Metal Additive Manufacturing - MEC454 PDF
Document Details
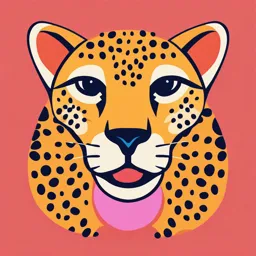
Uploaded by ReverentBowenite2086
2024
Prof Kamran Mumtaz
Tags
Summary
This document covers metal additive manufacturing, specifically laser powder bed fusion (LPBF). It discusses process control, melt pool dynamics, heat flow, defects, residual stresses, and support structures, with examples such as LPBF and EBM.
Full Transcript
Additive Manufacturing – MEC454 Metal Additive Manufacturing – Laser Powder Bed Advanced Topics November 25th-26th 2024 Prof Kamran Mumtaz Overview 1) Brief Metals Recap 2) Melt Pool Dynamics, Heat Flow & Defects 3) Residual Stresses & Support Structur...
Additive Manufacturing – MEC454 Metal Additive Manufacturing – Laser Powder Bed Advanced Topics November 25th-26th 2024 Prof Kamran Mumtaz Overview 1) Brief Metals Recap 2) Melt Pool Dynamics, Heat Flow & Defects 3) Residual Stresses & Support Structures 4) Microstructural Customisation 5) Multi-material 6) Productivity increase Part 1 of 6 Brief Metals Recap Metal AM Techniques Powder Bed Fusion Powder/Wire Feed Binder Jetting Sheet Lamination Other Powder Bed Fusion Most widely and extensively used AM process for the production of metal parts Uses thermal energy, typically laser or electron beam Can produce high density fully functional parts in one step Excellent mechanical properties Good material variety and part properties PBF – Post-processing Parts may require following operations for complete parts Removal of excess powder – tapping, compressed air, ultrasonic Thermal processing – relieve stress of or improve mechanical properties. Furnace cycles or HIPPING to reduce pores and heal micro-cracks Support removal – Wire EDM or band saw to cut parts off platform. Often hand finishing (with pliers) to pull off remaining supports Surface finishing operations - machining, shot-peening, tumbling and hand benching, electro-polishing, abrasive flow machining (for internal cavities). Micro-machining, chemical reaction at surface of material driven by fluid flow Process Control Control of material, process parameters and environment conditions have major influence on final properties of part, e.g. microstructure, density, surface roughness etc. Thermal Energy Power Spot Size etc. Scanning Environment Speed COMPONENT Inert gas/vacuum Hatch distance Pressure etc. Exposure time/dwell etc. Powder Bed Powder Morphology Powder particle size distribution Layer thickness Substrate type Pre-heat etc. Part 2 of 6 Melt Pool Dynamics, Heat Flow, Defects LPBF - Lasers Laser Based Systems Materials are melted by ABSORBING laser energy Typically fibre laser used Lasers are versatile, accurate and have a high energy density LPBF – Heat Transfer Absorbance Portion of laser energy, reflected, absorbed and transmitted (generally negligible) Materials with high reflectance (i.e Aluminium, copper etc.) difficult to process Materials reflectance also determined by surface roughness and oxides As the temperature of a material increases its absorbance also increases LPBF – Thermal Conductivity & Melt Energy Heat Flow Absorbed energy transformed into heat and flows via conduction. Thermal conductivity of powders depends on number of contacts between particles High thermal conductivity of Aluminium/Copper can make it difficult to process. Heat flows rapidly creating large melt pools, difficult to control part resolution LPBF – Energy Loss Heat will be lost after a melt pool has formed. Magnesium difficult to process due to close melt and vaporisation temperatures LPBF – Melt pool Complex, lots going on, lots can and will go wrong LPBF – Thermo capillary Flows Marangoni Convection and Melt Pool Topology Non uniform heating creates surface tension variations that induces fluid motion known as Marangoni convection. Forces are larger than natural convection and gravity and tend to dominate flow. Surface tension decreases with increasing temperature in pure liquid metals LPBF – Melt Pool Dynamics Fluid behaviour - wetting Wettability determines spreading behaviour of liquid on solid. Affecting interlayer connection, porosity and strength. Surface free energy at Liquid Vapour, Solid Vapour, Solid Liquid interfaces. If LV smaller than SV-SL, liquid will wet Liquid metals exhibit poor wetting on a solid with an oxide film causing higher contact angles increasing liquids tendency to undergo balling (discussed later) Capillary instabilities Fluid behaviour - balling Breakup of liquid into smaller entities in an effort to reduce surface tension Surface tension is a function of temperature and due to non uniform heat distribution along the melt pool surface tension variation induces To reduce instability melt pool Length to Diameter ratio should be as small as possible Melt Pool Ejection and Recoil Pressures Material can exceed its vaporisation temperature transforming it into a gas. Laser interacts with vapour causing it to ionise and create a plasma plume. Shock wave generated applying recoil pressure on melt pool Oxidation Oxidation is the combining of an element with Oxygen. A metal’s surface is continually oxidising when exposed to air. Powders have a large surface area, Aluminium forms a very thick oxide layer making it difficult to process. Laser has to break oxides before melting initiates Oxidation potential increases with temperature. Processing must take place within an inert environment LPBF – Melt pool formation X-ray imagery of melt pool formation LPBF – influence of gas flow X-ray imagery of melt pool formation LPBF – Part Properties High Density Complete melting in single step, parts produced to full density  Can match or exceed properties of cast and approaches that of wrought  Less than full density compromises fracture toughness and fatigue properties. Could lead to premature failure, act as crack initiation sites when subjected to cyclic stress  Compliance to specifications for toughness and Z fatigue properties critical in aerospace industry and Axis 0.5mm orthopaedic and dental implants X Steel Alloy (316L) LPBF – Porosity and Cracks Types of Porosity  Lack of fusion porosity (irregular shaped pores) Corrective measure - Overcome with correct energy density  Gas occluded porosity (spherically shaped pores Corrective measure - Allow more time for gas to escape  Pores within powder (typically spherically shaped pores) Corrective measure - Improve quality of powder o Solidification cracking – Rapid solidification, thermal gradients, varying expansion/contraction of material leading to crack formation. Corrective measure, reduce thermal gradient, modify material composition to increase lattice stress Part 3 of 6 Residual Stresses and Support Structures Stresses Stresses Stresses Stresses Stresses 150x150x45mm Stresses Stresses Stresses Stresses Stresses Stresses Stresses Stresses Stresses Stresses Stresses Stresses Stresses Stresses Support Structures Some parts require support/anchors due to thermal warpage Rapid heating/cooling Large thermal variations Stresses/warpage Limits geometric freedom, Incurs post processing and cost for anchor removal Cannot easily stack parts on top of each other (as with polymer SLS) 10mm M2 Why are supports required Warpage 10mm 10mm Metal Anchors/Supports 44 Slide 44 M2 Removes the requirement for anchors/supports within the Selective Laser Process (SLM) Improving SLM design freedom Reduces time/cost involved in removing supports Potential to increase SLM productivity Master, 19/07/2011 Test Part Design Overhang length 1mm-15mm Thickness 0.5mm-3mm z x Warp Measurement Data points Warp Height Warp Length L EBM Overhang Ti6Al4V 0.5mm thickness 1mm thickness 2mm thickness 3mm thickness EBM Effect of Thickness LPBF Overhang AlSi12 0.5mm thickness 1mm thickness 2mm thickness 3mm thickness 140ºC Bed Temperature 0.5mm thickness 1mm thickness 2mm thickness 3mm thickness z x 25ºC Bed Temperature 50 How to eliminate supports? In Conventional processes- Reducing thermal gradient in powder bed. Better understanding of processing parameters to reduce residual stress. A Novel Method- Anchorless Metal Additive Manufacturing!! 51 AnchorlessMetalAdditive Manufacturing Metal Eutectics Anchorless Metal AM Conventional metal AM* * Heated powder bed Anchorless Selective Laser Melting - Certain combinations of metals when Material B melt point alloyed Material A melt point can form a eutectic alloy/system (e.g Material A & B) Liquid - An alloy forming a eutectic alloy/system has a melting point lower than one or more of the individual metals melting point. Temperature Eutectic melting point - A lower melting point will allow processed Specific alloyed compositions of A & B material to remain in a will solidify at lower temperature liquid/mushy state similar to that seen during processing of polymers if adequate pre- heating is applied Solid A Material Wt % B 52 ASLM 1 2 3 Heated powder bed, mix of Laser melts metal A & B Bed temperature held un-alloyed powders forming a new eutectic (or at temperature T. Processed A&B hypo/hyper eutectic) alloy material cools but does not fully solidify Alloy of metal A & B formed with freezing Powder A & B point below T Formed alloy remain solid Still semi-liquid Will not warp No need for anchors Bed temp T Bed temp T Bed temp T Z (mix of metal A & (mix of metal A & (mix of metal A & metal B) metal B) metal B) X 53 Anchorless Selective Laser Melting Bi melts at 271°C Zn melts at 420°C Forms eutectic at Liquid Bi 97%Wt. & Zn 3%Wt. Liquid + Eutectic solidification Eutectic Liquid temperature 254.5°C composition Zn 420°C Liquid + Zn Bi 271°C 254°C Solid 54 Zinc melts 420°C HEATING PHASE Bismuth melts 270°C 55 DSC trace of feedstock Bi and Zn powder DSC solidification temperature 10-15°C less than actual eutectic (254°C) solidification Eutectic alloy formed temperature. Result of solidification temperature undercooling and DSC thermal approximately 240°C lag COOLING PHASE Zinc melts 420°C Bismuth melts 270°C 10 Anchorless Selective Laser Melting 10mm Failed stainless steel component Successful part built using ASLM built with SLM & without (Bi3Zn) anchors/supports 11 Anchorless Selective Laser Melting x50 100µm layers Eutectic Bi3Zn Zinc Steel 40mm Success Failed within 10 layers  Large flat geometries notoriously difficult to Failed within 10 layers build without Anchors  Eutectic material composition All built with 250°C pre-heating allowed geometry to be built without supports 58 Anchorless Selective Laser Melting 59 Anchorless Selective Laser Melting 60 Anchorless Selective Laser Melting 61 Anchorless Selective Laser Melting Eutectic Structure Presence of BiZn Presence of Bi only 300um 62 Anchorless Selective Laser Melting Atomic Percent Silicon 1414 ºC Al melts at 660°C Temperature, ºC Liquid Si melts at 1414°C Forms eutectic at Al 88%Wt. & Si 12%Wt. 660.45ºC 577 ºC Eutectic solidification 12% Si temperature 577°C Al Weight % Silicon Si Eutectic Hypo Hyper * Source: ASM Handbook Anchorless Selective Laser Melting Part 4 of 6 Microstructural customisation SLM - Custom microstructure Fine Microstructure Rapid melting and cooling of thin layers of material Uniform microstructure Process parameters will effect resultant microstructure Z Axis 25um X Etched CoCr Alloy SLM – Custom microstructure Stress reduction and custom microstructure Residual stress reduction using heat jackets - Studying effects of heat jackets on residual stress - It can reduce stress but could strategic placement also modify mechanical performance? Heat jacket Stress reduction and custom microstructure - No apparent microstructural changes for Ti64 SLM components Stress reduction and custom microstructure - HJ improved ductility, increasing elongation to failure from 1.09% to 2,39% - HJ UTS improved by ~100Mpa due to work hardening capacity afforded by ductility of samples Stress reduction and custom microstructure Residual stress/microstructural modification – optical pre-heat High temperature location specific powder bed pre-heat Fibre laser optics Pyrometer 40x40mm pre-heat area within Renishaw 250 system Part 5 of 6 Multi-material Multi-material Z Z X X Deposition of different materials within the powder bed. Creating unique component properties Part 6 of 6 Productivity Increase Productivity How can we increase productivity in laser powder bed fusion? Productivity How can we increase productivity in laser powder bed fusion? Diode-based additive manufacturing of metals using an optically- addressable light valve by Lawrence Livermore National Labs USA Productivity Need for speed – solution more lasers Challenges associate with increasing number of lasers using current sources More scalable processing methodology required Productivity Diode bar Diode laser technology Generally lower power output Relatively cheap Lower powering/cooling requirement Highly scalable 10mm Wavelength tuneable Focusing on 450-808nm wavelength range Productivity Diode Area Melting (DAM) method Productivity Diode Area Melting v1 Productivity High temperatures achieved Productivity Beam total Beam total P1 dimensions P1 dimensions LBP1 4.75 x 0.25 mm LBP3 9.4 x 0.4mm V 1 mm/s 3 mm/s 5 mm/s V 1 mm/s 3 mm/s 5 mm/s P P Insuf icient Insuf icient 30 W 30 W bonding bonding Insuf icient 40 W 40 W bonding 50 W 50 W y y x x 500µm 500µm Process optimisation Productivity 96% density 4.5mm y x DAM v2 system Traversing laser head DAM v2 – SS316L and Invar processing (4w) DAM v2 Ti64 processing (single layer) Diode Area Melting Concept Future work focused on enhanced thermal control with novel pre/post-heat linear arrays Control of Ti6Al4V microstructure through scan speed and use of customised laser beam arrays Diode Area Melting Microstructural control Effect of turning individual beam on/off with increased spacing between beams DAM v2 – theoretical productivity Quad laser systems EBM Arcam territory Current DAM setup (theoretical build rate) Have a think about what else could be done to address the issues/challenges we have discussed in this lecture series How to deal will - Porosity - Contamination - Material losses - Stress - Microstructural customisation - Productivity etc Hope you enjoyed Any questions let me know [email protected]