Fundamentals of Biochemistry - BCHE2030 Lecture Notes PDF
Document Details
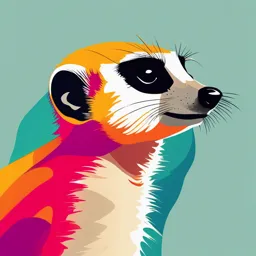
Uploaded by TenderGoshenite6897
The Chinese University of Hong Kong
Shannon Au
Tags
Summary
These are lecture notes on Fundamentals of Biochemistry (BCHE2030). The notes cover topics such as thermodynamics, bioenergetics, and energy relationships between catabolic and anabolic pathways. They are suitable for undergraduate students.
Full Transcript
BCHE2030 Fundamentals of Biochemistry Thermodynamics & Bioenergetics Shannon Au Room 178, Science Center School of Life Sciences Chinese University of Hong Kong e-mail: [email protected] Energy relationships between catabolic and anabolic pathways Living cells are comp...
BCHE2030 Fundamentals of Biochemistry Thermodynamics & Bioenergetics Shannon Au Room 178, Science Center School of Life Sciences Chinese University of Hong Kong e-mail: [email protected] Energy relationships between catabolic and anabolic pathways Living cells are composed of a complex and intricately regulated system Ø Anabolism: biosynthetic phase; require energy Ø Catabolism: degradative phase; release energy Catabolic pathways deliver chemical energy in the form of ATP and reduced electron carriers (NADH, NADPH and FADH2). These energy carriers are used in anabolic pathways. Thermodynamics and Bioenergetics Thermodynamics The study of energy and its effect on matter Allows us to investigate the distribution of energy in a system Bioenergetics Quantitative study of energy transfer within a biological system One goal of biochemistry is to understand, in quantitative and chemical terms, the means by which energy is extracted, stored, and channeled into useful work in living cells. We can consider cellular energy conversions —like all other energy conversions—in the context of the laws of thermodynamics. Topic outline Laws of thermodynamics and concepts of free energy Concepts of high energy compounds and coupled reactions Flow of cellular energy - oxidation-reduction reactions Energetics of biological electron transfers Laws of thermodynamics & concepts of free energy Learning Objectives: Describe the 1st and 2nd laws of thermodynamics Understand the relationship between free energy, enthalpy and entropy Understand the concept of spontaneity for a biological process Understand the relationship between equilibrium constant and free energy Laws of thermodynamics & concepts of free energy Key readings: Chapter 13, Section 13.1 Bioenergetics and Thermodynamics Lehninger Principles of Biochemistry, 7th Edition Chapter 1, Section 1.3 Laws of thermodynamics govern the behaviour of biochemical reaction Chapter 8, Section 8.2 Free energy is a useful thermodynamic function for understanding enzymes Biochemistry, Berg, Tymoczko & Stryer 7th Edition Supplementary readings: Chapter 1, Section 1.3 Physical Foundations Lehninger Principles of Biochemistry, 7th Edition Living organisms exist in a dynamic steady state, never at equilibrium with their surroundings First laws of thermodynamics Conservation of energy In any physical or chemical change, the total amount of energy in the universe remains constant, although the form of the energy may change. While energy is “used” by a system, it is not “used up”; rather, it is converted from one form into another Second law of thermodynamics The universe always tends toward increasing disorder: in all natural processes, the entropy of the universe increases Entropy S : a measure of the degree of randomness C6H12O6 + 6 O2 à 6 CO2 + 6 H2O Free-energy change ∆G When a chemical reaction occurs at a constant temperature, the free energy change can be determined as: DG = DH - T DS H: enthalpy change; reflecting the number and kinds of bonds and non-covalent interactions broken and formed S: entropy change T: absolute temperature (in Kelvin) When DG = negative, reaction proceeds forward When DG = positive, reaction proceeds in reverse When DG = 0, at equilibrium Free-energy change ∆G Exergonic reaction: proceeds with a net release of free energy; occurs spontaneously Endergonic reaction: absorbs free energy from its surroundings, occurs non-spontaneously (a) Exergonic reaction Reactants Amount of energy released (DG < 0) Free energy Energy Products Progress of the reaction Energy coupling links reactions Coupling of an exergonic reaction to an endergonic reaction through a shared intermediate Chemical example Mechanical example Biochemical reactions as equilibrium a, b, c, d : number of molecules of A, B, C, and D [A]eq, [B]eq, [C]eq, [D]eq are the molar concentrations of A, B, C and D at the point of equilibrium Keq > 1, more products than reactants are formed Keq < 1, more reactants than products are formed Standard free-energy change and its relationship with equilibrium constant Standard free-energy change, DGo - under standard conditions (298K=25oC), reactants and products are initially present at 1 M For reactions involve hydrogen ions, if [H+] = 1 M, what is the pH of the system? For biochemists, we have a different standard state, - 298K=25oC, [H+] = 10 -7 M (pH 7), [water]= 55.5 M, all other reactants at 1 M, (for reactions involving Mg2+, [Mg2+] = 1 mM) DG’o = - RT In K’eq R = gas constant = 8.314 J / mol. K T = absolute temperature in Kelvin Relationship between ∆G’o and K’eq is exponential DG’o = - RT In K’eq Example I DG’o = - RT In K’eq DG’o = -7.3 kJ / mol -DG’o 7300 RT 8.314 x 298 K’eq = e =e = 19 Actual free-energy changes depend on [reactant] and [product] Each chemical reaction has a characteristic DG’o. It tells in which direction and how far a given reaction must go to reach equilibrium at standard condition. Actual free-energy change, DG of a chemical reaction is a function of [reactant] and [product] (which are not necessarily match the standard condition). A+B C+D [C] [D] DG = DG’o + RT ln [A] [B] ( Mass-action ratio Q) Example II DG = DG’o + RT ln Q [G1P] = 0.01 M, [G6P] = 1 M, Q = 100 DG’o = -7.3 kJ / mol DG = -7300 + RT ln 100 = 4.1 kJ/mol Reaction will proceed backward, i.e. conversion of G6P to G1P Actual free-energy changes depend on [reactant] and [product] D G = D G’o + RT ln [products] / [reactants] = D G’o + RT lnQ When DG is large and negative, the reaction tends to go in the forward direction When DG is large and positive, the reaction tends to go in the reverse direction When DG = 0 , the system is at equilibrium Standard free-energy changes are additives Standard free-energy changes are additives, but equilibrium constants are multiplicative Thermodynamics allows us: To predict whether the process can occur (spontaneity) However, it cannot tell us: How a reaction occurs Rate of the reaction Topic outline Laws of thermodynamics and concepts of free energy Concepts of high energy compounds and coupled reactions Flow of cellular energy - oxidation-reduction reactions Energetics of biological electron transfers Concepts of high energy compounds and coupled reactions Learning Objectives: Describe the coupling to ATP with endergonic reactions Understand the chemical logic of the ATP hydrolysis Identify high- and low-energy compounds and their importance Concepts of high energy compounds and coupled reactions Key readings: Chapter 13, Section 13.3 Phosphoryl group transfers and ATP Lehninger Principles of Biochemistry, 7th Edition Chapter 15 Section 15.2 ATP is the universal currency of free energy in biological systems Biochemistry, Berg, Tymoczko & Stryer 7th Edition High energy phosphate bonds Phosphate anhydride bond The repulsion between the negatively charged phosphates causes the phosphate anhydride bond to behave like a ‘coiled spring’. When the bond is broken, the phosphates separate rapidly, and energy is released. Chemical basis of large and negative DG’o for ATP hydrolysis 1) Charge separation results from hydrolysis relieves electrostatic repulsion among the four negative charges on ATP 2) Product Pi is stabilized by formation of resonance forms 3) Product ADP2- immediately ionizes, releasing H+ 4) Better hydration of ADP and Pi Actual free energy change DG in ATP hydrolysis in living cells is very different Mg2+ in the cytosol binds to ATP and ADP, the true substrate of hydrolysis is MgATP2- [ATP] , [ADP], [Pi] are −25 kJ/mol Is ATP a high-energy or low-energy compound? Ranking of biological phosphate compounds Flow of phosphoryl groups (P) from high-energy phosphoryl group donors via ATP to acceptor molecules to form their low-energy phosphate derivatives. This flow of phosphoryl groups, catalyzed by kinases, proceeds with an overall loss of free energy under intracellular conditions. Hydrolysis of low-energy phosphate compounds releases Pi, which has a very lower phosphoryl group transfer potential. Phosphorylated compounds can be synthesized by coupling to the hydrolysis of another phosphorylated compounds with a more negative DG’o Energy provided by ATP By group transfer Part of the ATP (a phosphoryl group) is first transferred to a substrate, becoming covalently attached to the substrate and raising its free energy content Then the phosphoryl group is displaced generating Pi By direct hydrolysis of terminal phosphoanhydride bond Non-covalent binding of ATP e.g. DNA helicase Source of ATP during exercise Phosphocreatine (or called creatine phosphate) At rest, muscle contains only enough ATP to sustain contractile activity for less than a second Phosphocreatine serves as a reservoir of high-potential phosphoryl groups in muscle. Source of ATP during exercise Phosphocreatine - contains a P-N bond anhydride Topic outline Laws of thermodynamics and concepts of free energy Concepts of high energy compounds and coupled reactions Flow of cellular energy - oxidation-reduction reactions Energetics of biological electron transfers Flow of cellular energy and electron transfer Learning Objectives: Understand the concept of two half-reactions in oxidation- reduction reactions Describe the relationship between standard reduction potential and standard free energy Describe the importance of NAD and NADP as electron carriers Flow of cellular energy and electron transfer Key readings: Chapter 13, Section 13.4 Biological oxidation-reduction reactions Lehninger Principles of Biochemistry, 7th Edition Chapter 18 Section 18.2 Oxidative phosphorylation depends on electron transfer Biochemistry, Berg, Tymoczko & Stryer 7th Edition Central features of metabolism Transfer of phosphoryl groups Transfer of electron in oxidation-reduction reaction (electron donor à electron acceptor) Where are the sources of electrons? Oxidation – Reduction Oxidation of ferrous ion by cupric ion Reducing agent (reductant): e- donating Oxidizing agent (oxidant): e- accepting Electron donor e- + electron acceptor Fe2+ and Fe3+ : conjugate redox pair Biological oxidations often involve dehydrogenation Unequal sharing of an electron between C and another atom Order of increasing electronegativity H < C < S < N < O With each loss of electrons, the carbon atom has undergone oxidation (even no oxygen is involved). Alkane (Ethane) Alkene (Ethene) no. of e- owned by Different oxidation states of carbon the red carbon atom More reduced More oxidized Standard reduction potential, E’o measures affinity for electrons Tendency for electron transfer from e- donor of one redox pair to e- acceptor of the other redox pair depends on the relative affinity of the electron acceptor of each redox pair for electrons Reference cell: Standard hydrogen electrode, pH=0 2H+ + 2 e- H2 Test cell: any redox couple, concentrations of oxidant and reductant = 1 M Strongly reducing couples donate e- : Eo very negative Strongly oxidizing couples accept e- : Eo very positive Which has the strongest electron affinity? Standard reduction potential, E’o measures affinity for electrons Positive value of E’o : stronger tendency to acquire electrons DE’o = 0.123V Relationship between DE’o and DG’o No. of electrons Faraday constant = 96.5kJ/V. mol transferred in the reaction e.g. D E’o = 0.123V DG’o = -2 (96.5 kJ/V. mol) (0.123V) = -23.7 kJ/mol under a condition of pH = 7.0, [acetaldehyde], [ethanol], [NAD+] and [NADH] are all present at 1M Relationship between DE’o and DG’o If [acetaldehyde] and [NADH] = 1 M, [ethanol] and [NAD+] = 0.1M, what is the DG? DG = -23.7 kJ/mol + (8.315 J/mol.K) (298 K) ln [(0.1)(0.1) / (1)(1)] = -35 kJ / mol NADH and NADPH as universal e- carriers NADH (reduced) Nicotinamide adenine dinucleotide (NAD+) undergo reduction to NADH, accepting a hydride ion (two electrons and one proton) from an oxidizable substrate. The hydride ion is added to the nicotinamide ring. NADH and NADPH as universal e- carriers In many cells, [NAD]>>[NADH], favoring the hydride transfer from a substrate to NAD to form NADH. However, [NADPH]>> [NADP], favoring the hydride transfer from NADPH to a substrate NAD function in oxidations (catabolism) NADPH function in reductions (anabolism) Dietary deficiency of niacin, the vitamin form of NAD and NADP causes pellagra The nicotinamide ring of NAD and NADP are derived from the vitamin niacin (Vit B3) which is synthesized from tryptophan. Humans cannot synthesize niacin in sufficient quantities. Niacin deficiency, which affects all the NAD(P)-dependent dehydrogenases, causes pellagra (糙皮病) - characterized by dermatitis, diarrhea, and dementia. In early 1900s, in the southern US, where maize/corn was a major diet, about 10,000 died.