Cellular Respiration PDF
Document Details
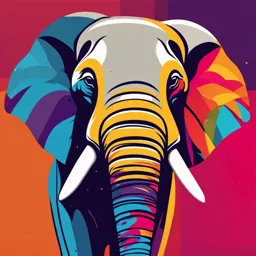
Uploaded by MightyGorgon
Tags
Summary
This document provides a comprehensive overview of cellular respiration, focusing on the stages of aerobic respiration and the role of electron carriers in energy production. It explains the importance of cellular respiration for various biological processes, and discusses the different types of respiration.
Full Transcript
**5 CELLULAR RESPIRATION** **LEARNING OBJECTIVES** At the end of this module the students will be able to: 1\. Explain the four stages of cellular respiration and their respective roles in energy production. 2\. Compare and contrast aerobic respiration with anaerobic respiration, highlighting th...
**5 CELLULAR RESPIRATION** **LEARNING OBJECTIVES** At the end of this module the students will be able to: 1\. Explain the four stages of cellular respiration and their respective roles in energy production. 2\. Compare and contrast aerobic respiration with anaerobic respiration, highlighting the key differences in energy yield, electron acceptors, and products. 3\. Analyze the role of electron carriers (NADH and FADH2) and the electron transport chain in oxidative phosphorylation, explaining how the proton gradient drives ATP synthesis. **LEARNING CONTENT** **AEROBIC RESPIRATION** \- Aerobic respiration is the process by which cells break down glucose in the presence of oxygen to produce energy (ATP), carbon dioxide, and water. - Occurs in: Most eukaryotic organisms, including humans, animals, plants, and some microorganisms \- Importance: Primary method of energy production in complex life forms ***The Equation of Aerobic Respiration*** Glucose + Oxygen → Carbon Dioxide + Water + Energy \- Net ATP yield: Approximately 30-32 ATP molecules per glucose molecule ***Stages of Aerobic Respiration*** 1\. Glycolysis (occurs in cytoplasm) 2\. Pyruvate oxidation (transition step) 3\. Citric Acid Cycle (Krebs Cycle) 4\. Electron Transport Chain and Oxidative Phosphorylation These stages occur in specific cellular locations and involve various enzymes and coenzymes. **Glycolysis** \- Occurs in: Cytoplasm \- Process: Breaks down one glucose molecule into two pyruvate molecules - Energy yield: 2 ATP and 2 NADH \- Key enzymes: Hexokinase, phosphofructokinase, pyruvate kinase - Importance: Universal process in both aerobic and anaerobic respiration ***Pyruvate Oxidation and Citric Acid Cycle*** \- ***Pyruvate Oxidation*** o Converts pyruvate to acetyl-CoA o Produces: 2 NADH, 2 CO2 \- ***Citric Acid Cycle (Krebs Cycle)*** \- Occurs in: Mitochondrial matrix \- Key molecule: Acetyl-CoA enters the cycle \- Products per glucose: 2 ATP, 6 NADH, 2 FADH2, 4 CO2 \- Key enzymes: Citrate synthase, aconitase, isocitrate dehydrogenase ***Electron Transport Chain (ETC)*** \- Location: Inner mitochondrial membrane \- Process: Electrons from NADH and FADH2 pass through a series of protein complexes \- Complexes: I, II, III, and IV \- Function: Generates proton gradient across the membrane \- Final electron acceptor: Oxygen, which combines with H+ to form water ***Oxidative Phosphorylation*** \- Process: Uses proton gradient generated by ETC to synthesize ATP - Key enzyme: ATP synthase (Complex V) \- Chemiosmosis: Protons flow through ATP synthase, driving ATP production - Efficiency: Produces the majority of ATP in aerobic respiration (approximately 26- 28 ATP) ***Importance and Applications*** \- Cellular energy production for vital functions \- Understanding metabolic disorders \- Sports science and exercise physiology \- Biotechnology and fermentation processes \- Environmental science: Role in carbon cycle and climate change ***Regulation and Control*** \- Allosteric regulation of key enzymes \- Feedback inhibition by ATP \- Hormonal control (e.g., insulin, glucagon) \- Oxygen availability \- Substrate concentration (glucose, ADP) \- Cellular energy demand **GLYCOLYSIS** \- Glycolysis is a fundamental metabolic pathway that occurs in nearly all living organisms. \- It is the first step in cellular respiration, where glucose is broken down to produce energy. \- This process takes place in the cytoplasm of cells and does not require oxygen, making it a crucial energy source for both aerobic and anaerobic organisms. ***Overview of Glycolysis*** \- Definition: The breakdown of glucose into pyruvate \- Location: Cytoplasm of cells o Input: One glucose molecule (C6H12O6) o Output: Two pyruvate molecules (C3H3O3) \- Net energy gain: 2 ATP and 2 NADH molecules \- Two main phases: Energy Investment and Energy Harvesting ***Energy Investment Phase: Steps 1-3*** 1\. Glucose Phosphorylation: o Enzyme: Hexokinase o Glucose + ATP → Glucose-6-phosphate + ADP 2\. Isomerization: o Enzyme: Phosphoglucose isomerase o Glucose-6-phosphate → Fructose-6-phosphate 3\. Fructose Phosphorylation: o Enzyme: Phosphofructokinase o Fructose-6-phosphate + ATP → Fructose-1,6-bisphosphate + ADP ***Energy Investment Phase: Steps 4-5*** 4\. Splitting of Fructose-1,6-bisphosphate: o Enzyme: Aldolase o Fructose-1,6-bisphosphate → Dihydroxyacetone phosphate + Glyceraldehyde-3-phosphate 5\. Isomerization of Dihydroxyacetone phosphate: o Enzyme: Triose phosphate isomerase o Dihydroxyacetone phosphate ⇌ Glyceraldehyde-3-phosphate Note: At this point, we have two 3-carbon molecules entering the Energy Harvesting Phase. ***Energy Harvesting Phase: Steps 6-7*** 6\. Oxidation and Phosphorylation: o Enzyme: Glyceraldehyde-3-phosphate dehydrogenase o Glyceraldehyde-3-phosphate + NAD⁺ + Pi → 1,3-Bisphosphoglycerate + NADH + H⁺ 7\. First ATP Generation: o Enzyme: Phosphoglycerate kinase o 1,3-Bisphosphoglycerate + ADP → 3-Phosphoglycerate + ATP ***Energy Harvesting Phase: Steps 8-10*** 8\. Isomerization: o Enzyme: Phosphoglycerate mutase o 3-Phosphoglycerate → 2-Phosphoglycerate 9\. Dehydration: o Enzyme: Enolase o 2-Phosphoglycerate → Phosphoenolpyruvate + H₂O 10.Second ATP Generation: o Enzyme: Pyruvate kinase o Phosphoenolpyruvate + ADP → Pyruvate + ATP ***Net Results of Glycolysis*** \- For each glucose molecule: o 2 Pyruvate molecules produced o 2 ATP molecules consumed (steps 1 and 3) o 4 ATP molecules produced (steps 7 and 10) o Net gain: 2 ATP molecules o 2 NADH molecules produced o 2 water molecules produced **Significance of Glycolysis** \- Universal energy pathway in living organisms \- Provides energy for both aerobic and anaerobic processes \- Supplies pyruvate for the citric acid cycle in aerobic respiration \- Crucial for fermentation in anaerobic conditions \- Important in various metabolic pathways (e.g., gluconeogenesis, lipid synthesis) ***Regulation of Glycolysis*** Key regulatory enzymes: 1\. Hexokinase (step 1) 2\. Phosphofructokinase (step 3) 3\. Pyruvate kinase (step 10) Factors affecting regulation: ∙ Energy status of the cell (ATP/AMP ratio) ∙ Hormonal control (e.g., insulin, glucagon) ∙ Substrate availability ∙ Product inhibition **PYRUVATE OXIDATION** \- Pyruvate oxidation is a crucial metabolic process \- Occurs in the mitochondrial matrix \- Links glycolysis to the citric acid cycle (Krebs cycle) \- Converts pyruvate into acetyl-CoA \- Involves a series of enzyme-catalyzed reactions \- Key player: Pyruvate Dehydrogenase Complex (PDC) ***The Pyruvate Dehydrogenase Complex (PDC)*** \- Large, multi-enzyme complex \- Composed of three main enzymes: 1\. Pyruvate dehydrogenase (E1) 2\. Dihydrolipoyl transacetylase (E2) 3\. Dihydrolipoyl dehydrogenase (E3) \- Requires five coenzymes: o Thiamine pyrophosphate (TPP) o Lipoic acid o Coenzyme A (CoA) o Flavin adenine dinucleotide (FAD) o Nicotinamide adenine dinucleotide (NAD+) ***Step 1: Decarboxylation*** \- Catalyzed by pyruvate dehydrogenase (E1) \- Pyruvate (C3) loses a carbon dioxide (CO2) \- Forms a hydroxyethyl-TPP intermediate \- Reaction: Pyruvate + TPP \\rightarrow Hydroxyethyl-TPP + CO2 - TPP stabilizes the carbanion intermediate ***Step 2: Oxidation and Transfer to Lipoamide*** \- Hydroxyethyl group oxidized to an acetyl group \- Transferred to lipoic acid (bound to E2) \- Forms acetyl-lipoamide \- Reaction: Hydroxyethyl-TPP + Lipoamide \\rightarrow Acetyl-lipoamide + TPP - E1 is regenerated for the next cycle ***Step 3: Transfer to Coenzyme A*** \- Catalyzed by dihydrolipoyl transacetylase (E2) \- Acetyl group transferred from lipoamide to CoA \- Forms acetyl-CoA (the end product) \- Reaction: Acetyl-lipoamide + CoA \\rightarrow Acetyl-CoA + Dihydrolipoamide - Dihydrolipoamide remains bound to E2 ***Step 4: Regeneration of Lipoamide*** \- Catalyzed by dihydrolipoyl dehydrogenase (E3) \- Oxidizes dihydrolipoamide back to lipoamide \- Uses FAD as an electron acceptor \- FADH2 then transfers electrons to NAD+ \- Reaction:  ***Overall Reaction and Energy Yield*** ∙ Net reaction: ∙ Energy yield: o 1 NADH (equivalent to 2.5 ATP in electron transport chain) o 1 CO2 released o High-energy acetyl-CoA produced (feeds into citric acid cycle) ***Regulation of Pyruvate Dehydrogenase Complex*** ∙ PDC activity is tightly controlled ∙ Inhibited by: o High ratios of ATP/ADP, NADH/NAD+, and acetyl-CoA/CoA o Phosphorylation by pyruvate dehydrogenase kinase ∙ Activated by: o Dephosphorylation by pyruvate dehydrogenase phosphatase o High pyruvate concentrations o Insulin (in some tissues) ***Clinical Significance*** ∙ PDC deficiency can lead to lactic acidosis ∙ Important in metabolic disorders and diabetes ∙ Therapeutic target for various diseases **KREBS CYCLE** \- Also known as the Citric Acid Cycle or Tricarboxylic Acid (TCA) Cycle - Central metabolic pathway in cellular respiration \- Occurs in the mitochondrial matrix \- Discovered by Hans Krebs in 1937 \- Key role in energy production and biosynthesis ***Overview of the Krebs Cycle*** ∙ Cyclic process with 8 major steps ∙ Oxidizes acetyl-CoA derived from carbohydrates, fats, and proteins ∙ Produces energy carriers (NADH and FADH₂) and CO\_2 ∙ Generates 2 ATP molecules per glucose molecule ∙ Provides precursors for amino acid and nucleotide synthesis ***Step 1: Citrate Formation*** \- Acetyl-CoA (2-carbon) combines with oxaloacetate (4-carbon) - Catalyzed by citrate synthase \- Forms citrate (6-carbon molecule) \- Reaction: ***Steps 2-3: Isomerization and Oxidative Decarboxylation Step 2: Isomerization*** \- Citrate converted to isocitrate \- Catalyzed by aconitase \- Involves formation of cis-aconitate intermediate ***Step 3: Oxidative Decarboxylation*** \- Isocitrate oxidized to α-ketoglutarate \- Catalyzed by isocitrate dehydrogenase \- Produces NADH and releases CO2 **Steps 4-5: α-Ketoglutarate to Succinyl-CoA to Succinate *Step 4: Oxidative Decarboxylation*** \- α-Ketoglutarate converted to succinyl-CoA \- Catalyzed by α-ketoglutarate dehydrogenase complex \- Produces NADH and releases CO2 ***Step 5: Substrate-level Phosphorylation*** \- Succinyl-CoA converted to succinate \- Catalyzed by succinyl-CoA synthetase \- Produces GTP (equivalent to ATP) ***Steps 6-7: Succinate to Fumarate to Malate*** ***Step 6: Oxidation of Succinate*** \- Succinate oxidized to fumarate \- Catalyzed by succinate dehydrogenase \- Produces FADH2 ***Step 7: Hydration of Fumarate*** \- Fumarate hydrated to malate \- Catalyzed by fumarase \- Adds water molecule to fumarate ***Step 8: Regeneration of Oxaloacetate*** \- Malate oxidized to oxaloacetate \- Catalyzed by malate dehydrogenase \- Produces NADH \- Regenerates oxaloacetate to continue the cycle ***Energy Yield and Importance*** \- Per acetyl-CoA molecule: o 3 NADH and 1 FADH2 produced o 1 GTP (or ATP) generated \- Per glucose molecule (2 acetyl-CoA): o 6 NADH, 2 FADH2, and 2 ATP produced \- NADH and FADH2 feed into electron transport chain - Crucial for ATP production in cellular respiration **Clinical Significance and Disorders** \- Krebs cycle defects can lead to various metabolic disorders - Examples: o Fumarase deficiency o Pyruvate dehydrogenase deficiency o Isocitrate dehydrogenase mutations in cancer \- Understanding the cycle is crucial for: o Diagnosing metabolic disorders o Developing targeted therapies o Exploring cancer metabolism **ELECTRON TRANSPORT CHAIN** \- The Electron Transport Chain (ETC) is a crucial process in cellular respiration - Occurs in the inner mitochondrial membrane of eukaryotic cells - Final stage of cellular respiration, following glycolysis and the citric acid cycle - Main purpose: Generate ATP through oxidative phosphorylation - Involves a series of redox reactions and protein complexes ***Components of the Electron Transport Chain*** 1\. Electron carriers: o NADH and FADH₂ (from earlier stages of respiration) o Coenzyme Q (ubiquinone) o Cytochrome c 2\. Protein complexes: o Complex I (NADH dehydrogenase) o Complex II (Succinate dehydrogenase) o Complex III (Cytochrome bc₁ complex) o Complex IV (Cytochrome c oxidase) 3\. ATP synthase (Complex V) ***Steps of the Electron Transport Chain*** 1\. Electron donation: o NADH donates electrons to Complex I o FADH₂ donates electrons to Complex II 2\. Electron transfer: o Electrons move through the complexes and mobile carriers o Energy released pumps protons (H⁺) into the intermembrane space 3. Oxygen reduction: o Electrons reach Complex IV and reduce oxygen to water ***Proton Gradient Formation*** \- As electrons move through the ETC, energy is released \- This energy is used to pump protons (H⁺) from the matrix to the intermembrane space \- Proton pumping occurs at: o Complex I o Complex III o Complex IV \- Results in a higher concentration of H⁺ in the intermembrane space - Creates an electrochemical gradient across the inner mitochondrial membrane **CHEMIOSMOSIS** \- The movement of ions across a selectively permeable membrane down their electrochemical gradient \- In ETC, chemiosmosis refers to the flow of protons (H⁺) back into the mitochondrial matrix \- Protons move through ATP synthase (Complex V) \- The energy from this proton flow drives ATP synthesis \- Couples the electron transport chain to oxidative phosphorylation **OXIDATIVE PHOSPHORYLATION** \- The process of ATP production using the energy from the electron transport chain \- Occurs in ATP synthase (Complex V) \- Steps: 1\. Protons flow through ATP synthase, causing it to rotate 2\. Rotation changes the shape of the enzyme\'s active site 3\. ADP and inorganic phosphate (Pi) are brought together 4\. ATP is formed:  \- Produces the majority of ATP in aerobic respiration (up to 34 ATP molecules) ***Energy Yield and Efficiency*** \- NADH yields more ATP than FADH₂: o NADH: \~2.5 ATP per molecule o FADH₂: \~1.5 ATP per molecule \- Theoretical maximum ATP production: o 10 NADH × 2.5 = 25 ATP o 2 FADH₂ × 1.5 = 3 ATP o 2 ATP from substrate-level phosphorylation o Total: \~30 ATP per glucose molecule \- Actual yield is lower due to inefficiencies (typically 26-28 ATP) \- ETC is more efficient than anaerobic respiration (2 ATP from glycolysis alone) ***Regulation and Inhibition*** ∙ Regulation: o ATP/ADP ratio o NADH/NAD⁺ ratio o Oxygen availability ∙ Inhibitors: o Rotenone (Complex I) o Antimycin A (Complex III) o Cyanide (Complex IV) o Oligomycin (ATP synthase) ∙ Uncouplers (e.g., 2,4-dinitrophenol) disrupt the proton gradient without inhibiting electron flow ***Clinical Relevance and Research*** ∙ Mitochondrial diseases: o Genetic mutations affecting ETC components o Can lead to various disorders (e.g., MELAS syndrome, Leigh syndrome) ∙ Aging: o Mitochondrial theory of aging involves ETC dysfunction ∙ Drug targets: o ETC components as potential targets for new therapeutics ∙ Research areas: o Improving mitochondrial function o Developing treatments for mitochondrial disorders **ANAEROBIC RESPIRATION** \- Anaerobic respiration is a metabolic process that produces energy without the use of oxygen \- Occurs in some microorganisms and in muscle cells during intense exercise - Less efficient than aerobic respiration but allows organisms to survive in oxygen-poor environments \- Key difference from aerobic respiration: final electron acceptor is not oxygen ***Steps of Anaerobic Respiration*** 1\. Glycolysis: o Glucose is broken down into two pyruvate molecules o Produces 2 ATP and 2 NADH 2\. Fermentation: o Pyruvate is converted to either lactic acid or ethanol o Regenerates NAD+ for continued glycolysis 3\. Electron Transport Chain (partial): o Electrons are passed through a shortened chain o Final acceptor varies (e.g., sulfate, nitrate) ***Types of Anaerobic Respiration*** ***1. Lactic Acid Fermentation:*** o Occurs in muscle cells during intense exercise o End product: lactic acid o Example: ***2. Alcoholic Fermentation:*** o Occurs in yeast and some bacteria o End products: ethanol and CO₂ o Example:  ***Importance and Applications*** ∙ Survival mechanism for organisms in oxygen-poor environments ∙ Industrial applications: o Bread making and beer brewing (yeast fermentation) o Production of biofuels ∙ Medical relevance: o Understanding muscle fatigue and recovery o Studying certain bacterial infections **SYNTHESIS** Aerobic respiration, the primary energy-producing process in most living organisms, breaks down glucose in the presence of oxygen to generate ATP, the cell\'s energy currency. This complex process occurs in four stages: glycolysis, pyruvate oxidation, the citric acid cycle, and oxidative phosphorylation. Glycolysis, occurring in the cytoplasm, breaks down glucose into pyruvate, producing a small amount of ATP and NADH. Pyruvate oxidation and the citric acid cycle, taking place in the mitochondrial matrix, further oxidize pyruvate, generating more NADH and FADH2, along with CO2. The final stage, oxidative phosphorylation, utilizes the electron carriers NADH and FADH2 to drive ATP synthesis via the electron transport chain and chemiosmosis, producing the majority of ATP. Aerobic respiration is a highly efficient process, generating significantly more ATP per glucose molecule than anaerobic respiration. This process is essential for the survival of most living organisms, providing the energy needed for vital functions such as muscle contraction, nerve impulse transmission, and biosynthesis. Understanding aerobic respiration is crucial for comprehending the intricate workings of cellular metabolism, the development of metabolic disorders, and the impact of exercise and nutrition on human health. Furthermore, this knowledge is vital for exploring the role of aerobic respiration in various fields, including biotechnology, environmental science, and sports science. **WORKSHEET** **DIRECTION:** On a separate paper answer the following questions: **Fill in the Blank**: Fill in the blank with the correct words. 1\. The first stage of cellular respiration, which occurs in the cytoplasm and does not require oxygen, is called\_\_\_\_\_\_\_\_\_\_\_\_\_\_\_\_\_\_\_\_\_. 2\. The \_\_\_\_\_\_\_\_\_\_\_\_\_\_\_\_\_\_\_\_\_ is the final stage of cellular respiration, where the majority of ATP is produced through oxidative phosphorylation. 3\. The process that converts pyruvate into acetyl-CoA, linking glycolysis to the citric acid cycle, is called \_\_\_\_\_\_\_\_\_\_\_\_\_\_\_\_\_\_\_\_\_. 4\. In the absence of oxygen, some cells perform \_\_\_\_\_\_\_\_\_\_\_\_\_\_\_\_\_\_\_\_\_respiration, which produces less ATP but allows for continued energy production. 5\. The enzyme \_\_\_\_\_\_\_\_\_\_\_\_\_\_\_\_\_\_\_\_\_ is responsible for catalyzing the formation of ATP from ADP and inorganic phosphate during oxidative phosphorylation. **Multiple Choice Questions**: Choose the correct answer from the choices for each question. 1\. Which of the following is NOT a stage of aerobic cellular respiration? a) Glycolysis b\) Citric acid cycle c\) Fermentation d\) Electron transport chain 2\. How many ATP molecules are typically produced from one glucose molecule during aerobic respiration? a\) 2 b\) 4 c\) 18 d\) 30-32 3\. Where does the citric acid cycle take place in eukaryotic cells? a\) Cytoplasm b\) Mitochondrial matrix c\) Inner mitochondrial membrane d\) Endoplasmic reticulum 4\. Which molecule serves as the final electron acceptor in the electron transport chain? a\) NAD+ b\) FAD c\) Oxygen d\) Pyruvate 5\. What is the primary purpose of the electron transport chain? a\) To break down glucose b\) To generate a proton gradient c\) To produce acetyl-CoA d\) To directly synthesize ATP **Open Ended Questions**: Answer the following questions in complete sentences: 1\. Explain the importance of oxygen in aerobic cellular respiration. How does its presence affect ATP production? 2\. Compare and contrast aerobic and anaerobic respiration in terms of their efficiency and end products. 3\. Describe the role of electron carriers (such as NADH and FADH2) in the electron transport chain and how they contribute to ATP production. **REFERENCES** 1\. Berg, J. M., Tymoczko, J. L., & Stryer, L. (2012). Biochemistry (7th ed.). W.H. Freeman and Company. 2\. Nelson, D. L., & Cox, M. M. (2017). Lehninger Principles of Biochemistry (7th ed.). W.H. Freeman and Company. 3\. Alberts, B., Johnson, A., Lewis, J., Morgan, D., Raff, M., Roberts, K., & Walter, P. (2014). Molecular Biology of the Cell (6th ed.). Garland Science. 4\. Voet, D., & Voet, J. G. (2011). Biochemistry (4th ed.). John Wiley & Sons. 5\. Lodish, H., Berk, A., Kaiser, C. A., Krieger, M., Bretscher, A., Ploegh, H., Amon, A., & Martin, K. C. (2016). Molecular Cell Biology (8th ed.). W.H. Freeman and Company. 6\. Voet, D., Voet, J. G., & Pratt, C. W. (2016). Fundamentals of Biochemistry: Life at the Molecular Level (5th ed.). Wiley. 7\. Ferrier, D. R. (2017). Lippincott Illustrated Reviews: Biochemistry (7th ed.). Wolters Kluwer. 8\. Patel, M. S., Nemeria, N. S., Furey, W., & Jordan, F. (2014). The pyruvate dehydrogenase complexes: Structure-based function and regulation. Journal of Biological Chemistry, 289(24), 16615-16623. 9\. Patel, K. P., O\'Brien, T. W., Subramony, S. H., Shuster, J., & Stacpoole, P. W. (2012). The spectrum of pyruvate dehydrogenase complex deficiency: Clinical, biochemical and genetic features in 371 patients. Molecular Genetics and Metabolism, 106(3), 385-394. 10.Holness, M. J., & Sugden, M. C. (2003). Regulation of pyruvate dehydrogenase complex activity by reversible phosphorylation. Biochemical Society Transactions, 31(6), 1143-1151. 11.Krebs, H. A., & Johnson, W. A. (1937). The role of citric acid in intermediate metabolism in animal tissues. Enzymologia, 4, 148-156. 12.Akram, M. (2014). Citric acid cycle and role of its intermediates in metabolism. Cell Biochemistry and Biophysics, 68(3), 475-478. 13.Fernie, A. R., Carrari, F., & Sweetlove, L. J. (2004). Respiratory metabolism: glycolysis, the TCA cycle and mitochondrial electron transport. Current Opinion in Plant Biology, 7(3), 254-261. 14.Nicholls, D. G., & Ferguson, S. J. (2013). Bioenergetics (4th ed.). Academic Press.