Document Details
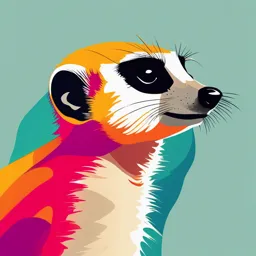
Uploaded by EffectualJubilation
University of Missouri, Columbia
Full Transcript
UNIT 10 Chapter 51: The Eye: II. Receptor and Neural Function of the Retina Slides by Thomas H. Adair, PhD Copyright © 2021 by Saunders, an imprint of Elsevier Inc. Retina • Light sensitive portion of eye. • Contains cones for color vision. • Contains rods for night vision. • Contains neural arc...
UNIT 10 Chapter 51: The Eye: II. Receptor and Neural Function of the Retina Slides by Thomas H. Adair, PhD Copyright © 2021 by Saunders, an imprint of Elsevier Inc. Retina • Light sensitive portion of eye. • Contains cones for color vision. • Contains rods for night vision. • Contains neural architecture. • Light must pass through neural elements to activate light sensitive rods and cones. But this is not so bad since neural elements are virtually transparent in vivo. • Each retina has 100 million rods; 3 million cones; and 1.6 million ganglion cells. TMP14, Figure 51-1 The Fovea • It’s a small area at center of retina ~1 mm2. • center of fovea, called “central fovea” or “fovea centralis” contains only cones. • these cones have special structure. • aid in detecting detail. • In central fovea, neuronal cells and blood vessels are displaced to each side so light can strike cones with less obstruction. • This is area of greatest visual acuity. • At central fovea: no rods, and ratio of cones to ganglion cells is 1:1; this explains high degree of visual acuity in central retina. Figure 51-2 Figure 51-2 Distribution of rods and cones Structure of Rods and Cones Pigment layer Guyton, Figure 51-4 Guyton, Figure 51-3 Rods and Cones RODS • high sensitivity; specialized for night vision (scotopic vision) • more photopigment • high amplification; single photon detection • saturate in daylight • slow response, long integration time • more sensitive to scattered light • low acuity; highly convergent retinal pathways, not present in central fovea • Achromatic; one type of rod pigment (rhodopsin) •More common in the periphery CONES • lower sensitivity; specialized for day vision (photopic vision) • less photopigment • less amplification • saturate only with intense light • fast response, short integration time • more sensitive to direct axial rays • high acuity; less convergent retinal pathways, concentrated in central fovea • Trichromatic; three types of cones, each with a different pigment (photopsin) that is sensitive to a different part of visible spectrum • More common in the fovea and macula Pigment Layer of Retina • • • • • Contains black pigment called melanin. Prevents light reflection in globe of eye. Without pigment, light would scatter diffusely; normal contrast between dark and light would be diminished. Albinos lack pigment layer – poor visual acuity because of scattering of light. – Even with corrective lenses, vision is rarely better than 20/200. Pigment epithelium/choroid contains high levels of vitamin A (needed for phototransduction). TMP14, Figure 51-1 Visual Phototransduction Rhodopsin resides in disk membrane of outer segment of rod and encloses light sensitive 11cis retinal molecule. Absorption of a photon of light by retinal leads to a change in configuration from 11-cis to all-trans retinal. The retinal then breaks away from rhodopsin, causing rhodopsin to be activated. The activated rhodopsin (aka, Metarhodopsin II) then begins the 2nd messenger cascade which leads to Conversion of light into electrical signals ROD Rhodopsin 11-cis retinal light 11-cis retinal All-trans retinal • Occurs in rods, cones and photosensitive ganglion cells. • The pigment protein in rods is called rhodopsin; • The pigment protein in cones is called photopsin, a close analog of rhodopsin (there are 3 types of photopsin: types I (red), II (green), and III) (blue). • The pigment portion of photosensitive retinal ganglion cells is called melanopsin. • The transduction mechanism is similar for • The rods, cones, and probably light sensitive all-trans retinal is then reduced ganglion cells. retinol, which then to all-trans travels back to the retinal pigment epithelium layer to be “recharged” to become 11-cis retinal. • The 11-cis retinal travels back to the rod where it is conjugated to an opsin to form new rhodopsin or a new photopsin. Vitamin A1 is required for Phototransduction Vitamin A1 (aka, all-trans retinal) is converted into 11-cis retinal within the retinal pigment epithelium. Food source: two types found in diet. • Preformed vitamin A: animal products such as meat, fish, poultry and dairy foods. • Pro-vitamin A: plant-based foods such as fruits and vegetables. The most common type of provitamin A is beta-carotene. Pro-vitamin A is a Vitamin A deficiency precursor of vitamin A. • the leading cause of preventable childhood blindness worldwide in developing countries • Prolonged and severe vitamin A deficiency can produce total and irreversible blindness. • ~250,000–500,000 children become blind each year (with the highest prevalence in Southeast Asia and Africa). (nyctalopia): Lack of vitamin • Night blindness A1 causes a decrease in retinal, which results in decreased production of rhodopsin; and a lower sensitivity of retina to light. Night blindness can occur in patients with GI absorption problem, e.g., celiac disease, cholestasis. Why? Br J Ophthalmol. 2006 Aug; 90(8): 955–956. http://medical-dictionary.thefreedictionary.com/vitamin+A+deficiency TMP14, Figure 51-5 Rod Receptor Potential • • • • • Normally about -40 mV (in dark). Normally, outer segment of rod is permeable to Na+ and Ca++ ions. Dark In the dark, an inward current (the current dark current) carried by Na+ and Ca + + ions flows into outer segment of rod. An outward current carried by K+ ions occurs in inner segment of rod. When rhodopsin decomposes, it causes hyperpolarization of rod by decreasing Na+ and Ca++ permeability of outer segment. Greater amounts of light produce So, photoreceptor cells greater electronegativity. depolarize in scotopic conditions (low light or no Ca light) and hyperpolarize in photopic conditions (well-lit conditions). Na+ Ca++ TMP14, Figure 51-6 -40 mV -70 mV ++ Ca++ Phototransduction – Closure of cGMP-gated Na+ and Ca++ channels with subsequent hyperpolarization (1) Light activated rhodopsin (metarhodopsin II) activates transducin. (2) Transducin activates cGMP phospho- diesterase, which destroys cGMP. (3) cGMP levels decrease, (4) causing sodium channels to close. (5) Closure of sodium channels causes photoreceptors to hyperpolarize Metarhodopsin II is deactivated rapidly after activating transducin by rhodopsin kinase and arrestin. 1 2 4 3 5. -40 mV mV →-70 Na+, Ca++ channel TMP14, Figure 51-7 Duration and Sensitivity of Receptor Potential • Rods: a single pulse of light activates receptor potential for more than 1 s. • Cones: occurs 4 X faster. • Receptor potential is proportional to logarithm of light intensity. Information within the neural elements of the retina is conveyed by electrotonic potentials, not action potentials. – very important for discrimination of light intensity. Color Vision • Color vision results from activation of cones. • The pigment protein in cones is called photopsin, a close analog of rhodopsin (there are 3 types of photopsin: types I (red), II (green), and III) (blue). • The retinal portion of the photopsins is the same as in rods. • Each cone is receptive to a particular wavelength of light. Figure 51-8 Color Blindness • • • • • Lack of a particular type of cone. Genetic disorder mostly passed along on X chromosome. Hence, occurs almost exclusively in males. Blue color blindness affects both men and women equally, because it is carried on a non-sex chromosome. Most color blindness results from lack of red or green cones. – lack of a red cone, protanope – lack of a green cone, deuteranope – Lack of a blue cone, tritanopia What happens in hereditary color deficiency? – Red or green cone peak sensitivity is shifted. – Red or green cones absent. Normal cone sensitivity curves (TRICHROMAT) 437 nm B 533 nm 564 nm G R Deuteranomaly (green shifted toward red) 5% of Males 437 nm B 564 nm G R Deutan Dichromat (no green cones; only red and blue) 437 nm 1% of Males (there is no green curve) B 564 nm R Deuteranope Vision Normal Color Deuteranope, shades of orange, no yellow or green Protanomalous (red shifted toward green) 1% of Males 533 nm 437 nm B G R Protan Dichromat (no red cones; only green and blue) 1% of Males (there is no red curve) 533 nm 437 nm B G Protanomaly Normal Protanomaly Protanope Vision Normal Vision Protanope, red-green colors are difficult to distinguish Neural Organization of Retina Direction of light Figure 51-12 Signal Transmission in the Retina • • • Neural elements in retina use electrotonic potentials (aka, graded potentials) to move current along their membranes instead of action potentials (exceptions include ganglion cells and some of the amacrine cells). The electrotonic potentials allow graded conduction of signal strength proportional to light intensity. Ganglion cells have action potentials. – send signals to brain via optic nerve (CN 2). – spontaneously active with continuous action potentials. – visual signals are superimposed on this background. – many excited by changes in light intensity. – respond to contrast borders, this is the way the pattern of the scene is transmitted to the brain. Figure 51-12 Lateral Inhibition Processing the visual image begins in the retina. One example is lateral • inhibition. • • • Enhances visual contrast. Horizontal cells provide inhibitory feedback to rods and cones and bipolar cells. Output of horizontal cells is always inhibitory. Prevents lateral spread of light excitation on retina. Contrast is enhanced with excitatory center and inhibitory surround. TMP14, Figure 51-12 TMP14, Figure 51-13 Lateral Inhibition (cont.) APs from ganglion cell 1. Area excited by spot of light. 2. Area adjacent to excited spot. Figure 51-14 Lateral inhibition, the function of horizontal cells Figure 51-15 The Optic Disc (also called the optic nerve head) What is it? • point where ganglion cell axons (~1 million) exit the eye to form the optic nerve (2nd cranial nerve). • entry point for retinal blood vessels • creates a blind spot since there are no rods or cones. • located 3-4 mm to nasal side of fovea. • size: 1.76mm (horizontally) x 1.92mm vertically • Has a central depression called the optic cup edematous optic disc Aka, papilledema Function of Amacrine Cells • About 30 different types. • Their primary targets are ganglion cells • Some are involved in the direct pathway from rods to bipolar cells to amacrine cells to ganglion cells. • A few have action potentials • Some amacrine cells respond strongly to the onset of the visual signal, some to the extinguishment of the signal. • Some respond to movement of a light signal across the retina. • Amacrine cells are a type of interneuron that aid in the beginning of visual signal analysis. Most (but not all) amacrine cells release inhibitory transmitters, GABA or glycine. https://www.ncbi.nlm.nih.gov/pmc/articles/PMC36 52807/ Figure 51-12 Ganglion Cells • More than 20 different types • Different types respond to the following: • Specific directions of motion or orientation • Fine detail • Increases or decreases in light Two classes of ganglion cells: P and M • Particular colors cells P cells (parvocellular cells): • Project to parvocellular layers of lateral geniculate nucleus (LGN) of thalamus • Small receptive fields, slow impulse conduction, sensitive to color and fine details, relatively insensitive to lowM cells (magnocellular cells): contrast signals • Project to magnocellular layers of lateral geniculate nucleus of thalamus • Larger receptive fields • Fast impulse conduction • More sensitive to low contrast B&W stimuli Figure 50-12