interventions, such as the holding of cricoid pressure (Sellick maneuver) and rapid sequence induction, may only offer limited protection. Cricoid pressure can be applied incorrectly and fail to occlude the esophagus. Whether it has any beneficial effect on outcomes even when it is applied correctly remains unproven. Anesthetic agents can decrease lower esophageal sphincter tone and decrease or obliterate the gag reflex, theoretically increasing the risk for passive aspiration. Additionally, inadequately anesthetized patients can vomit; if the airway is unprotected, aspiration of gastric contents may occur. Different combinations of premedications have been advocated to reduce gastric volume, increase gastric pH, or augment lower esophageal sphincter tone. These agents include antihistamines, antacids, and metoclopramide. HISTAMINE-RECEPTOR ANTAGONISTS Histamine Physiology Histamine is found in the central nervous system, in the gastric mucosa, and in other peripheral tissues. It is synthesized by decarboxylation of the amino acid histidine. Histaminergic neurons are primarily located in the posterior hypothalamus but have wide projections in the brain. Histamine also normally plays a major role in the secretion of hydrochloric acid by parietal cells in the stomach (Figure 17–1). The greatest concentrations of histamine are found in the storage granules of circulating basophils and mast cells. Mast cells tend to be concentrated in connective tissue just beneath epithelial (mucosal) surfaces. Histamine release (degranulation) from these cells can be triggered by chemical, mechanical, or immunological stimulation FIGURE 17–1 Secretion of hydrochloric acid is normally mediated by gastrin- induced histamine release from enterochromaffin-like cells (ECL) in the stomach. Note that acid secretion by gastric parietal cells can also be increased indirectly by acetylcholine (AC) via stimulation of M3 receptors and directly by gastrin through an increase in intracellular Ca2+ concentration. Prostaglandin E2 (PGE2) can inhibit acid secretion by decreasing cyclic adenosine monophosphate (cAMP) activity. ATP, adenosine triphosphate; Gi, G inhibitory protein; Gs, G stimulatory protein. Multiple receptors (H1–H4) mediate the effects of histamine. The H1 receptor activates phospholipase C, whereas the H2 receptor increases intracellular cyclic adenosine monophosphate (cAMP). The H3 receptor is primarily located on histamine-secreting cells and mediates negative feedback, inhibiting the synthesis and release of additional histamine. The H4 receptors are present on hematopoietic cells, mast cells, and eosinophils and are active in allergy and inflammation. Histamine-N-methyltransferase metabolizes histamine to inactive metabolites that are excreted in the urine. A. Cardiovascular Histamine reduces arterial blood pressure but increases heart rate and myocardial contractility. H1-Receptor stimulation increases capillary permeability and enhances ventricular irritability, whereas H2-receptor stimulation increases heart rate and increases contractility. Both types of receptors mediate peripheral arteriolar dilation and some coronary vasodilation. B. Respiratory Histamine constricts bronchiolar smooth muscle via the H1 receptor. H2- Receptor stimulation may produce mild bronchodilation. Histamine has variable effects on the pulmonary vasculature; the H1 receptor appears to mediate some pulmonary vasodilation, whereas the H2 receptor may be responsible for histamine-mediated pulmonary vasoconstriction. C. Gastrointestinal Activation of H2 receptors in parietal cells increases gastric acid secretion. Stimulation of H1 receptors leads to contraction of intestinal smooth muscle. D. Dermal The classic wheal-and-flare response of the skin to histamine results from increased capillary permeability and vasodilation, primarily via H1-receptor activation. E. Immunological Histamine is a major mediator of type 1 hypersensitivity reactions. H1-Receptor stimulation attracts leukocytes and induces synthesis of prostaglandin. In contrast, the H2 receptor appears to activate suppressor T lymphocytes. 1. H1-Receptor Antagonists Mechanism of Action Diphenhydramine (an ethanolamine) is one of a diverse group of drugs that competitively blocks H1 receptors (Table 17–1). Many drugs with H1-receptor antagonist properties have considerable antimuscarinic, or atropine-like, activity (eg, dry mouth), or antiserotonergic activity (antiemetic). Promethazine is a phenothiazine derivative with H1-receptor antagonist activity as well as antidopaminergic and α-adrenergic–blocking properties. TABLE 17–1 Properties of commonly used H1-receptor antagonists.1 Clinical Uses Like other H1-receptor antagonists, diphenhydramine has a multitude of therapeutic uses: suppression of allergic reactions and symptoms of upper respiratory tract infections (eg, urticaria, rhinitis, conjunctivitis); vertigo, nausea, and vomiting (eg, motion sickness, Ménière disease); sedation; suppression of cough; and dyskinesia (eg, parkinsonism, drug-induced extrapyramidal side effects). Some of these actions are predictable from an understanding of histamine physiology, whereas others are the result of the drugs’ antimuscarinic and antiserotonergic effects (Table 17–1). Although H1 blockers prevent the bronchoconstrictive response to histamine, they are ineffective in treating bronchial asthma, which is primarily due to other mediators. Likewise, H1 blockers will not completely prevent the hypotensive effect of histamine unless an H2 blocker is administered concomitantly. Although many H1 blockers cause significant sedation, ventilatory drive is usually unaffected in the absence of other sedative medications. Promethazine and hydroxyzine were often combined with opioids to potentiate analgesia. Newer (second-generation) antihistamines tend to produce little or no sedation because of limited penetration across the blood–brain barrier. This group of drugs is used primarily for allergic rhinitis and urticaria. They include loratadine, fexofenadine, and cetirizine. Many preparations for allergic rhinitis often also contain vasoconstrictors such as pseudoephedrine. Meclizine and dimenhydrinate are used primarily as an antiemetic, particularly for motion sickness, and in the management of vertigo. Cyproheptadine, which also has significant serotonin antagonist activity, has been used in the management of Cushing disease, carcinoid syndrome, and vascular (cluster) headaches. Dosage The usual adult dose of diphenhydramine is 25 to 50 mg (0.5–1.5 mg/kg) orally, intramuscularly, or intravenously every 3 to 6 h. The doses of other H1-receptor antagonists are listed in Table 17–1. Drug Interactions The sedative effects of H1-receptor antagonists can potentiate other central nervous system depressants such as barbiturates, benzodiazepines, and opioids. 2. H2-Receptor Antagonists Mechanism of Action H2-Receptor antagonists include cimetidine, famotidine, nizatidine, and ranitidine (Table 17–2). These agents competitively inhibit histamine binding to H2 receptors, thereby reducing gastric acid output and raising gastric pH. TABLE 17–2 Pharmacology of aspiration pneumonia prophylaxis.1 Clinical Uses All H2-receptor antagonists are equally effective in the treatment of peptic duodenal and gastric ulcers, hypersecretory states (Zollinger–Ellison syndrome), and gastroesophageal reflux disease (GERD). Intravenous preparations are also used to prevent stress ulceration in critically ill patients. Duodenal and gastric ulcers are usually associated with Helicobacter pylori infection, which is treated with combinations of bismuth, tetracycline, and metronidazole. By decreasing gastric fluid volume and hydrogen ion content, H2 blockers reduce the perioperative risk of aspiration pneumonia. These drugs affect the pH of only those gastric secretions that occur after their administration. The combination of H1- and H2-receptor antagonists provides some protection against drug-induced allergic reactions (eg, intravenous radiocontrast, chymopapain injection for lumbar disk disease, protamine, vital blue dyes used for sentinel node biopsy). Although pretreatment with these agents does not reduce histamine release, it may decrease subsequent hypotension. Side Effects Rapid intravenous injection of cimetidine or ranitidine has been rarely associated with hypotension, bradycardia, arrhythmias, and cardiac arrest. H2-Receptor antagonists change the gastric flora by virtue of their pH effects. Complications of long-term cimetidine therapy include hepatotoxicity (elevated serum transaminases), interstitial nephritis (elevated serum creatinine), granulocytopenia, and thrombocytopenia. Cimetidine also binds to androgen receptors, occasionally causing gynecomastia and impotence. Finally, cimetidine has been associated with changes in mental status ranging from lethargy and hallucinations to seizures, particularly in elderly patients. In contrast, ranitidine, nizatidine, and famotidine do not affect androgen receptors and penetrate the blood–brain barrier poorly. Dosage As a premedication to reduce the risk of aspiration pneumonia, H2-receptor antagonists should be administered at bedtime and again at least 2 h before surgery. Because all four drugs are eliminated primarily by the kidneys, the dose should be reduced in patients with significant renal dysfunction. Drug Interactions Cimetidine may reduce hepatic blood flow and binds to the cytochrome P-450 mixed-function oxidases. These effects slow the metabolism of a multitude of drugs, including lidocaine, propranolol, diazepam, theophylline, phenobarbital, warfarin, and phenytoin. Ranitidine is a weak inhibitor of the cytochrome P-450 system, and no significant drug interactions have been demonstrated. Famotidine and nizatidine do not appear to affect the cytochrome P-450 system. ANTACIDS Mechanism of Action Antacids neutralize the acidity of gastric fluid by providing a base (usually hydroxide, carbonate, bicarbonate, citrate, or trisilicate) that reacts with hydrogen ions to form water. Clinical Uses Common uses of antacids include the treatment of peptic ulcers and GERD. In anesthesiology, antacids provide protection against the harmful effects of aspiration pneumonia by raising the pH of gastric contents. Unlike H2-receptor antagonists, antacids have an immediate effect. Unfortunately, they increase intragastric volume. Aspiration of particulate antacids (aluminum or magnesium hydroxide) produces abnormalities in lung function comparable to those that occur following acid aspiration. Nonparticulate antacids (sodium citrate or sodium bicarbonate) are much less damaging to lung alveoli if aspirated. Furthermore, nonparticulate antacids mix with gastric contents better than particulate solutions. Timing is critical, as nonparticulate antacids lose their effectiveness 30 to 60 min after ingestion. Dosage The usual adult dose of a 0.3 M solution of sodium citrate—Bicitra (sodium citrate and citric acid) or Polycitra (sodium citrate, potassium citrate, and citric acid)—is 15 to 30 mL orally, 15 to 30 min prior to induction (Table 17–2). Drug Interactions Because antacids alter gastric and urinary pH, they change the absorption and elimination of many drugs. The rate of absorption of digoxin, cimetidine, and ranitidine is slowed, whereas the rate of phenobarbital elimination is quickened. METOCLOPRAMIDE Mechanism of Action Metoclopramide acts peripherally as a cholinomimetic (ie, facilitates acetylcholine transmission at selective muscarinic receptors) and centrally as a dopamine receptor antagonist. Its action as a prokinetic agent in the upper gastrointestinal (GI) tract is not dependent on vagal innervation but is abolished by anticholinergic agents. It does not stimulate secretions. Clinical Uses By enhancing the stimulatory effects of acetylcholine on intestinal smooth muscle, metoclopramide increases lower esophageal sphincter tone, speeds gastric emptying, and lowers gastric fluid volume (Table 17–2). These properties account for its efficacy in the treatment of patients with diabetic gastroparesis and GERD, as well as prophylaxis for those at risk for aspiration pneumonia. Metoclopramide does not affect the secretion of gastric acid or the pH of gastric fluid. Metoclopramide produces an antiemetic effect by blocking dopamine receptors in the chemoreceptor trigger zone of the central nervous system. However, at doses used clinically during the perioperative period, the drug’s ability to reduce postoperative nausea and vomiting is negligible. Side Effects Rapid intravenous injection may cause abdominal cramping, and metoclopramide is contraindicated in patients with complete intestinal obstruction. It can induce a hypertensive crisis in patients with pheochromocytoma by releasing catecholamines from the tumor. Sedation, nervousness, and extrapyramidal signs from dopamine antagonism (eg, akathisia) are uncommon and reversible. Nonetheless, metoclopramide is best avoided in patients with Parkinson disease. Prolonged treatment with metoclopramide can lead to tardive dyskinesia. Metoclopramide-induced increases in aldosterone and prolactin secretion are probably inconsequential during short-term therapy. Metoclopramide may rarely result in hypotension and arrhythmias. Dosage An adult dose of 10 to 15 mg of metoclopramide (0.25 mg/kg) is effective orally, intramuscularly, or intravenously (injected over 5 min). Larger doses (1–2 mg/kg) have been used to prevent emesis during chemotherapy. The onset of action is much more rapid following parenteral (3–5 min) than oral (30–60 min) administration. Because metoclopramide is excreted in the urine, its dose should be decreased in patients with kidney dysfunction. Drug Interactions Antimuscarinic drugs (eg, atropine, glycopyrrolate) block the GI effects of metoclopramide. Metoclopramide decreases the absorption of orally administered cimetidine. Concurrent use of phenothiazines or butyrophenones (droperidol) increases the likelihood of extrapyramidal side effects. PROTON PUMP INHIBITORS Mechanism of Action These agents, including omeprazole (Prilosec), lansoprazole (Prevacid), rabeprazole (AcipHex), esomeprazole (Nexium), and pantoprazole (Protonix), bind to the proton pump of parietal cells in the gastric mucosa and inhibit secretion of hydrogen ions. Clinical Uses Proton pump inhibitors (PPIs) are indicated for the treatment of peptic ulcer, GERD, and Zollinger–Ellison syndrome. They may promote healing of peptic ulcers and erosive GERD more quickly than H2-receptor blockers. There are ongoing questions regarding the safety of PPIs in patients taking clopidogrel (Plavix). These concerns relate to inadequate antiplatelet therapy when these drugs are combined due to inadequate activation of clopidogrel by hepatic enzyme CYP2C19, which is inhibited to varying degrees by PPIs. Side Effects PPIs are generally well tolerated, causing few side effects. Adverse side effects primarily involve the GI system (nausea, abdominal pain, constipation, diarrhea). On rare occasions, these drugs have been associated with myalgias, anaphylaxis, angioedema, and severe dermatological reactions. Long-term use of PPIs has also been associated with gastric enterochromaffin-like cell hyperplasia and an increased risk of pneumonia secondary to bacterial colonization in the higher-pH environment. Dosage Recommended oral doses for adults are omeprazole, 20 mg; lansoprazole, 15 mg; rabeprazole, 20 mg; and pantoprazole, 40 mg. Because these drugs are primarily eliminated by the liver, repeat doses should be decreased in patients with severe liver impairment. Drug Interactions PPIs can interfere with hepatic P-450 enzymes, potentially decreasing the clearance of diazepam, warfarin, and phenytoin. Concurrent administration can decrease clopidogrel (Plavix) effectiveness, as the latter medication is dependent on hepatic enzymes for activation. Postoperative Nausea & Vomiting (PONV) Without any prophylaxis, PONV occurs in approximately 30% or more of the general surgical population and up to 70% to 80% in patients with predisposing risk factors. The Society for Ambulatory Anesthesia (SAMBA) provides extensive guidelines for the management of PONV. Table 17–3 identifies risks factors for PONV and scores the evidence for assessing risk. When PONV risk is sufficiently great, prophylactic antiemetic medications are administered and strategies to reduce its incidence are initiated. The Apfel score provides a simplified assessment tool to predict risk of PONV (Figures 17–2 and 17–3). (Obesity, anxiety, and reversal of neuromuscular blockade are not independent risk factors for PONV.) TABLE 17–3 Risk factors for PONV.1-3 FIGURE 17–2 Risk score for PONV in adults. Simplified risk score from Apfel et al to predict the patient’s risk for PONV. When 0, 1, 2, 3, and 4 of the risk factors are present, the corresponding risk for PONV is about 10%, 20%, 40%, 60%, and 80%, respectively. PONV, postoperative nausea and vomiting. (Reproduced with permission from Gan TJ, Diemunsch P, Habib A, et al. Consensus guidelines for the management of postoperative nausea and vomiting. Anesth Analg. 2014 Jan;118(1):85-113.) FIGURE 17–3 Simplified risk score for POV in children. Simplified risk score from Eberhart et al to predict the risk for POV in children. When 0, 1, 2, 3, or 4 of the depicted independent predictors are present, the corresponding risk for PONV is approximately 10%, 10%, 30%, 50%, or 70%, respectively. POV, postoperative vomiting; PONV, postoperative nausea and vomiting. (Reproduced with permission from Gan TJ, Diemunsch P, Habib A, et al. Consensus guidelines for the management of postoperative nausea and vomiting. Anesth Analg. 2014 Jan;118(1):85-113.) Drugs used in the prophylaxis and treatment of PONV include 5-HT3 antagonists, butyrophenones, dexamethasone, neurokinin-1 receptor antagonists (aprepitant); antihistamines and transdermal scopolamine may also be used. At- risk patients often benefit from one or more prophylactic measures. Because all drugs have adverse effects, the SAMBA algorithm can be used to help guide PONV prophylaxis and therapy (Figure 17–4). FIGURE 17–4 Algorithm for management of postoperative nausea and vomiting. PACU, postanesthesia care unit; PONV, postoperative nausea and vomiting; POV, postoperative vomiting. (Reproduced with permission from Gan TJ, Diemunsch P, Habib A, et al. Consensus guidelines for the management of postoperative nausea and vomiting. Anesth Analg. 2014 Jan;118(1):85-113.) 5-HT3 RECEPTOR ANTAGONISTS Serotonin Physiology Serotonin, 5-hydroxytryptamine (5-HT), is present in large quantities in platelets and the GI tract (enterochromaffin cells and the myenteric plexus). It is also an important neurotransmitter in multiple areas of the central nervous system. Serotonin is formed by hydroxylation and decarboxylation of tryptophan. Monoamine oxidase inactivates serotonin into 5-hydroxyindoleacetic acid (5- HIAA). The physiology of serotonin is very complex because there are at least seven receptor types, most with multiple subtypes. The 5-HT3 receptor mediates vomiting and is found in the GI tract and the brain (area postrema). The 5-HT2A receptors are responsible for smooth muscle contraction and platelet aggregation, the 5-HT4 receptors in the GI tract mediate secretion and peristalsis, and the 5- HT6 and 5-HT7 receptors are located primarily in the limbic system where they appear to play a role in depression. All except the 5-HT3 receptor are coupled to G proteins and affect either adenylyl cyclase or phospholipase C; effects of the 5-HT3 receptor are mediated via an ion channel. A. Cardiovascular Except in the heart and skeletal muscle, serotonin is a powerful vasoconstrictor of arterioles and veins. Its vasodilator effect in the heart is endothelium dependent. When the myocardial endothelium is damaged following injury, serotonin produces vasoconstriction. The pulmonary and kidney vasculatures are very sensitive to the arterial vasoconstrictive effects of serotonin. Modest and transient increases in cardiac contractility and heart rate may occur immediately following serotonin release; reflex bradycardia often follows. Vasodilation in skeletal muscle can subsequently cause hypotension. Excessive serotonin can produce serotonin syndrome, characterized by hypertension, hyperthermia, and agitation. B. Respiratory Contraction of smooth muscle increases airway resistance. Bronchoconstriction from released serotonin is often a prominent feature of carcinoid syndrome C. Gastrointestinal Direct smooth muscle contraction (via 5-HT2 receptors) and serotonin-induced release of acetylcholine in the myenteric plexus (via 5-HT3 receptors) greatly augment peristalsis. Secretions are unaffected. D. Hematological Activation of 5-HT2 receptors causes platelet aggregation. Mechanism of Action Ondansetron, granisetron, tropisetron, and dolasetron selectively block serotonin 5-HT3 receptors, with little or no effect on dopamine receptors (5-HT3 receptors, which are located peripherally (abdominal vagal afferents) and centrally (chemoreceptor trigger zone of the area postrema and the nucleus tractus solitarius), appear to play an important role in the initiation of the vomiting reflex. The 5-HT3 receptors of the chemoreceptor trigger zone in the area postrema reside outside the blood–brain barrier (Figure 17–5). The chemoreceptor trigger zone is activated by substances such as anesthetics and opioids and signals the nucleus tractus solitarius, resulting in PONV. Emetogenic stimuli from the GI tract similarly stimulate the development of PONV. FIGURE 17–5 Neurological pathways involved in pathogenesis of nausea and vomiting (see text). (Reproduced with permission from Krakauer EL, Zhu AX, Bounds BC, et al. Case records of the Massachusetts General Hospital. Weekly clinicopathological exercises. Case 6-2005. A 58-year-old man with esophageal cancer and nausea, vomiting, and intractable hiccups, N Engl J Med. 2005 Feb 24;352(8):817-825.) Clinical Uses 5-HT3-Receptor antagonists are generally administered at the end of surgery. All these agents are effective antiemetics in the postoperative period. A new agent, palonosetron has an extended duration of action and may reduce the incidence of postdischarge nausea and vomiting (PDNV). SAMBA guidelines suggest risk factors for PDNV include: Female sex History of PONV Age 50 years or younger Use of opioids in the postanesthesia care unit (PACU) Nausea in the PACU Side Effects 5-HT3-Receptor antagonists are essentially devoid of serious side effects, even in amounts several times the recommended dose. They do not appear to cause sedation, extrapyramidal signs, or respiratory depression. The most commonly reported side effect is headache. All three drugs can slightly prolong the QT interval on the electrocardiogram. This effect may be more frequent with dolasetron (no longer available in the United States). Nonetheless, these drugs, should be used cautiously in patients who are taking antiarrhythmic drugs or who have a prolonged QT interval. Ondansetron undergoes extensive metabolism in the liver via hydroxylation and conjugation by cytochrome P-450 enzymes. Liver failure impairs clearance several-fold, and the dose should be reduced accordingly. BUTYROPHENONES Droperidol (0.625–1.25 mg) was previously used routinely for PONV prophylaxis. Given at the end of the procedure, it blocks dopamine receptors that contribute to the development of PONV. Despite its effectiveness, many practitioners no longer routinely administer this medication because of a U.S. Food and Drug Administration (FDA) black box warning related to concerns that doses described in the product labeling (“package insert”) may lead to QT prolongation and development of torsades des pointes arrhythmia. However, the doses relevant to the FDA warning, as acknowledged by the FDA, were those used for neurolept anesthesia (5–15 mg), not the much smaller doses employed for PONV. Cardiac monitoring is warranted when large doses of the drug are used. There is no evidence that use of droperidol at the doses routinely employed for PONV management increases the risk of sudden cardiac death in the perioperative population. As with other drugs that antagonize dopamine, droperidol use in patients with Parkinson disease and in other patients manifesting extrapyramidal signs should be carefully considered. The phenothiazine, prochlorperazine (Compazine), which affects multiple receptors (histaminergic, dopaminergic, muscarinic), may be used for PONV management. It may cause extrapyramidal and anticholinergic side effects. Promethazine (Phenergan) works primarily as an anticholinergic agent and antihistamine and likewise can be used to treat PONV. As with other agents of this class, anticholinergic effects (sedation, delirium, confusion, vision changes) can complicate the postoperative period. DEXAMETHASONE Dexamethasone (Decadron) in doses as small as 4 mg has been shown to be as effective as ondansetron in reducing the incidence of PONV. Dexamethasone should be given at induction as opposed to the end of surgery, and its mechanism of action is unclear. It may provide analgesic and mild euphoric effects. Dexamethasone can increase postoperative blood glucose concentration, and some practitioners have suggested that dexamethasone could increase the risk of postoperative infection. Nonetheless, most studies have not demonstrated any increase in wound infections following dexamethasone administration for PONV prophylaxis. NEUROKININ-1 RECEPTOR ANTAGONIST Substance P is a neuropeptide that interacts at neurokinin-1 (NK1) receptors. NK1 antagonists inhibit substance P at central and peripheral receptors. Aprepitant, an NK1 antagonist, has been found to reduce PONV perioperatively and is additive with ondansetron for this indication. OTHER PONV STRATEGIES Several other agents and techniques have been employed to reduce the incidence of PONV. Transdermal scopolamine has been used effectively, although it may produce central anticholinergic effects (confusion, blurred vision, and dry mouth). Acupuncture, acupressure, and transcutaneous electrical stimulation of the P6 acupuncture point can reduce PONV incidence and medication requirements. As no single agent will both treat and prevent PONV, perioperative management centers on identifying patients at greatest risk so that prophylaxis, often with multiple agents, may be initiated. Since systemic opioid administration is associated with PONV, opioid-sparing strategies (eg, use of regional anesthetics and nonopioid analgesics) can markedly reduce the risk of PONV. Other Drugs Used as Adjuvants to Anesthesia KETOROLAC Mechanism of Action Ketorolac is a parenteral nonsteroidal antiinflammatory drug (NSAID) that provides analgesia by inhibiting prostaglandin synthesis. A peripherally acting drug, it has become a popular alternative to opioids for postoperative analgesia because of its minimal central nervous system side effects. Clinical Uses Ketorolac is indicated for the short-term (50 kg weight) dose of 1 g is infused to a maximum total dose of 4 g/d. Patients weighing 50 kg or less should receive a maximal dose of 15 mg/kg and a maximal total dose of 75 mg/kg/d. Hepatoxicity is a known risk of overdosage, and the drug should be used with caution in patients with hepatic disease or undergoing hepatic surgery. Oral and rectal acetaminophen are as effective as the intravenous form and orders of magnitude less expensive. CLONIDINE Mechanism of Action Clonidine is an imidazoline derivative with predominantly α2-adrenergic agonist activity. It is highly lipid soluble and readily penetrates the blood–brain barrier and the placenta. Studies indicate that binding of clonidine to receptors is highest in the rostral ventrolateral medulla in the brainstem (the final common pathway for sympathetic outflow), where it activates inhibitory neurons. The overall effect is to decrease sympathetic activity, enhance parasympathetic tone, and reduce circulating catecholamines. There is also evidence that some of clonidine’s antihypertensive action may occur via binding to a nonadrenergic (imidazoline) receptor. In contrast, its analgesic effects, particularly in the spinal cord, are mediated entirely via pre- and possibly postsynaptic α2-adrenergic receptors that block nociceptive transmission. Clonidine also has local anesthetic effects when applied to peripheral nerves and is frequently added to local anesthetic solutions to increase duration of action. Clinical Uses Clonidine is a commonly used antihypertensive agent that reduces sympathetic tone, decreasing systemic vascular resistance, heart rate, and blood pressure. In anesthesia, clonidine is used as an adjunct for epidural, caudal, and peripheral nerve block anesthesia and analgesia. It is often used in the management of patients with chronic neuropathic pain to increase the efficacy of epidural opioid infusions. When given epidurally, the analgesic effect of clonidine is segmental, being localized to the level at which it is injected or infused. When added to local anesthetics of intermediate duration (eg, mepivacaine or lidocaine) administered for epidural or peripheral nerve block, clonidine will markedly prolong both the anesthetic and analgesic effects. Unlabeled/investigational uses of clonidine include serving as an adjunct in premedication, control of withdrawal syndromes (nicotine, opioids, alcohol, and vasomotor symptoms of menopause), and treatment of glaucoma as well as various psychiatric disorders. Side Effects Sedation, dizziness, bradycardia, and dry mouth are common side effects. Less commonly, orthostatic hypotension, nausea, and diarrhea may be observed. Abrupt discontinuation of clonidine following long-term administration (>1 month) can produce a withdrawal phenomenon characterized by rebound hypertension, agitation, and sympathetic overactivity. Dosage Epidural clonidine is usually started at 30 mcg/h in a mixture with an opioid or a local anesthetic. Oral clonidine is readily absorbed, has a 30 to 60 min onset, and lasts 6 to 12 h. In the initial treatment of hypertension, 0.1 mg can be given two times a day and adjusted until the blood pressure is controlled. The maintenance dose typically ranges from 0.1 to 0.3 mg twice daily. Transdermal preparations of clonidine can also be used for maintenance therapy. They are available as 0.1, 0.2, and 0.3 mg/d patches that are replaced every 7 days. Clonidine is metabolized by the liver and excreted by the kidney. Dosages should be reduced for patients with kidney disease. Drug Interactions Clonidine enhances and prolongs sensory and motor blockade from local anesthetics. Additive effects with hypnotic agents, general anesthetics, and sedatives can potentiate sedation, hypotension, and bradycardia. The drug should be used cautiously, if at all, in patients who take β-adrenergic blockers and in those with significant cardiac conduction system abnormalities. Lastly, clonidine can mask the symptoms of hypoglycemia in diabetic patients. DEXMEDETOMIDINE Mechanism of Action Dexmedetomidine is a parenteral selective α2 agonist with sedative properties. It appears to be more selective for the α2 receptor than clonidine. At higher doses, it loses its selectivity and also stimulates α1-adrenergic receptors. Clinical Uses Dexmedetomidine causes dose-dependent sedation, anxiolysis, some analgesia, and blunting of the sympathetic response to surgery and to other stress. Most importantly, it has an opioid-sparing effect and does not significantly depress respiratory drive; excessive sedation, however, may cause airway obstruction. The drug can be used for short-term (