A Level Genetic Technology PDF
Document Details
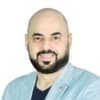
Uploaded by DR.MOATAZ
Tags
Summary
These are notes on genetic engineering and the production of genetically engineered organisms using bacteria, such as in the production of insulin.
Full Transcript
## A LEVEL 19 Genetic Technology ### 19.1 Principles of Genetic Technology **Genetic Engineering** Genetic engineering is the manipulation of genes in living organisms. * Genes extracted from one organism can be inserted into another. This can be done within the same species (for example, in ge...
## A LEVEL 19 Genetic Technology ### 19.1 Principles of Genetic Technology **Genetic Engineering** Genetic engineering is the manipulation of genes in living organisms. * Genes extracted from one organism can be inserted into another. This can be done within the same species (for example, in gene therapy) or genes may be transferred from one species to another. The genes can be extracted from the DNA of a donor organism or synthesized from the mRNA of a donor organism. * Genes can also be synthesized in the laboratory by linking DNA nucleotides together in a particular order, and then inserting this gene into an organism. The transferred gene is then expressed in the recipient organism. * Both these processes produce recombinant DNA - that is, DNA whose base sequences would not normally be found in an organism. **Producing Genetically Engineered Organisms** A wide range of techniques are used to genetically engineer organisms. In order to understand how genetic technology can produce recombinant organisms, we will look at the production of recombinant DNA containing the human insulin gene and its insertion into bacteria, which then express the gene and make insulin. * Insulin is a small protein. It is a hormone secreted by β cells in the islets of Langerhans in the pancreas in response to raised blood glucose concentration (pp. 157-160). * In type I diabetes, no or insufficient insulin is secreted, and the person has to inject insulin. This used to be obtained from animals such as pigs. Today, almost all insulin used in this way is obtained from genetically modified bacteria. **Identifying the Insulin Gene** * The amino acid sequence of insulin was already known. From this, the probable base sequence of the gene that codes for it, and of the mRNA transcribed from the gene, could be worked out (Figure 19.1). **Making the Human Insulin Gene** * Messenger RNA was extracted from β cells. These cells express the gene for insulin, so much of this mRNA had been transcribed from this gene. * The appropriate mRNA was then incubated with the enzyme reverse transcriptase, which built single-stranded cDNA molecules against it. These were then converted to double-stranded DNA - the insulin gene (Figure 19.2). * The human insulin gene, like all eukaryotic genes, is composed of exons (coding sequences) and introns (non-coding sequences). Prokaryotic genes do not have introns and so prokaryotes do not have the ability to splice them out of the RNA produced by transcription. By making cDNA from the mRNA, there are no introns present, so the bacteria can produce the correct polypeptide. * Some extra single-stranded DNA was then added to each end of the DNA molecules. These are called sticky ends. Because they are single-stranded, they are able to form hydrogen bonds with other single-stranded DNA with complementary base sequences, enabling DNA molecules to join up with one another. **Cloning the DNA** * Multiple copies of the DNA were then made (Figure 19.3) using PCR (pp. 213-214). This process uses an enzyme called DNA Taq polymerase to copy the DNA. **Inserting the DNA into a Plasmid Vector** * A plasmid is a small, circular DNA molecule found in many bacteria. A vector is something that is used to transfer the DNA from one organism or cell to another. * A plasmid was cut open using a restriction endonuclease. * The restriction endonucleases made a stepped cut across the DNA molecule, leaving single-stranded regions (Figure 19.4). * The cut plasmids and the insulin gene (the cloned DNA) were then mixed together, along with the enzyme DNA ligase. * Complementary base pairing took place between the sticky ends added to the insulin genes and the sticky ends of the cut plasmids. * DNA ligase then joined up the sugar-phosphate backbones of the DNA strands. This resulted in closed plasmids containing the insulin gene (Figure 19.5). * Genes conferring resistance to an antibiotic were also introduced into the plasmids, next to the insulin gene. * Not all of the plasmids took up the gene. Some just closed back up again without it. **Inserting the Plasmid into a Bacterium** * The plasmids were mixed with a culture of the bacterium Escherichia coli. * About 1% of the bacteria took up the plasmids containing the insulin gene (Figure 19.6). **Identifying the Genetically Modified Bacteria** * Antibiotics were then added to the culture of E. coli bacteria. * The only bacteria that survived were the ones that had successfully taken up the plasmids containing the antibiotic resistance gene. * Most of these plasmids would also have contained the insulin gene. Most of the surviving E. coli bacteria were therefore ones that now contained the human insulin gene (Figure 19.7). **Cloning the Bacteria and Harvesting the Insulin** * The bacteria were then grown in fermenters, where they were provided with nutrients and oxygen to allow them to reproduce to form large populations (Figure 19.8). * Bacterial reproduction is asexual, so all the bacteria were genetically identical (clones). * These GM (genetically modified) bacteria are now cultured on a large scale. The bacteria synthesize and secrete insulin, which is harvested from the fermenters and purified before sale. **Promoters** * Each gene is associated with a region of DNA called a promoter (pp. 188–190). * The promoter partially controls when and where a gene is transcribed. * The enzyme RNA polymerase must bind to the promoter before it can begin transcribing the DNA to produce mRNA. * It was therefore important to ensure that there was a promoter associated with the human insulin gene when it was inserted into E. coli. * When genetically engineering organisms, promoters can be used to control when and where a gene is switched on. For example, a promoter that only switches a gene on in udder cells could be used to create goats that produce a particular protein in their milk. **Marker Genes** * The antibiotic resistance genes added to the plasmids along with the human insulin gene act as markers. They make it possible to identify the bacteria that have taken up the gene. * There is concern that using antibiotic resistance genes as markers could increase the likelihood of the development of populations of harmful bacteria that are resistant to antibiotics. * Today, the most common markers used are genes that code for the production of green fluorescent protein (GFP). These genes were originally taken from fluorescent jellyfish. * The gene for this protein can be inserted along with the desired gene. Cells that fluoresce green when ultraviolet light is shone onto them are therefore likely to have taken up the desired gene. **Gene Editing** Recent research has led to the development of a new method of genetic engineering called gene editing. This is a way in which specific sites in the genome can be targeted so that specific sequences of DNA can be inserted, deleted or replaced to correct harmful mutations. The method is called CRISPR-Cas9 technology, and the basic steps are as follows (Figure 19.9): * A guide RNA is synthesized - this has a sequence complementary to the section of DNA that is to be edited. * The guide RNA is inserted into cells along with an enzyme called Cas9. The guide RNA binds to the targeted section of DNA. * The Cas9 enzyme cuts the DNA where the guide RNA has bound. * Another piece of DNA can now be inserted into the target area and the cells' enzymes naturally rejoin the phosphodiester backbone of the DNA. * CRISPR-Cas9 can be used to delete sections of DNA, insert sections of DNA or swap a harmful, mutated allele for a 'correct' allele. **Polymerase Chain Reaction** The polymerase chain reaction (PCR) is an automated method of making multiple, identical copies of (cloning) a tiny sample of DNA. * PCR involves the exposure of the DNA to a repeating sequence of different temperatures, allowing different enzymes to work. The process shown in Figure 19.10 is repeated over and over again, eventually making a large number of identical copies of the original DNA molecule. * The primer is a short length of DNA with a base sequence complementary to the start of the DNA strand to be copied. This is needed to make the DNA polymerase begin to link nucleotides together as it makes a copy of the exposed DNA strand. * The DNA polymerase that is used in PCR is called Taq polymerase. This enzyme was sourced from a bacterium called Thermophilus aquaticus, which lives in hot springs in Yellowstone Park in the USA. Its enzymes are stable even at high temperatures, which is why it is suitable for catalysing DNA synthesis in PCR. **Gel Electrophoresis** Gel electrophoresis is a way of separating fragments of DNA of different lengths. * It does this by applying an electrical potential difference across a gel in which a sample is placed. Lengths of DNA of different masses, or with different charges, will move across the gel at different speeds. * Before electrophoresis of DNA is carried out, the sample of DNA is exposed to a set of restriction enzymes (restriction endonucleases). These enzymes cut DNA molecules where particular base sequences are present. For example, a restriction enzyme called BamH1 cuts where the base sequence -GGATCC- is present on one strand of the DNA. Other restriction enzymes target different base sequences. This cuts the DNA into fragments of different lengths. * To carry out gel electrophoresis, a small, shallow tank is partly filled with a layer of agarose gel. * A mix of the DNA fragments to be separated is placed in wells at one end of the gel. * A potential difference is applied across the gel, so that a direct current flows through it. * DNA fragments carry a small negative charge, so they slowly move towards the positive terminal. The larger they are, the more slowly they move. * After some time, the current is switched off and the DNA fragments stop moving through the gel. * The DNA fragments must be made visible in some way, so that their final positions can be determined. This can be done by adding fluorescent markers to the fragments (Figure 19.11). * The DNA in the gel can be permanently transferred to nylon membranes in a process known as Southern blotting. Small single strands of DNA (probes) made using radioactive isotopes, and with base sequences thought to be similar to those in the DNA fragments, can be washed over the nylon membrane with the DNA. The probes pair up with fragments that have complementary base sequences, so their positions are now emitting radiation. This can be detected by its effects on a photographic plate. **Microarrays** A microarray is a tool used to identify particular DNA sequences in a sample of DNA. The microarray is a small piece of glass (often 2cm²) to which DNA probes (see Figure 19.11) have been attached in a regular pattern. As many as 10000 different probes can be attached to one microarray. * The DNA to be tested is cut into fragments using restriction endonucleases, and is then exposed to the microarray. * Fragments whose base sequences are complementary to one of the probes will attach to it by complementary base pairing. * The microarray is then washed, which removes all the fragments that have not bound to any of the probes. * The microarray can then be 'read' by a computer, which will identify the probes to which DNA fragments have attached, and therefore the base sequences present in the DNA sample. * This technique can be used to compare the genome (all the DNA present) in two different species, or in two individuals of the same species. * The technique can also be used to identify which genes are being expressed in a cell. mRNA is extracted from the cell, and then used to make cDNA (see p. 211). The cDNA is then analyzed using a microarray. Any DNA that attaches to the probes must represent a gene that was being expressed and producing mRNA in the cell. **Using Databases** When new gene sequences or whole genomes are discovered, most are added to global genetic databases. * There are now databases listing the base sequences of all the DNA in several individual people, as well as databases for the genomes of other species, such as the nematode worm Caenorhabditis and the malarial parasite Plasmodium. There are also databases for the proteomes of different species. (The proteome is all the different proteins in an organism.) * The information stored in these databases is available to scientists all over the world. There are many potential uses for it. **Uses of Genetic Databases** * People have different combinations of alleles of genes in their cells, and this can affect their susceptibility to diseases and the way in which drugs work in their bodies. It should one day be possible to determine which is the best drug to give to a person with a particular DNA profile. * Knowing the detailed DNA sequences of different species or varieties of Plasmodium can help scientists to develop drugs that will act against them. * Matches can be made between DNA sequences and protein sequences, which can help to unravel how genes affect metabolism and therefore health. * Models can be made of enzymes using amino acid sequences derived from the proteome, and drugs can then be designed that could inhibit these enzymes, using computer modelling. AI (artificial intelligence) is helping to make these processes much faster. * Because the genetic code is universal, we can work out the amino acid sequence of proteins and help to predict the structures of proteins. * When someone sequences a new gene, they can search the database and look for similar genes to help them understand its function. * When trying to classify species, databases can be searched with DNA sequences to find the most similar organisms. ### 19.2 Genetic Technology Applied to Medicine **Advantages of Producing Human Proteins by Gene Technology** Examples of human proteins produced by GM organisms include: * Insulin for the treatment of diabetes, produced by E. coli (see pp. 210-212) * Factor VIII for the treatment of haemophilia, produced by tissue cultures of GM hamster cells * Adenosine deaminase for the treatment of severe combined immunodeficiency (SCID), produced by GM bacteria There are numerous advantages of using proteins produced in this way. For example: * The insulin produced by the genetically engineered E. coli is identical to human insulin, because it is made by following the genetic code on the human insulin gene. Insulin obtained from the pancreas of an animal is slightly different, and therefore may have different effects when used to treat diabetes in humans. * Large quantities of insulin can be made continuously using E. coli, and this can be done under controlled conditions. Only small quantities of insulin can be obtained from the pancreas of an animal, and it is not easy to purify the insulin to produce a standard product that is safe for medicinal use. * Many religions and cultures, and also many individuals, are against the idea of harvesting insulin from a dead animal for use in humans. **Genetic Screening** Finding out the genes that a person has is called genetic screening. It can be used to see if people actually have a genetic condition or to see if they are carrying one that could be passed on to their children. Examples of genetic screening include the following: * Identifying people who are carriers, i.e. who have a copy of a harmful recessive allele, such as the cystic fibrosis allele. A couple with one of these genetic conditions in the family could therefore find out if they are both heterozygous and therefore might have a child with the condition. * Preimplantation genetic diagnosis, which involves checking the genes of an embryo produced in vitro (i.e. by fertilization outside the body) before it is placed in the mother's uterus. This can ensure that only embryos that do not have the genes for a genetic disease are implanted. * Prenatal testing, i.e. checking the genes of an embryo or fetus in the uterus. This could enable the mother to decide to have her pregnancy terminated if the baby would have a genetic disease. * Identifying people who will develop a genetic condition later in life. For example, Huntington's disease is caused by a dominant allele, but does not manifest itself until middle age. A person with this disease in the family could check if they have the gene before they decide to have children themselves. * Identifying people with alleles that put them at risk of developing other diseases. For example, a woman who has relatives with breast cancer could find out if she has the BRCA1 or BRCA2 alleles, which are known to be associated with an increased risk of breast cancer, and could decide to have a mastectomy in order to prevent developing the illness. A genetic counsellor helps people to interpret the results of screening and therefore to make decisions. This can be difficult, and involves moral and ethical, as well as scientific, issues. For example, if a pregnant woman finds that her child will have cystic fibrosis, are these sufficient grounds to have her pregnancy terminated, or does the child have a right to life? **Cystic Fibrosis** * Cystic fibrosis (CF) is a genetic condition resulting from a mutation in a gene that codes for a transporter protein called CFTR. This protein lies in the cell surface membrane of cells in many parts of the body, including the lungs, pancreas and reproductive organs. The CFTR protein actively transports chloride ions out of cells. * There are several different mutations in the CFTR gene that result in changes in the CFTR protein. In all cases, the protein that is made does not work properly. * Normally, chloride ions are transported out of the cells through the CFTR protein. Water follows by osmosis. When the CFTR protein is not working, this does not happen. There is therefore less water on the outer surface of the cells than there should be. The mucus that is produced in these areas therefore does not mix with water in the usual way. The mucus is thick and sticky. As a result: * The abnormally thick mucus collects in the lungs, interfering with gas exchange and increasing the chance of bacterial infections * The pancreatic duct may also become blocked with sticky mucus, interfering with digestion in the small intestine * Reproductive passages, such as the vas deferens, may become blocked, reducing fertility **Treating Genetic Conditions Using Gene Therapy** Gene therapy is the treatment of a genetic disease by changing the genes in a person's cells. * Although attempts have been made to treat several different diseases using gene therapy, and in the future gene therapy may be a common treatment, there are still many problems to be solved before treatments become widely available and successful. * The genes are transferred to tissues using liposomes or viruses. In a few cases, trials have been carried out using 'naked' DNA. **Severe Combined Immunodeficiency (SCID)** * SCID is a genetic condition caused by a lack of the enzyme adenosine deaminase. * It results from mutations in the gene for the enzyme, which result in it not being expressed. * Trials have been carried out using viruses to transfer the normal allele into white blood cells taken from a person with SCID. These trials have been successful, with many patients now having a functional immune system. * In some cases this has resulted in genes controlling cell division becoming altered and causing cancer (leukaemia). Gene editing, using CRISPR, may be a future option for editing the DNA safely. **Inherited Eye Diseases** * Choroideraemia is a genetic condition in which cells in the retina gradually die, and is due to a faulty allele of the CHM gene on the X chromosome. * Several patients have now had their eyesight partially restored after viruses carrying normal alleles of the CHM gene were injected beneath their retina in one eye. **Social and Ethical Considerations of Using Genetic Screening and Gene Therapy** The ability to detect genetic conditions, and perhaps to cure some of them, raises ethical questions that society needs to debate. You might like to think about some of the questions raised by the examples given below, and consider the answers to those questions. There are no right or wrong answers. Try to think about two different viewpoints for each one. * A person undergoes genetic screening and finds that they have a genetic condition that cannot be treated. How does this affect their life? * A couple undergo genetic screening and find that they both have a recessive allele for an unpleasant but not life-threatening genetic condition. What do they do? * A mother finds that her embryo will have cystic fibrosis. Does she have her pregnancy terminated? * A person with a genetic condition that affects their quality of life but is not life threatening is offered a place in an experimental gene therapy trial. What are the benefits and risks of getting involved with the trial? * A couple very much want a baby girl rather than a boy. They decide to have IVF and have the sperm screened so that they can choose a sperm carrying an X chromosome to fertilise the egg. Should this be allowed? * Should it be the decision of humans to decide which embryos are genetically correct and which are not genetically correct? ### 19.3 Genetically Modified Organisms in Agriculture We have seen that crops can be improved by selective breeding. However, this takes a long time, and can only involve alleles that are already present in the genome of that crop species. Genetic engineering allows the targeted addition of alleles for a desired characteristic into a species where that allele is not naturally present. It may help to solve the global demand for food. **Examples of GM Crops and Livestock** **GM Salmon** * Salmon are farmed for food in many parts of the world. * One GM variety of salmon contains a growth hormone gene from another species of salmon, and a promoter from a different species of fish. This makes the GM salmon grow much faster and larger than normal. * Growing GM salmon could allow more food to be produced more quickly. * There are concerns that, if these fish escape from the cages where they are kept, they could reproduce in the wild and adversely affect populations of other marine organisms. To avoid these problems, it is planned to farm only sterile female fish. Moreover, the GM salmon are all triploid, making it difficult for them to produce viable gametes. It is also recommended that the fish are only grown in land-based water tanks, so that they are much less likely to escape into the wild.