Nervous Tissue PDF
Document Details
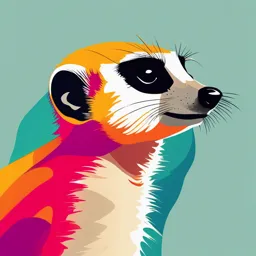
Uploaded by LogicalBaroque
University of Veterinary and Pharmaceutical Sciences Brno
Alvin J. Beitz, Thomas F. Fletcher
Tags
Summary
This document provides a detailed overview of nervous tissue. It describes neuronal structure, including the cell body, dendrites, and axon. The document also discusses neuroglia and types of neurons present in the peripheral nervous system.
Full Transcript
6 Nervous Tissue ALVIN J. BEITZ THOMAS F. FLETCHER Neurons...
6 Nervous Tissue ALVIN J. BEITZ THOMAS F. FLETCHER Neurons Peripheral Nervous System Gliocytes Neuronal Structure Ganglionic gliocytes Cell body Neurolemmocytes Nucleus Myelin Sheath Cell body cytoplasm Peripheral Nervous Tissue Neuron processes Nerves Dendrites Ganglia Axon Efferent Neurons Terminal branches (telodendrites) Receptors Classification Nonencapsulated receptors Regions of a Neuron Encapsulated receptors Neuronal Communication Central Nervous Tissue Interneuronal chemical synapses Cerebral Cortex Synaptic ultrastructure and function Cerebellum Neuroglia Spinal Cord Central Nervous System Gliocytes Meninges, Blood Vessels, and Cerebrospinal Fluid Astrocytes Meninges Oligodendrocytes Blood Vessels Microglia Cerebrospinal Fluid Ependymal cells Nervous tissue parenchyma consists of neurons and support- NEURONS ive cells called neuroglia. Nervous tissue forms the nervous system, which may be divided into the central nervous system Neurons are the structural and functional units of the nervous and the peripheral nervous system. The central nervous sys- system. They are also trophic units because often they transform tem (CNS) includes the brain and spinal cord. The peripheral and sustain what they innervate. Generally, neurons must last a nervous system (PNS) consists of cranial and spinal nerves, in- lifetime because, with some exceptions (e.g., olfactory neurons cluding associated nerve roots and ganglia. Nerves and ganglia and neuronal stem cells), mature neurons are incapable of mito- that innervate viscera are designated the autonomic nervous sis. However, recent evidence of new neuron formation in several system. regions of the brain has been discovered. 91 92 Dellmann’s Textbook of Veterinary Histology Morphologically, neurons feature elongated processes, termed easily excited to threshold than larger ones; that is, less synaptic axons and dendrites, that extend variable distances from the cell activity is required to trigger action potentials in small neurons body (perikaryon) (Fig. 6-1). Metabolically, neurons are actively (which have high input impedance, by virtue of their size). involved in maintaining their structural integrity and in synthe- sizing, packaging, transporting, and releasing secretory products. Nucleus Neurons specialize in excitability and they communicate by Typically, the nucleus of a neuron is centrally positioned, spher- releasing chemical agents (neurotransmitters, neuromodulators, or ical or ovoid, and relatively euchromatic, reflecting its high syn- neurohormones). Excitation involves ions passing through protein thetic activity. Small neurons have rather heterochromatic nuclei. channels embedded in neuronal plasma membrane that otherwise Neurons in autonomic ganglia have eccentric nuclei. Nuclear acts as a hydrophobic barrier to ion flow. Via chemical secretion, size is proportional to neuron size. The nucleus appears relatively neurons transmit excitation to other neurons or to muscle or glands. large in a neuron because the cell body cytoplasm surrounding it represents only a minor fraction of total cell volume. Neuronal Structure A prominent nucleolus is evident within the nucleus. In females of some species, sex chromatin (Barr body) may be evi- Neurons assume a variety of shapes and sizes, related to their func- dent in the vicinity of the nucleolus (cats, rodents) or nuclear tional roles. Neuronal processes are configured according to the membrane (primates). connections that must be made. Neurons with processes that ex- tend long distances must necessarily be larger than less extensive Cell body cytoplasm neurons. Also, because larger processes conduct more rapidly than Many proteins are synthesized in the cell body cytoplasm of the smaller ones, neurons that convey urgent information are large. neuron, including cytoskeletal proteins (e.g., for neurofilaments and microtubules), membrane proteins (e.g., for ion channels and Cell Body active transport), enzymatic proteins (e.g., for glucose metabo- The cell body (perikaryon; soma) of a neuron consists of the nu- lism and neurotransmitter synthesis), and secretory peptides cleus plus the surrounding cytoplasm and plasma membrane (e.g., neuromodulators and neurohormones). (Fig. 6-1). In routinely stained histologic sections, the cell body The cell body cytoplasm of large neurons stained with ani- is the most identifiable feature of a neuron. Cellular constituents line dyes and examined by light microscopy features clumps of are synthesized within the cell body and then flow distally into chromatophilic substance (Nissl substance), which represent neuronal processes (axon and dendrites). aggregations of rough endoplasmic reticulum (rER), free ribo- Cell bodies range from less than 10 µm to more than 100 µm somes, and polyribosomes. Chromatophilic substance extends in diameter. Cell body size is proportional to neuronal total vol- into the trunks of dendrites, but it is absent from the axon and ume, although the cell body itself represents a minor portion of axon hillock, a pale-staining region of the cell body where the the total volume (and an even smaller fraction of total surface axon originates. Instead, the hillock contains neurofilaments area). In multipolar neurons, where the cell body plasma mem- and grouped microtubules. In small neurons, cytoplasmic chro- brane integrates synaptic input, smaller cell bodies are more matophilia appears relatively pale and diffuse. FIGURE 6-1 Schematic illustration of a typical (multipolar) neuron, showing multiple dendrites and one axon emanating from the cell body. Functional regions include a dendritic zone (cell body and dendrites), which receives synaptic input; telodendritic zone (axon terminals), which makes synaptic contact with other neurons; and the axon, which conducts excitation between the two zones. This axon is drawn myelinated. The myelin insulation is interrupted by nodes (gaps). The myelin of each internode is formed by individual glial cells (not shown). Nervous Tissue Alvin J. Beitz and Thomas F. Fletcher 93 When a neuron is injured (e.g., by transection of its axon), the drite emerges as a main trunk that branches repeatedly into smaller cell body swells, the nucleus shifts to an eccentric position, and ri- and smaller twigs. The trunk has an organelle content similar to bosomes disperse so that chromatophilic substance disappears from that of the cell body. Small dendritic branches feature predomi- the center of the cell body (chromatolysis). This response to in- nantly microtubules, augmented by neurofilaments, mitochon- jury, called the axonal reaction (Fig. 6-2), begins within days of dria, and smooth endoplasmic reticulum (sER). the injury and may persist several weeks. The reaction is consid- Synaptic sites on dendrites are distinguished by a band of ered pathologic evidence of nervous tissue damage in the CNS. electron-dense cytoplasm of variable thickness that lines a region Organelles in the cell body cytoplasm of the neuron include of postsynaptic membrane that faces a presynaptic element plentiful mitochondria, which support the aerobic energy needs across a synaptic cleft (Fig. 6-3). The dense material represents of the cell, and a prominent Golgi complex. Secretory vesicles proteins (receptors, channels, enzymes, etc.) responsible for post- originate from the complex and are transported through the axon synaptic activity. to synaptic boutons (expansions of the axon terminal). Secretory vesicles commonly contain neuroactive peptides that influence the excitability or growth of target cells. In the case of certain hypothalamic neurons, secretory vesicles contain neurohormones that are released in proximity to blood vessels and ultimately enter the bloodstream. Synaptic vesicles containing neurotransmitters are con- centrated in axon terminals, where they fuse with plasma mem- brane and release neurotransmitter into a synaptic cleft. Long-lived neurons may accumulate lipofuscin granules in the cytoplasm as a residue of lysosomal activity. Microtubules (25 nm in diameter) and neurofilaments (10 nm in diameter) are numerous in the cell body. Microtubules are involved in the rapid transport of membrane-bounded organelles within the neuron. Groups of neurofilaments, re- ferred to as neurofibrils, can be visualized with light microscopy. Neuron Processes A typical neuron has a single axon and multiple dendrites origi- nating as processes from its cell body (Fig. 6-1). The axon ends in terminal branches that synapse with dendrites and cell bodies of other neurons or innervate muscle or glandular epithelium. Each type of neuronal process has a distinct functional role within the neuron. Thus, the processes have different populations of plasma membrane proteins (channels, receptors, transporters, pumps). Dendrites Dendrites are highly branched processes designed to receive nu- merous synaptic contacts from other neurons. Treelike, each den- FIGURE 6-3 Schematic illustration of an axodendritic synapse. The terminal bulb of an axon is separated from a dendrite (D) by a synaptic cleft (SC) containing glycoprotein material. Astrocyte processes (A) border the synaptic cleft bilaterally. Within the terminal bulb, synaptic vesicles (S) are clustered around an active zone in which an electron-dense band lines the plasma membrane. As a consequence of Ca++ influx, synaptic vesicles are able to dock with the plasma membrane and release neurotransmitter molecules by exocytosis. Vesicle membrane is recovered from the plasma membrane and conveyed, as coated vesicles (C), to smooth endoplasmic reticulum (sER) for recycling. A solitary, large, dense- FIGURE 6-2 Two light micrographs at different magnifications core secretory vesicle (possibly containing a peptide or monoamine of chromatolytic cell bodies from the spinal cord of a dog that had neuroactive substance) is also shown (V), along with mitochondria, spinal nerves transected. The cell bodies are swollen, nuclei are microtubules, and neurofilaments. The transverse section of the shifted to an eccentric position, and chromatophilic substance is lost dendrite features an electron-dense band (E) along the postsynaptic except for small amounts accumulated marginally. Nissl’s stain (left, membrane. Numerous microtubules and neurofilaments and a few ×200; right, ×400). sER profiles are evident in the dendrite. 94 Dellmann’s Textbook of Veterinary Histology Many neurons display numerous dendritic spines (gem- found in parasympathetic ganglia or in the brain, the axon orig- mules), the postsynaptic partner in many excitatory synapses. A inates as a branch of a dendrite. Except at its termination, axonal spine is a short expanded process attached to the dendritic branch branches are sparse. When branches are present along the course by a narrow stalk, like a bud on a twig (Fig. 6-4). A spine ap- of the axon, they emerge at right angles (from nodes in the case paratus, consisting of alternating membrane sacs and dense ma- of myelinated axons) and are called collateral branches. Axo- terial, may be found within the spine. Spines increase dendritic plasm, the cytoplasm of the axon, contains microtubules, neuro- surface area and act to restrict the spread of postsynaptic excita- filaments, mitochondria, and sER. Devoid of rER, axoplasm is not tion. For a century or more, dendritic spines were considered very well stained by hematoxylin and eosin and other routine histo- static structures, but within the last 5 years, research has shown logic stains. that the structure of the spine is very dynamic. Time-lapse im- The initial segment of the axon is a site just distal to the aging shows that adult spines can change shape by as much as axon hillock, where action potentials normally originate. It is 30% of their length or width within a few seconds to minutes. narrower than the rest of the axon. Ultrastructurally, bundled Furthermore, high-frequency stimulation induces protrusion of microtubules are present and electron-dense material is evident new dendritic processes as well as spine bifurcation, also within along the inner surface of the plasma membrane of the initial seg- minutes. These data suggest that changes in spine number or ment. The density represents protein accumulation associated shape may contribute to synaptic plasticity, perhaps by provid- with ion channels and pumps. Similar electron-dense protein ing a lasting structural substrate for newly encoded memories. accumulations are also found at nodes of myelinated axons. A slow flow of cytoplasm (1 mm/day) and a slow transport of Axon cytoskeletal elements (10 mm/day) progress along the axon, from The axon is a relatively long, cylindrical process that originates axon hillock to terminal branches (anterograde transport). In ad- from the axon hillock of the cell body and ends in terminal dition, axons have a fast transport capability (up to 400 mm/day) branches and synaptic boutons, which are described below. While involving microtubule-linked movement of mitochondria and the axon typically emerges from the cell body, in some neurons constituents packaged in vesicles. There is also a retrograde trans- port mechanism that rapidly conveys remnants of organelles from terminal branches back to lysosomes within the cell body. Viruses (e.g., rabies) and neurotoxins (e.g., tetanus toxin) can also be carried to the cell body by this route. Axons are capable of regenerative conduction; that is, they convey an excitation signal to the end of the axon with the same magnitude as it had when it began at the initial segment. Large axons conduct more rapidly than small ones, but to further in- crease conduction velocity, large axons are myelinated (coated with a myelin sheath formed by glial cells). Small nonmyelinated axons are ensheathed by glial cells in peripheral nerves, but they are not ensheathed at all in the CNS. Axon degeneration may be causally linked to the docu- mented physiologic and behavioral deficits observed in aged animals. Traditionally, the decline in memory function and be- havioral dysfunction that accompany normal aging in animals have been attributed to loss of neurons in certain areas of the brain. Recent data, however, show that aging does not induce prominent cell loss in the brain, but rather leads to degeneration of axons that innervate certain forebrain structures. Terminal branches (telodendrites) Axons terminate by successively branching (Fig. 6-1). The col- lective terminal branches constitute the axon terminal (teloden- dritic) zone of a neuron. Each terminal branch ends in an expansion called a terminal synaptic bulb (synaptic bouton). Along the non- myelinated terminal branches, synapses occur at multiple focal swellings called axonal varicosities (preterminal boutons). The FIGURE 6-4 A pyramidal neuron from the cerebral cortex is shown (Golgi silver impregnation). This multipolar neuron has a number of axonal varicosities is not insignificant; a single hip- pyramidal cell body from which apical and basal dendrites arise. A pocampal cell axon makes 50,000 synapses over a distance of only single axon (not visible) leaves the lower surface of the cell body. 200 mm. Both axonal varicosities and terminal synaptic boutons The insert, an enlargement of the middle of the apical dendrite, are sites where neurotransmitter molecules are packaged and shows dendritic spines. stored within synaptic vesicles. Nervous Tissue Alvin J. Beitz and Thomas F. Fletcher 95 The most common synaptic vesicle is spherical (40 to 50 nm mechanism of neural plasticity, enabling neural development in diameter) with an electron-lucent (agranular) core (Figs. 6-3 and and subsequently behavioral modification and learning. 6-5). Such vesicles may contain any one of a number of neuro- transmitters. Electron-lucent synaptic vesicles that appear flat- Classification tened when exposed to solutions of high osmolarity are associated with inhibitory synapses. Some neurons have spherical vesicles (40 Neurons are anatomically classified as unipolar, bipolar, or multi- to 60 nm in diameter) with an electron-dense (granular) core. The polar according to the number of processes that emanate from the electron-dense synaptic vesicles usually contain dopamine or nor- cell body (Fig. 6-6). epinephrine molecules. In a unipolar neuron, the cell body gives off a single axon that Synaptic vesicle proteins are synthesized in the cell body and soon bifurcates into central and peripheral branches. The periph- transported rapidly to axon terminal sER, where they supplement eral branch terminates in receptors that are sensitive to environ- local production of synaptic vesicles from recycled vesicular mem- mental energy. The central branch conveys the environmentally brane. Transporter proteins in vesicle membrane load newly formed induced excitation into the CNS. Unipolar cell bodies are found synaptic vesicles with neurotransmitter molecules that were also re- in sensory ganglia located in roots of cranial and spinal nerves. cycled. Linked by cytoskeletal actin filaments, synaptic vesicles are Mammalian unipolar neurons are often referred to as pseudo- clustered together, in ready reserve for plasma membrane docking unipolar, because they originate as bipolar cells and only become and exocytosis during synaptic activity (Fig. 6-3). In addition to unipolar during development. The unique geometry of unipolar synaptic vesicles, terminal branches may contain secretory vesi- sensory neurons can lead to the generation of spontaneous dis- cles that store neuroactive peptides (several dozen have been iden- charge in the cell bodies, potentially disrupting the fidelity of tified). Peptides are found in spherical, electron-dense vesicles that afferent signaling in normal animals. More importantly, follow- are relatively large (100 to 200 nm in diameter). The secretory vesi- ing nerve injury, discharge originating ectopically within spinal cles are synthesized in the cell body and transported to preterminal ganglion cells is greatly augmented and can be a major contrib- and terminal synaptic boutons for storage and release. The peptides utor to neuropathic dysesthesias and chronic pain. generally act as neuromodulators (agents that augment neurotrans- In a bipolar neuron, two processes emanate from the cell mitter effects). Secretory vesicles of certain hypothalamic neurons body, which is situated either within the axon (vestibulocochlear contain peptide hormones (e.g., vasopressin and oxytocin are stored afferent neurons; bipolar cells of the retina) or at the juncture of and released in the neurohypophysis). the axon and a solitary dendrite (olfactory afferent neurons). Like Sprouting of new terminal branches and degenerations of unipolar neurons, bipolar neurons are afferent neurons that con- existing branches in response to environmental change is a major vey sensory information to the CNS. 4 5 7 8 1 3 2 9 6 10 FIGURE 6-5 Schematic illustrations of types of central nervous system synapses. 1. Axosomatic synapse with electron-lucent, spherical, synaptic vesicles. 2. Axosomatic synapse with synaptic vesicles that are flattened (an artifact associated with inhibitory synapses). 3. Axosomatic synapse with electron-dense synaptic vesicles. 4. Axodendritic synapse. 5. Axoaxonic synapse in which one terminal bulb synapses on another (associated with presynaptic inhibition). 6. Synapse on an axon hillock, close to the initial segment of the axon. 7. Axodendritic synapse on a dendritic spine. 8. Relatively elaborate synapse on a dendritic spine. 9. Dendritic spine exhibiting a spine apparatus (smooth endoplasmic reticulum plus electron-dense material). 10. Three axonal varicosities making synapses in passing (en passant). 96 Dellmann’s Textbook of Veterinary Histology distinct regions: (a) an input region or dendritic zone, where excitation is initially received; (b) an output region or telo- dendritic (axon terminal) zone, where excitation is transmit- ted to other cells; (c) an axon, which conducts excitation between the dendritic and axon terminal zones; and (d) a cell body, which nurtures the cell. In a typical multipolar neuron (Fig. 6-1), the receptive den- dritic zone features a large surface area encompassing the cell body and highly branched dendrites. An elongated, cylindrical axon originates from the cell body. The axon terminal zone is a highly branched region located at the distal end of the axon. The terminal branches feature localized expansions (boutons or bulbs) where neurotransmitter molecules are stored and released at synapses. In the case of a unipolar afferent neuron, the cell body is sit- uated along the axon and the dendritic zone consists of receptors that change environmental energy into neural excitation. Dendritic bipolar unipolar multipolar zones of bipolar afferent neurons may involve receptors (olfaction) neuron neuron neuron or synaptic contact with receptor cells (in the retina and inner ear). From dendritic zones, excitation is conveyed along the axon to terminal branches within the CNS. The protein composition of the plasma membrane is necessarily different at each functional region of a neuron. For example, dendritic zone membrane has ligand-reactive protein receptors that open ion channels, either directly or through sec- ond messengers. Here the term “receptor” refers to membrane proteins interacting with extracellular physiologic signals and converting them into intracellular effects. Axon membrane has voltage-gated Na+ channels that enable membrane polarity re- versal and regenerative conduction. The membrane of termi- nal branches and synaptic boutons has voltage-gated Ca++ channels (Ca++ is involved in the release of neurotransmitter mole- cules) and membrane-associated receptors for reuptake of released neurotransmitter. FIGURE 6-6 Schematic illustration of a bipolar neuron (left), a Neuronal Communication unipolar neuron (center), and a multipolar neuron (right). Neurons are anatomically classified according to the number of processes Neurons communicate with one another, with glial cells, and emanating from the cell body. Unipolar and bipolar neurons are with the muscles and glands that neurons innervate. Alterations sensory. Most neurons are multipolar; however, their shapes vary in excitation communicated from neuron to neuron (neural cir- considerably. The cytoplasm of the cell bodies features clumps of cuits) constitute the basis of nervous system function. chromatophilic substance (Nissl substance). Among the billions of neurons that comprise the nervous system, the primary means of communication is localized release of neurotransmitter molecules at interneuronal chemical synapses. In a multipolar neuron, the cell body gives rise to multiple Chemical synaptic arrangements are also encountered between branches, several dendrites, and an axon. Nearly all of the billions efferent neurons and the muscles and glands they innervate (see of neurons comprising the CNS are multipolar, as are the neu- Peripheral Nervous Tissue section). A few neurons communicate rons contained in autonomic ganglia of the PNS. through gap junctions (electrotonic synapses), especially in inver- tebrates and fish. In mammals, gap junction communication is common between neuroblasts during embryonic development, Regions of a Neuron but it is relatively rare between mature neurons. Other neurons A typical neuron becomes excited at its input region, conducts communicate by producing a gas that passes freely through neuro- excitation to its output region, and transmits excitation via nal membranes (e.g., the gases nitric oxide and carbon monoxide chemical secretion at synapses (Fig. 6-1). Reception, conduction, are increasingly appreciated as major neurotransmitters; NO and transmission of excitation require functionally different ion and CO transmit signals between neurons by binding to a heme channels and cellular features. Thus, an individual neuron has moiety at the active site of soluble guanylyl cyclase, leading to Nervous Tissue Alvin J. Beitz and Thomas F. Fletcher 97 an increase in the intracellular second messenger molecule cyclic tential continually registers the net effect of total synaptic input guanosine 5′-monophosphate [cGMP]). to the neuron. In turn, the cell body affects the membrane poten- tial at the nearby initial segment of the axon. At any moment, the Interneuronal Chemical Synapses collective synaptic input to a neuron will be sufficient to trigger An interneuronal chemical synapse is a site of morphologic an action potential at the initial segment of the axon or be insuf- specialization where one neuron influences the excitability of ficient to do so. another by releasing neurotransmitter molecules from synaptic The influence that one neuron has on another depends on vesicles. A majority of neurotransmitters are biogenic amines the number of synaptic contacts it makes with the target neuron (e.g., glutamate, glycine, dopamine, norepinephrine, serotonin, and where the synapses are positioned. Synapses close to the ini- acetylcholine, etc.). Generally, each neurotransmitter can inter- tial segment will have much greater influence triggering an ac- act with a variety of membrane receptors. tion potential than will synapses on distal dendrites. A receptor is a site on a membrane protein to which a neuro- transmitter binds briefly. The receptor protein may be a channel Synaptic Ultrastructure and Function that undergoes reconfiguration to allow passage of selective ions, Ultrastructurally, an interneuronal chemical synapse may be iden- or the receptor may be coupled to a G-protein cascade that opens tified by the juxtaposition of a presynaptic element, a synaptic ion channels either directly or indirectly by activating second mes- cleft, and a postsynaptic membrane (Fig. 6-3). The presynaptic sengers (such as cyclic adenosine monophosphate [cAMP]). Second element contains multiple synaptic vesicles clustered around an messengers provide a means of amplifying the scope and impact active zone indicated by electron density (protein accumulation) of a neurotransmitter signal as well as prolonging its time course. just inside the plasma membrane. The synaptic cleft (in the CNS, Autoreceptors (receptors in presynaptic plasma membrane that approximately the same width as the general intercellular space, influence neurotransmitter synthesis and release) and neuro- 20 to 30 nm wide) contains a protein that holds presynaptic and modulators (peptides released from secretory vesicles) typically postsynaptic membranes together. The postsynaptic somato- act via second messengers. dendritic membrane contains many types of protein receptors Ionotropic membrane receptors represent one large class that accumulate to form functional microdomains opposite pre- of neurotransmitter receptors in which the receptor protein is part synaptic terminal boutons that release neurotransmitters. These of an ion channel–receptor protein complex. When activated, the domains are associated with postsynaptic densities that, under the complex opens or closes the ion channel, resulting in a change in electron microscope, appear as electron-dense material facing the membrane permeability. Metabotropic receptors represent the presynaptic active zone. The postsynaptic density, which consists other large class of neurotransmitter receptors in which the re- of adhesive proteins, such as cadherin–catenin complexes, receptor ceptor protein acts via another protein such as a G protein to ac- proteins, subsynaptic scaffolding proteins, and associated compo- tivate or inhibit enzymes and possibly open, close, or modify ion nents of the cell’s cytoskeleton, is involved in the stabilization and channels, ultimately leading to a change in cell function. trafficking of receptors and in signal transduction. Based on the ion channel or the G protein to which a recep- When an action potential arrives at the end of an axon, it pas- tor is coupled, the receptor may be excitatory or inhibitory. Thus, sively depolarizes the presynaptic element (e.g., terminal synaptic it is the nature of the receptor type, rather than the neurotrans- bouton). Voltage-sensitive channels in the presynaptic membrane mitter itself, that determines the function of a synapse (e.g., ex- open to allow Ca++ influx. Elevated cytoplasmic Ca++ activates en- citatory or inhibitory, short or long acting, etc.). Nonetheless, zymes that phosphorylate synaptic vesicle proteins responsible for some neurotransmitters (e.g., glutamate) are associated with ex- reversibly linking vesicles to cytoskeletal actin filaments and merg- citatory synapses, and others (e.g., glycine) are associated with ing vesicles with plasma membrane. This mobilizes synaptic vesi- inhibitory synapses. It is worth noting that glutamate receptors cles, enabling them to dock with the plasma membrane and release are the most prevalent excitatory neurotransmitter receptors in several thousand neurotransmitter molecules by exocytosis. The the brain, and they mediate almost all excitatory communica- molecules diffuse into the synaptic cleft and bind with various tions between CNS neurons. Thus, it is perhaps not surprising receptors and transporters. that epileptic seizures are a problem of overactivation of brain Synapses are very dynamic structures and there is constant neurons that are driven mainly by glutamate synapses. movement of receptors into and out of synaptic membranes. Most synapses between neurons involve terminal synaptic Recent data have shown that rapid gain and loss of neurotrans- boutons of one neuron contacting the input region of another mitter receptors from synaptic sites are accounted for by endo- neuron, forming axodendritic or axosomatic synapses (Fig. 6-5). cytosis and exocytosis, as well as by lateral diffusion in the plane of However, among the trillions of synapses in the nervous system, the membrane. These events are interdependent and are regulated every synaptic combination has been observed, including axo- by neuronal activity and interactions with scaffolding proteins. axonic, dendrodendritic, dendrosomatic, somatodendritic, and Thus, it is known that activity-dependent elevation in post- somatosomatic synapses. synaptic intracellular calcium concentration triggers rapid recep- The input region of a typical multipolar neuron receives tor immobilization and local accumulation on the neuronal surface. thousands of synaptic contacts. Excitatory and inhibitory synap- Neurotransmitter receptor movement into and out of the synap- tic effects are collectively summated and integrated at the cell tic membrane is one of the core mechanisms for rapidly changing body of the target neuron, so that the cell body membrane po- the number of functional receptors during synaptic plasticity. 98 Dellmann’s Textbook of Veterinary Histology Synaptic activity ceases when neurotransmitter molecules Astrocytes are removed from the synaptic cleft (or degraded in the case of With routine stains, astrocytes are identified by their pale, ovoid acetylcholine). Molecules are actively transported intracellularly nuclei, which are largest among glial nuclei. With silver stains, by transporters (protein pumps) located in the presynaptic mem- astrocytes exhibit numerous processes that contain glial fibrils. brane or in membranes of adjacent glial cells. Thus, neurotrans- In white matter, the processes are long, slender, and moderately mitter molecules are recycled to minimize the need for synthesis branched; in gray matter, the processes appear shorter and highly within presynaptic cytoplasm. As well, synaptic vesicle mem- branched. Thus, white matter is said to contain fibrous astro- brane is recycled. Vesicle membrane is extracted from the pre- cytes, whereas gray matter contains protoplasmic astrocytes synaptic plasma membrane and transported as coated vesicles to (Fig. 6-7). sER within the presynaptic cytoplasm (Fig. 6-3). Under the electron microscope, astrocytes feature packed bundles of intermediate filaments (8 nm in diameter) and pale cytoplasm. The glial filaments, which are composed of glial fi- NEUROGLIA brillar acidic protein (GFAP), are unique to astrocytes and form the glial fibrils seen with light microscopy. The filaments are Neuroglia (gliocytes) comprise over 90% of the cells that make denser in fibrous astrocytes than in protoplasmic ones. up the nervous system. Recent studies have shown that glia are not Adjacent astrocytes are joined by gap junctions. In response simply the support structure in which neurons are embedded. to local stimulation, a widening excitatory wave of elevated cyto- While the biology of glial cells is poorly understood, it is clear that plasmic Ca++ can spread outward from cell to cell via the gap glial–neuronal and glial–glial interactions are essential for many junctions. The adjacent astrocytes are also linked by small, button- of the critical functions that occur in the nervous system. Neurons like adhering junctions (spot desmosomes). communicate with glial cells by releasing ATP at synapses and Astrocyte processes terminate in expansions called end feet. from axons during conduction. Glial cells have vital roles in neuro- Collections of end feet form a glial-limiting membrane to which nal development, activity, plasticity, and recovery from injury. pia mater is attached at the CNS surface. End feet are prominent Neuroglia are endowed with a rich assortment of ionic chan- in the subependymal glial layer, and they form septa in the spinal nels, neurotransmitter receptors, and transport mechanisms that cord. End feet cover vessels within the brain and spinal cord, and enable the gliocytes to respond to many of the same signals acting they are believed to be responsible for inducing formation of tight on neurons and also to modulate the neuronal response. In parallel junctions between capillary endothelial cells (a basis for the blood- to the serial flow of information along chains of neurons, glia com- brain barrier further described on page 115). municate with other glial cells through intracellular waves of cal- Astrocytes provide structural support through their glial cium and via intercellular diffusion of chemical messengers. By fibrils, but in addition, these cells play a critical role in several releasing neurotransmitters and other extracellular signaling mol- aspects of brain function. By storing glycogen and releasing glu- ecules, glia can affect neuronal excitability and synaptic transmis- cose, they represent a source of reserve energy. Astrocyte plasma sion and perhaps coordinate activity across networks of neurons. membranes have ionic pumps that regulate K+ throughout the Moreover, glia secrete cytokines, growth factors, and other trophic narrow extracellular space of the CNS. Astrocyte processes insu- factors that dictate long- and short-term survival of neurons as well late synapses and release substances that modulate synaptic sen- as the elaboration and retraction of synaptic connections. sitivity. Astrocytes can also take up neurotransmitter molecules From a histologic perspective, neuroglial cells are relatively from the synaptic cleft to stop ongoing synaptic activity. small. With routine histologic stains, only their nuclei and cell Recent data have revealed that astrocyte activity is probably bodies are evident, and thus in tissue sections they are distin- determined by neuronal activity and that astrocytes have the ca- guished primarily by the size and shape of their cell bodies and pacity to signal not only to each other, but also back to the neu- the size and chromatin pattern of their nuclei. However, the use rons. Furthermore, since astrocytes can sense synaptic activity, it of modern immunocytochemical staining for specific cell surface is thought that they are pivotal intermediaries between neurons or internal markers allows definitive identification of glial cells and the brain microcirculation. In this regard, increased synap- in brain sections. It should be noted that unlike mature neurons, tic activity triggers calcium waves in astrocytes, causing their glial cells remain capable of mitosis, and thus they can give rise end feet to release substances that cause local vasodilation. Thus, to tumors of the nervous system. astrocytes can act to couple brain activity not only to energy de- Except for microglial cells, which migrate into the CNS mands but also to blood flow requirements. from mesoderm, CNS gliocytes are derived from the ectodermal Finally, astrocytes seem to have an immune function; they cells that form the embryonic neural tube. Neurolemmocytes of can present antigens to T lymphocytes and can secrete a wide the PNS are derived from the embryonic neural crest (as are neu- array of chemokines and cytokines, allowing them to influence rons that have cell bodies in ganglia). T helper cell response and monocyte/microglia effector func- tions. It should be noted that the reaction of astrocytes follow- ing brain trauma or in certain pathologic states is characterized Central Nervous System Gliocytes by astrocyte proliferation and hypertrophy, elongation of astro- The gliocytes of the CNS include astrocytes, oligodendrocytes, cyte processes, up-regulation of various intermediate filaments, microglia, and ependymal cells. and, in cases of severe trauma or pathology, the formation of a Nervous Tissue Alvin J. Beitz and Thomas F. Fletcher 99 dense glial scar. This process is referred to as astrogliosis and the glial scar is formed by the hypertrophied processes of reactive astrocytes filling in the space that results from loss of myelin and neurons. Oligodendrocytes Oligodendrocytes have relatively few branches (Fig. 6-7). In oligodendrocytes routine stains, they are recognized by their small, spherical, densely stained nuclei. Ultrastructurally, oligodendrocyte cytoplasm is electron-dense and rich in microtubules and or- ganelles, especially rER and mitochondria. Oligodendrocytes lack gap junctions. In gray matter, oligodendrocytes serve as perineuronal satel- microglial lites. New data indicate that oligodendrocytes act as growth fac- cell tor providers. Oligodendrocytes synthesize defined growth factors and provide trophic signals to nearby neurons. They also appear to be quite sensitive to neurotrauma. In white matter, oligodendrocytes form myelin sheaths fibrous around axons, speeding action potential propagation in the CNS. astrocyte Intracellular trafficking of membrane constituents plays an essential role in the biogenesis and maintenance of myelin. The requisite proteins and lipids are transported from their sites of synthesis to myelin via intracellular carrier vesicles transported along elements of the cytoskeleton. Microglia Microglia are cells of mesodermal origin that invade the CNS when it is vascularized embryologically. They are sparse and dif- ficult to find in normal tissue. In routine stains, microglia are identified by their small, elongated, chromophilic nuclei. With protoplasmic silver impregnation, they are seen as small, elongated cells with astrocyte polar processes (Fig. 6-7). Under physiologic conditions, micro- glia synthesize and release trophic factors; however, in response to CNS injury, microglia react, proliferate, and express protec- tive or, in some situations, cytotoxic properties. Microglia activa- tion often precedes reactions of any other cell type in the brain and once activated, they transform into macrophages with antigen- presenting and phagocytic capabilities. Reactive microglia secrete cytokines, including tumor necrosis factor and IL-1β, which can affect synaptic transmission and have trophic effects on neurons. Microglia have been recognized to play crucial roles in important diseases such as viral infections, autoimmunity, and neurodegen- erative disorders. In addition to microglia, other cell types also respond to CNS damage. Hematogenous macrophages can invade the CNS. Pericytes, cells associated with CNS capillaries and believed to have contractile capability, are also thought to be phagocytic. FIGURE 6-7 Central nervous system gliocytes are drawn Although the functional role of glial scarring is not completely as they appeared following Golgi silver impregnation of understood, it has been suggested to be an attempt by the CNS monkey cerebral cortex (×700). The insert at the bottom to restore homeostasis through isolation of the damaged region. shows an astrocyte nucleus (astr) and an oligodendrocyte nucleus (oligo) as they appear in white matter stained with Ependymal Cells Luxol blue and hematoxylin. An axon (a) surrounded by a myelin vacuole and neurokeratin can be seen. (Adapted from Ependymal cells form an epithelium that lines ventricular cav- Weiss L. Cell and Tissue Biology. A Textbook of Histology. 6th Ed. ities within the brain and the central canal of the spinal cord. The Baltimore: Urban & Schwarzenberg, Inc., 1988.) cells are typically cuboidal or columnar with numerous motile 100 Dellmann’s Textbook of Veterinary Histology cilia on their apical surfaces (Fig. 6-8). Ependymal cells are in bulbs with stereocilia, are presumed to serve a receptive function. linked by zonulae adherens and gap junctions near their lumi- Also, neurons containing secretory vesicles can be found among nal borders. In the adult brain, mature ependyma is not merely ependymal cells; they are believed to release catecholamines. an inert lining but may instead regulate the transport of ions, small molecules, and water between the cerebrospinal fluid and Peripheral Nervous System Gliocytes neuropil. Ependyma serves an important barrier function that protects neural tissue from potentially harmful substances by The gliocytes of the PNS include ganglionic gliocytes and neu- mechanisms that are still incompletely understood. Ependymal rolemmocytes (Schwann cells). cells have only limited regenerative capacity and thus typically do not undergo mitotic proliferation. Tearing of the epithelium Ganglionic Gliocytes leaves discontinuities that become filled with processes of sub- Ganglionic gliocytes (satellite cells) encapsulate neuron cell ventricular astrocytes. bodies in the PNS. In cranial nerve and spinal (sensory) ganglia, Specific modified ependymal cells, called choroid plexus a tight capsule is formed around each neuron cell body (Fig. 6-9). epithelium, cover the surfaces of choroid plexus villi and pro- In autonomic ganglia (Fig. 6-10), capsules formed by ganglionic duce cerebrospinal fluid by a mechanism that involves active gliocytes are incomplete and may enclose more than one post- secretion of Na+. Modified ependymal cells are also present at cer- ganglionic cell body. Away from the cell body, ganglionic glio- tain sites (circumventricular organs) within brain ventricles. In cytes are replaced by neurolemmocytes that sheathe or myelinate both cases, the modified ependymal cells are cuboidal and have axons. microvilli instead of cilia extending into cerebrospinal fluid. Adjacent cells are linked by relatively impermeable junctions Neurolemmocytes (zonulae occludens), establishing a localized ependymal barrier Neurolemmocytes (Schwann cells) are gliocytes of the PNS that in association with a locally reduced blood-brain barrier due to sheathe and myelinate axons. Neurolemmocytes provide a pro- the presence of fenestrated capillaries. tected immediate environment for PNS neurons and are vital for Tanycytes are modified ependymal cells found in the hypo- axonal function and survival. Each neurolemmocyte is enclosed thalamic wall of the third ventricle. The luminal border of a tany- cyte has microvilli; the basal border features an elongated process that makes contact with capillaries and neurons. Tanycytes are thought to guide hypothalamic axons and to be involved in trans- port mechanisms between the ventricle and blood vessels of the hypothalamic–hypophysial portal system. Thus, tanycytes can in- fluence the release of hypothalamic hormones. At certain sites, neuronal processes extend between ependy- mal cells to contact cerebrospinal fluid. The processes, which end FIGURE 6-8 Ependymal cells (simple columnar epithelium) line the central canal of a canine spinal cord. Motile cilia projecting into FIGURE 6-9 Canine spinal ganglion. Unipolar neuron cell the lumen of the central canal are evident (arrows). Hematoxylin bodies (N) are surrounded by ganglionic gliocytes (satellite cells) and eosin stain. (arrows) within the ganglion. Triple stain. Nervous Tissue Alvin J. Beitz and Thomas F. Fletcher 101 FIGURE 6-10 Canine autonomic ganglion. Cell bodies (N) of postganglionic neurons are multipolar and have their nuclei positioned FIGURE 6-11 Drawing of the ultrastructure of a peripheral nerve eccentrically. Ganglionic gliocytes (satellite cells) (arrows) form an neurolemmocyte sheathing 22 nonmyelinated axons (A). Each axon is incomplete capsule around individual neuron cell bodies. Triple stain. enveloped by neurolemmocyte processes. Mesoaxons (M) are formed where the processes meet. A basal lamina (BL) surrounds the entire cell. (From Lentz TL. Cell Fine Structure. Philadelphia: WB Saunders, 1971.) within a basal lamina. Neurolemmocytes can proliferate and be- come phagocytic in the event of nerve damage. Every axon in the PNS is sheathed or myelinated along its electron microscopy, one can see that the myelin sheath is com- entire length by neurolemmocytes (except for the most terminal posed of multiple layers of neurolemmocyte plasma membrane branches in some cases). Because an individual neurolemmocyte and the neurolemmocyte is within a basal lamina (Fig. 6-12). is less than 1 mm in length, a tandem series of many neuro- Myelin formation in the PNS begins with a neurolemmo- lemmocytes is required to enclose the entire length of a long axon. cyte draped around a solitary axon, establishing a simple mes- In the case of small axons, an individual neurolemmocyte axon (Fig. 6-14). Induced by the axon itself, neurolemmocyte sheathes a number of axons simultaneously and the axons (nerve processes elongate, slide past one another, and proceed to pro- fibers) are considered nonmyelinated (Fig. 6-11). Each axon duce multilayered neurolemmocyte wrappings around the axon. resides in a furrow, protected by a pair of neurolemmocyte Cytoplasm is extruded from the wrappings, thereby leaving the processes so that the space surrounding the axon communicates concentric lamellae of plasma membrane that constitute the with the general interstitial space only by means of a narrow myelin sheath. gap known as the mesaxon. For PNS axons larger than 1 µm in diameter, each neurolemmocyte encloses a single axon and At high magnification, the myelin sheath exhibits a periodic- glial processes wrap around the axon to form a myelin sheath ity of concentric major dense lines separated by intraperiod (Fig. 6-12). lines. Each major dense line is formed by fusion of inner surfaces of plasma membrane as cytoplasm is extruded during myelin sheath formation. An intraperiod line is formed where the outer Myelin Sheath surfaces of adjacent plasma membranes are separated by a small The term myelin sheath (myelin) refers to wrappings of gliocyte gap. The gap is continuous with the inner mesaxon and the plasma membrane that surround an axon and insulate it to speed outer mesaxon, all of which are derived from the original sim- conduction. Myelin is formed by oligodendrocytes in the CNS ple mesaxon of early myelin sheath development. Occasionally, and neurolemmocytes in the PNS. a major dense line appears to split and contain a pocket of cyto- In the PNS, a transverse section of a myelinated fiber viewed plasm. Adjacent pockets of cytoplasm may extend throughout by light microscopy reveals an axon enclosed in a myelin sheath the thickness of the myelin sheath, thereby establishing a myelin surrounded by neurolemmocyte cytoplasm (Fig. 6-13). With incisure in the sheath. 102 Dellmann’s Textbook of Veterinary Histology BL myelinated nerve fiber A N FIGURE 6-12 Ultrastructural drawing of a transected myelinated nerve fiber from the peripheral nervous system. A neurolemmocyte has produced a myelin sheath around a single axon (A). The myelin sheath consists of multiple wrappings of neurolemmocyte plasma membrane produced by paired neurolemmocyte processes that ultimately form outer and inner mesaxons (arrowheads at 12 and 6 o’clock, respectively). The nucleus of the neurolemmocyte is labeled (N). A basal lamina (BL) surrounds the neurolemmocyte. The insert (lower right) shows the outer mesaxon and myelin sheath enlarged. Notice that stained myelin exhibits major dense lines (formed by merger along the inner surface of neurolemmocyte plasma membrane), separated by intraperiod lines (formed by apposition of outer surfaces of neurolemmocyte plasma membrane). (From Lentz TL. Cell Fine Structure. Philadelphia: WB Saunders, 1971.) FIGURE 6-13 Transverse section through myelinated fibers of a canine peripheral nerve. Myelin sheath neurokeratin presents a radial pattern in this nerve (black arrows). The white arrow points to the nucleus of a neurolemmocyte. Its cytoplasm can be seen surrounding a myelinated axon (A). Hematoxylin and eosin stain. Nervous Tissue Alvin J. Beitz and Thomas F. Fletcher 103 FIGURE 6-14 Schematic illustration of myelinated and nonmyelinated developmental relationships between axons (ax) and neurolemmocytes in cross-sectional perspective. A. Early in development, several axons are sheathed in common by a neurolemmocyte. B. After neurolemmocyte proliferation, a solitary large axon that is destined to be myelinated is sheathed by a neurolemmocyte; a mesaxon is formed where neurolemmocyte processes meet. C. Neurolemmocyte processes elongate and encircle the axon, thereby lengthening the original mesaxon. D. A myelin sheath is formed when cytoplasm is extruded from encircling neurolemmocyte processes, thereby leaving layers of plasmalemma. Cytoplasm is generally retained internal and external FIGURE 6-15 Light micrograph of a longitudinal section of a to the myelin sheath, where an inner mesaxon and an outer canine peripheral nerve. An axon passing through a myelin node mesaxon are evident. E. In the case of nonmyelinated axons, (white arrow) is evident at the center. Lipid extraction during tissue neurolemmocyte invaginations provide a separate compartment and preparation disintegrates myelin, leaving a protein residue mesaxon for each sheathed axon. Neurolemmocyte nucleus (N). (neurokeratin) that presents a foamy appearance (nk). Nuclei in the (Adapted from Copenhaver WM, Bunge RP, Bunge MB. Bailey’s section belong to fibrocytes (small black arrow) or neurolemmocytes Textbook of Histology. 16th Ed. Baltimore: Williams & Wilkins, 1971.) (large black arrows). Triple stain. are exposed to the extracellular space (Figs. 6-16 and 6-17). A longitudinal view of a myelinated nerve fiber shows myelin Internodes are shorter and nodes are wider in the CNS compared to sheath gaps at the juncture of adjacent gliocytes (Fig. 6-15). Each the PNS. A single oligodendrocyte is known to contribute inter- gap is referred to as a node (of Ranvier), and the myelin sheath nodes to as many as 50 myelinated fibers and the outer cytoplasm between nodes is called an internode. The internodal transition of the internode is restricted to a single ridge that is connected to region immediately adjacent to a node is referred to as a para- the oligodendrocyte perikaryon by a thin process. node (Fig. 6-16). At the paranode, major dense lines split and It is well established that myelin sheaths provide electrical cytoplasm is retained in processes that overlap one another as each insulation so that action potentials jump from node to node in- contacts the axon plasma membrane. Outermost cytoplasmic stead of progressing continually, as in nonmyelinated axons. The processes of adjacent neurolemmocytes make contact, thus en- jumping process, called saltatory conduction, is much faster closing the node in the PNS. A continuous basal lamina is pres- than nonmyelinated conduction, and the longer the internode is, ent external to the neurolemmocytes. At the node, the axon bulges the faster is the conduction. Internode length is proportional to slightly and exhibits subplasmalemmal electron-dense material. myelin sheath thickness, and both are proportional to axon di- In normal axons, sodium channels are present at high density ameter. However, because neurolemmocytes develop an associa- within the nodal gap, and voltage-dependent potassium channels tion with axons early in development, axons that subsequently are sequestered on the internodal side of the paranode—a region grow farther (e.g., in the limbs) have longer internodes than known as the juxtaparanode. The juxtaparanode and its associ- axons (e.g., in the head) that do not grow as far. ated potassium channels appear to stabilize nerve conduction and The recent discovery of tight junctions and adhering junc- help maintain the internodal resting potential. tions between myelin lamellae may help elucidate other roles In the CNS, where myelin sheaths are formed by oligoden- played by this compact multilamellar structure. Whereas in epi- drocytes, nodes are not covered by cytoplasmic processes and they thelial cells these junctions are formed between different cells, in 104 Dellmann’s Textbook of Veterinary Histology ode rn te in ode CNS an a r p de no basal lamina e (reflected) nod ra on pa ax neurolemmocyte process PNS myelin FIGURE 6-16 Schematic illustration of nodal and paranodal regions of myelinated fibers from the central nervous system (CNS) (left) and the peripheral nervous system (PNS) (right). In the CNS, myelin is formed by oligodendrocytes and nodes are broadly exposed to the extracellular space. In the PNS, outer cytoplasmic processes of adjacent neurolemmocytes (Schwann cells) overlap to restrict node exposure to the extracellular space. Also, neurolemmocytes are surrounded by a continuous basal lamina. Myelin consists of compressed membranes of glial cells, distinguished by a series of major dense lines separated by intraperiod lines. At each paranode, major dense lines split to contain terminal cytoplasmic “loops” that contact the axon plasma membrane and impede ionic flow beyond the node. myelinating glia these so-called autotypic junctions are found bral canal. The term nerve fiber refers to one axon within a nerve. between membrane lamellae of the same cell. Such junctions In the case of a myelinated axon, the term nerve fiber (mye- were initially proposed to link adjacent membranes for increased linated nerve fiber) includes the axon plus the myelin sheath and mechanical strength. However, tight junctions in other cell types surrounding neurolemmocyte. play critical roles in paracellular nonbarrier function, including Individual nerve fibers may be classified as either afferent or signal transduction, and fluid movement between cells via aqueous efferent. Afferent fibers are sensory because they conduct exci- pores and channels. This knowledge, taken together with evidence tation to the CNS. Typical afferent neurons have unipolar cell from studies of normal and pathologic myelin, supports the pos- bodies located in cranial nerve and spinal (sensory) ganglia. The sibility that in addition to mechanical strength, a primary func- dendritic zone of an afferent neuron consists of receptors or post- tion of junctional plaques in myelin is to regulate perfusion of the synaptic endings on sensory epithelial cells in the case of sense periaxonal space. organs. Efferent axons arise from multipolar cell bodies located in the brain or spinal cord or in autonomic ganglia. They activate muscle or gland or neurons in autonomic ganglia. Individual nerve fibers are classified also as somatic or vis- PERIPHERAL NERVOUS TISSUE ceral. The somatic fibers innervate skin, skeletal muscles, and joints, whereas visceral fibers innervate cardiac and smooth Tissue of the PNS consists of cranial and spinal nerves, including muscles and glands. Visceral efferent fibers, in particular, (and their roots, distal branches, and ganglia. Cranial nerves originate often visceral fibers in general) are designated the autonomic from the brain and exit from the cranial cavity. Spinal nerves nervous system. The visceral efferent pathway involves two originate from the spinal cord and exit from the vertebral canal. neurons. The first (preganglionic) neuron has its cell body in A nerve root is the proximal region of a cranial or spinal nerve the CNS. The cell body of the second (postganglionic) neuron that is enveloped by meninges within the cranial cavity or verte- is located in an autonomic ganglion. Nervous Tissue Alvin J. Beitz and Thomas F. Fletcher 105 oligodendrocyte Cyt FIGURE 6-18 Diagram of peripheral nerve constituents: five fascicles constitute this peripheral nerve. The fascicles are united by a surrounding epineurium (connective tissue). Each fascicle is encircled by perineurium, which consists of layers of perineural epithelioid cells surrounded by fibrous perineurium. Within a fascicle, ES A endoneurium surrounds individual myelinated fibers. A myelinated Cyt fiber consists of an axon surrounded by a myelin sheath that is ES formed by a series of neurolemmocytes and interrupted by nodes. Somatic afferent and efferent myelinated fibers are illustrated. (Modified from Jenkins TW. Functional Mammalian Neuroanatomy. 2nd Ed. Philadelphia: Lea & Febiger, 1978.) FIGURE 6-17 Illustration of an oligodendrocyte providing myelin internodes to three axons. A bisected view of a node, sheets of the squamous cells, along with interposed collagen fi- adjacent paranode regions, and part of an internode is shown in the brils, form a continuous tube enclosing nerve fibers and endo- foreground. The axon (A) bulges at the node and is exposed to neurium within a fascicle. A perineural epithelioid tube may extracellular space (ES). Loop profiles containing cytoplasm (Cyt) have a dozen concentric layers at its origin, at the juncture of the contact the axon at the paranodal regions. Cytoplasm is retained nerve with its meningeal-covered root. The number of layers along the ridges associated with inner and outer mesaxons, and gradually decreases as a nerve branches. A single layer surrounds small cytoplasmic pockets may be seen interrupting major dense terminal branches, but epithelioid cells proliferate to encapsulate lines. (Adapted from Bunge MB, Bunge RP, Ris H. Ultrastructural study certain receptors. A blood-nerve barrier exists, constituted by of remyelination in an experimental lesion in adult cat spinal cord. the epithelioid cells and the endothelium of endoneurial micro- J Biophys Biochem Cytol 1961;10:67–94.) vessels. This barrier serves to protect axons and the internal nerve environment from fluctuations in blood plasma levels of hor- mones and ions and from toxic substances. While the perineural Nerves epithelioid tube affords nerve fibers a protected environment, A nerve typically consists of thousands of axons, each sheathed the enclosed intrafascicular space may also serve as a channel or myelinated by neurolemmocytes and all organized into fasci- for infectious or toxic agents once they invade the epithelioid cell cles enveloped by connective tissue (Fig. 6-18). A nerve fascicle barrier. is delimited by perineurium, which consists of fibrous tissue sur- The perineural epithelioid cells are squamous and arranged rounding epithelioid cells. The multiple fascicles of a nerve are in concentric sheets. The fibrous perineurium is collagenous bound together by connective tissue called epineurium. Within connective tissue. The morphologic distinction between fibrous a nerve fascicle, the fibrocytes and collagen fibers surrounding in- perineurium, which surrounds individual fascicles, and epineu- dividual neurolemmocytes constitute endoneurium. Blood ves- rium, which binds fascicles together, varies with the species. In the sels supplying a nerve are designated vasa nervorum. dog, for example, nerves are composed of a few relatively large fas- Individual perineural epithelioid cells are joined by zonulae cicles and fibrous perineurium is dense compared to epineurium, occludens and enveloped by basal laminae. Multiple concentric which is a flimsy, fatty connective tissue. In contrast, fibrous peri- 106 Dellmann’s Textbook of Veterinary Histology neurium and epineurium tend to blend indistinguishably in rons. The muscle fibers are readily fatigued and, because large bovine nerves, which feature multiple small fascicles. neurons require additional synaptic input to reach threshold, they fire only when strong muscle contractions are needed. A neuromuscular synapse consists of a presynaptic neu- Ganglia ronal end plate overlaying a postsynaptic muscle sole plate at A ganglion is a localized enlargement of a nerve produced by the the midregion of a muscle fiber (Fig. 6-19). A motor end plate accumulation of neuron cell bodies. Spinal ganglia located in is formed by very short branches within a circumscribed zone dorsal spinal roots and ganglia in cranial nerve roots are referred (plate) at the end of one terminal branch of an efferent neuron. to as sensory ganglia, because they contain cell bodies of pri- Each branch of the end plate lies in a corresponding trough of mary afferent neurons (Fig. 6-9). Afferent neurons are unipolar, the sole plate. The width of the neuromuscular gap is 40 to except in the case of sense organs (vestibular apparatus, cochlea, 50 nm; however, the gap is increased by junctional folds, where retina, and olfactory epithelium), in which afferent neurons are sarcolemma of the trough undergoes transverse enfolding. Neuro- bipolar. lemmocytes cover the end plate, and associated basal lamina Within a sensory ganglion, unipolar cell bodies are dis- extends into the neuromuscular gap and junctional folds tributed superficially and nerve fibers course through the center (Fig. 6-20). of the ganglion. These unipolar cell bodies range in size from End-plate cytoplasm contains many mitochondria and nu- large (30 to 50 µm in diameter) to small (15 to 25 µm in diam- merous agranular synaptic vesicles (40 nm in diameter). The vesi- eter), and each cell body gives rise to a single axon that may coil cles contain acetylcholine, which is released at active sites opposite initially before bifurcating into central and peripheral branches; the junctional folds. Acetylcholine molecules diffuse across the the peripheral branch is usually thicker than the central branch. neuromuscular gap and bind to postsynaptic receptor sites that Large cell bodies give rise to myelinated axons that bifurcate at open cation channels, leading to muscle fiber depolarization. a node. Each cell body is tightly encapsulated by ganglionic Some binding sites are cholinesterase enzymes that degrade gliocytes. acetylcholine and thereby stop synaptic activity. Transporter pro- Autonomic ganglia are accumulations of multipolar cell tein molecules are present in the presynaptic membrane to recap- bodies within autonomic nerves (Fig. 6-10). The neuron cell bod- ture choline and recycle it. ies, which have eccentric nuclei and marginally distributed chro- The fusimotor or motor neuron is another type of matophilic substance as normal features, are loosely encapsulated somatic efferent neuron. It innervates intrafusal muscle fibers by ganglionic gliocytes. Synapses occur in autonomic ganglia, within muscle spindles. These relatively small neurons have where terminals of cholinergic preganglionic neurons synapse on myelinated axons that terminate as end plates or trail endings dendritic zones of postganglionic neurons. Microscopic accumu- (multiple synaptic contacts along the muscle fiber surface). lations of postganglionic cell bodies within nerve plexuses of vis- Preganglionic autonomic neurons originate in the CNS ceral organs (especially the gut) constitute terminal autonomic and synapse on postganglionic neurons in autonomic ganglia, ganglia. making typical interneuronal synapses. Postganglionic auto- Postganglionic neurons are classified either as cholinergic nomic neurons have nonmyelinated axons that innervate car- if they synthesize and release acetylcholine or as adrenergic if diac muscle, smooth muscle, or glands. The postganglionic their associated neurotransmitter is noradrenaline (norepineph- axons terminate in branches that individually wander long dis- rine). Adrenergic neurons feature dense-core synaptic vesicles. tances without evidence of specialized contact with muscle or Some autonomic ganglia contain a few small intensely fluores- gland cells. cent (SIF) cells. These SIF cells feature numerous large, dense- Ultrastructurally, terminal autonomic nerves consist of iso- core vesicles containing dopamine, which is released under neural lated neurolemmocytes sheathing one or more terminal branches. control. The significance of SIF cells is unknown, but they The terminal branches have numerous preterminal synaptic vari- form somatodendritic synapses and thus appear to function as cosities along their course. Each preterminal varicosity bulges be- interneurons. yond the confines of the sheathing neurolemmocyte and contains a concentration of synaptic vesicles. Thus, neurotransmitter is re- leased from multiple sites and diffuses variable distances to bind Efferent Neurons with receptors on target cells. Postsynaptic specializations are not Somatic efferent neurons (α motor neurons) innervate skeletal evident. muscle. One such neuron, together with all the muscle fibers it There are several lines of evidence indicating that postgan- innervates, is regarded as a motor unit, because the muscle fibers glionic sympathetic axons innervating immune organs, such as contract as a unit when the neuron is excited. A motor unit may the spleen and lymph nodes (see Chapter 8), can regulate cytokine have few or several hundred muscle fibers, depending on muscle production by immune cells in these organs. This occurs via the size. Typical muscles have a range of large and small motor units. release of noradrenaline from nonsynaptic axonal varicosities, re- Small motor units have fatigue-resistant muscle fibers inner- sulting in the suppression of immune responses. Conversely, im- vated by small neurons that are the first to begin firing and the mune cells can release neurotrophic factors, such as nerve growth last to cease firing during muscular contraction. Large motor factor and brain-derived neurotrophic factor, that act on appro- units have hundreds of muscle fibers innervated by large neu- priate receptors present on neurons and astrocytes and that have Nervous Tissue Alvin J. Beitz and Thomas F. Fletcher 107 FIGURE 6-19 Drawing of three motor end plates synapsing on skeletal muscle fibers. Each of three terminal branches of an axon ends in a perfusion of short branches collectively designated an end plate. The bottom end plate is viewed from its edge. Neurolemmocytes that ensheathe axon terminal branches proceed to overlay the end plate branches. Neurolemmocyte nucleus (N. nuc.); muscle fiber nucleus (M. nuc.). (Adapted from Krstić RV. General Histology of the Mammal. New York: Springer-Verlag, 1985.) FIGURE 6-20 Ultrastructural drawing of one nerve ending (NE) of a branched motor end plate synapsing on a muscle fiber (MF). The nerve ending contains numerous synaptic vesicles (SV). The muscle fiber plasma membrane features junctional folds (JF) that greatly expand the synaptic cleft. The cleft contains basal lamina (BL) continuous with that covering the muscle fiber and the neurolemmocyte (N) overlaying the nerve ending. (From Lentz TL. Cell Fine Structure. Philadelphia: WB Saunders, 1971.) 108 Dellmann’s Textbook of Veterinary Histology neuroprotective effects. This highlights the fact that the nervous Nonencapsulated Receptors and immune systems are closely interlinked such that physiologic Free nerve endings are found throughout the body. They de- or pathologic effects on one system may affect the other. tect stimulation described as pain (noxious), warmth, cold, or touch. Simultaneously, the same stimulus information is used for Receptors subconscious reflex activity. The receptors are associated with nonmyelinated or thinly myelinated axons that branch exten- Afferent axons convey information to the CNS from receptors or sively to innervate a wide area (receptive field). The actual re- from sense organs. Sense organs are organized collections of sen- ceptors are simply unsheathed terminal branches enveloped by sory epithelial cells, neurons, and supporting cells that detect vi- basal lamina (Fig. 6-21). sual, auditory, olfactory, or taste stimuli. In contrast, receptors Hair follicle terminals, which detect body hairs being dis- are individual, isolated stimulus detectors that are widely dis- placed, are derived from myelinated axons that branch exten- tributed in the body (Fig. 6-21). Generally, the peripheral branch sively to innervate hundreds of follicles. Each follicle is encircled of an afferent axon branches repeatedly, and a receptor is located by a nonmyelinated plexus that disperses free nerve endings at the termination of each branch. All receptors of a single neuron among follicle epithelial cells. (A different situation prevails for have the same structure and function. tactile hair follicles [vibrissae], each of which receives several Receptors are classified in several ways. By location, extero- myelinated axons, giving rise to several kinds of receptors.) ceptors, proprioceptors, and enteroceptors are found at the Nonencapsulated tactile corpuscles are often collected at body surfaces, in musculoskeletal structures, and in viscera, respec- the base of a slight skin elevation called a tactile pad (Fig. 6-21). tively. Receptors are classified according to the type of stimulus Each tactile corpuscle consists of an expanded axonal terminal they are sensitive to as mechanoreceptors, chemoreceptors, and branch that is embraced by processes of an epithelioid tactile cell thermoreceptors. Morphologically, receptors may be classified as (Merkel cell). The epithelioid cell, which develops under the encapsulated and nonencapsulated. The following is a list of trophic influence of the nerve ending, contains large dense core common receptors. vesicles. Individual tactile corpuscles are innervated by a mye- linated axon that distributes to a restricted receptive field. The tactile corpuscles are capable of persistent firing (tonic receptors). Encapsulated Receptors Encapsulated tactile corpuscles (Meissner’s corpuscles) are oval, encapsulated phasic mechanoreceptors for touch and super- ficial pressure, found in the dermal papillae of mammalian skin (Fig. 6-21). Several myelinated axons give rise to nonmyelinated branches that permeate a stack of flattened neurolemmocytes en- capsulated by perineural epithelioid cells. Tactile corpuscles and their associated afferent nerve fibers adapt rapidly and provide great sensitivity to minute skin deformation. Lamellar corpuscles (Vater’s corpuscles, Pacini’s corpus- cles) are widely distributed throughout the body and are large enough to be seen without magnification (0.5 × 1.0 mm). A ter- minal branch of a myelinated axon is encased in several layers of flattened neurolemmocytes that are surrounded by a fluid space and multiple concentric layers derived from perineural epithelioid FIGURE 6-21 Schematic drawings of five receptors at various cells (Fig. 6-21). The layered lamellae of these corpuscles function magnifications. Free nerve endings branch among cells of the as an extremely selective high-pass filter, which serves to dampen epidermis. Two nonencapsulated tactile corpuscles are shown at the the large, dynamic stresses and strains applied to the skin, allow- base of the epidermis; each corpuscle consists of a terminal branch ing these receptors to be extremely sensitive to transient pressure, making contact with a specialized tactile cell (Merkel’s cell). An such as vibratory stimuli. Thus, these lamellar corpuscles are able encapsulated tactile corpuscle (Meissner’s corpuscle) is located in the to provide a neural image of vibrations transmitted to the skin. dermis; dendritic branches from multiple myelinated axons weave Bulbous corpuscles (Krause’s corpuscles, Golgi–Mazzoni among flattened neurolemmocytes within a capsule of perineural corpuscles, genital corpuscles) vary in location, size, and shape. epithelioid cells. A lamellar corpuscle (Pacini’s corpuscle) features the They are mechanoreceptors derived from myelinated axons that dendritic branch of a myelinated axon encased in a core of flattened neurolemmocytes within a laminated capsule composed of perineural have highly coiled terminal branches enclosed in a relatively thin epithelioid cells. A neurotendinous spindle splays among the collagen capsule derived from perineural epithelioid cells. bundles of a small tendon; the receptor has a thin capsule and is Neurotendinous spindles (Golgi tendon organs) are lo- associated with a myelinated axon (not shown). (Encapsulated tactile cated at muscle–tendon junctions and are activated by tension. corpuscle and neurotendinous spindle from Krstić RV. General Histology Derived from a large myelinated axon, the receptor consists of of the Mammal. New York: Springer-Verlag, 1985.) terminal branches traveling on bundles of collagen fibers within Nervous Tissue Alvin J. Beitz and Thomas F. Fletcher 109 a fluid-filled, thin capsule derived from perineural epithelioid Two types of receptors are found on intrafusal muscle fibers cells (Fig. 6-21). Ruffini’s corpuscle, a tonic mechanoreceptor (Fig. 6-22). A primary ending, also called an annulospiral found in dermis, fascia, and ligaments, is structurally similar to ending, is derived from a single, large myelinated axon that has the neurotendinous spindle. terminal branches that spiral around the nuclear regions of in- Neuromuscular spindles (muscle spindles) are so elabo- trafusal muscle fibers. A secondary ending is derived from a rate that they could qualify as sense organs. Located in most myelinated axon with dendritic branches arranged in a flower- skeletal muscles, the spindle has an elongated (1.5 mm) capsule spray configuration and situated on nuclear chain fibers adjacent derived from perineural epithelioid cells. The capsule encloses af- to the annulospiral endings. Collectively, the receptors are acti- ferent and efferent innervation as well as two kinds of intrafusal vated by the rate and degree of stretch that occurs when either muscle fibers, designated nuclear bag and nuclear chain fibers the polar ends of intrafusal muscle fibers contract or the whole (Fig. 6-22). A typical spindle has one or two nuclear bag fibers, muscle is stretched. Information from spindle receptors is pri- each with a dilated middle zone filled with nuclei and striated marily subconscious and