Diffusion of Gases and PFTS Lecture Outline PDF
Document Details
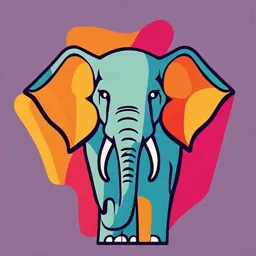
Uploaded by SuperiorPsaltery
Tags
Summary
This document provides an outline for a lecture on diffusion of gases and pulmonary function tests. It covers topics including gas movement, limitations of gas transfer, and diffusion of oxygen and carbon dioxide.
Full Transcript
Diffusion of Gases and PFTS Lecture Outline I. Gas Movement II. Limitations of Gas Transfer III. Diffusion of O2 and CO2 IV. Pulmonary Function Tests 1 Diffusion of Gases and PFTS Objectives 1. 2. 3. 4. 5. 6. 7. 8. Identify how gas moves through the respiratory system from ambient air to mitochondri...
Diffusion of Gases and PFTS Lecture Outline I. Gas Movement II. Limitations of Gas Transfer III. Diffusion of O2 and CO2 IV. Pulmonary Function Tests 1 Diffusion of Gases and PFTS Objectives 1. 2. 3. 4. 5. 6. 7. 8. Identify how gas moves through the respiratory system from ambient air to mitochondria using bulk flow and diffusion Understand Henry’s Law as it relates to respiratory physiology Understand the components of Fick’s Law for diffusion Identify how barometric pressure affects partial pressure of O2 Understand and compare the limitations to gas transfer Describe oxygen consumption and diffusing capacity during exercise Describe the etiology and effects of hyper- and hypoventilation Understand the use of PFTs including testing, interpretation, and common findings 2 References Assigned reading from your text: Levitzky Chapter 6 3 I. Gas Movement 4 Overview of Respiration Metabolic rate of cells sets the demand for gas exchange. The Respiratory system combines 3 Main Processes to Provide oxygen to cells: 1- Respiration During bulk flow- gas moves according to total pressure During diffusion- each gas moves according to its own individual partial pressure 2- Circulation During bulk flow- blood carries O2/CO2 to/from tissues 3- Metabolism Diffusion of O2 from peripheral capillaries to mitochondria depends on capillary PO2 (PO2 of mixed venous blood reflects average tissue PO2) Gas Movement- Bulk Flow q Gas movement through the conducting airways results from differences in total pressure External convection – Bulk flow- aka mass movement or convection – Molecules of different gases move together along the total pressure gradient Internal Convection – Bulk flow also occurs as blood carries oxygen out of lung by to tissues 6 Gas Movement- Diffusion q In the respiratory airways, gases move by diffusion – Gases move according to their own partial pressures q Oxygen must dissolve in and diffuse through the: § Alveolar-capillary barrier (respiratory or pulmonary membrane): – Pulmonary surfactant lining Alveolar epithelium – Interstitial space – Capillary endothelium § Plasma – Some O2 remains dissolved in plasma – Most enters the RBC to combine with hemoglobin in blood (bulk flow) § At the tissues, O2 diffuses from the RBC through: § Plasma § Capillary endothelium § Interstitium § tissue cell membrane § Cell interior into the mitochondrial membrane 7 Henry’s Law q States that at a constant temperature, the amount of gas that dissolves in solution is directly proportional to the partial pressure of that gas over solution The higher the partial pressure of the gas, the more it will dissolve into a liquid (solubility) Temperature affects solubility: - Lower temperature = increased solubility (Anesthetic emergence is prolonged during hypothermia) - Higher temperature= decreased solubility Each gas has its own solubility coefficient - O2 = 0.003 mL/dL/mmHg PaO2 x 0.003 = the amount of O2 dissolved in plasma - CO2= 0.06 mL/dL/mmHg PaCO2 x 0.06 = the amount of CO2 dissolved in plasma - CO2 is 20 x more soluble in plasma than O2 Anesthesia applications: - Increasing the partial pressure of the gas- FiO2 or pressure change (hyperbaric) - Increasing the concentration of VA to hasten transfer and “overpressure” the vaporizer 8 Dissolved Oxygen qOnly the part of gas that is dissolved contributes to the partial pressure of oxygen in blood Gases diffuse according to their individual partial pressure gradients An area of higher partial pressure has a greater number of molecules and causes the net movement of molecules to an area of lower partial pressure PO2 Mixed venous blood Alveolar gas Arterial blood Tissue gases Mitochondria PCO2 40 mmHg 45 mmHg 104 mmHg 40 mmHg 100 mmHg 40 mmHg 45 mmHg 1-5 mmHg 48 mmHg qMajor Considerations in this presentation: At the alveolar-capillary unit, O2 and CO2 continuously diffuse In lungs CO2 pressure gradient (5 mm Hg) is less than O2 pressure gradient (64 mmHg) CO2 diffuses 20x faster than O2 and does not require a large pressure gradient O2 is more likely to present a problem with diffusion than CO2 Movement of gases across the alveolar-capillary unit is rapid; Tensions equilibrate in 0.25 s Blood gas tension refers to the partial pressure of gases in blood 9 Fick’s Law of Diffusion q Fick’s Law describes the rate of diffusion of gas through a tissue sheet ‘ q Main factors of the Fick equation Rate of diffusion of a gas in a liquid is directly proportional to the: 1. (A) Surface area of the membrane – more membrane increases the rate of diffusion 2. (D) Diffusion coefficient or solubility – more soluble gas diffuses more quickly 3. (P1-P2) Partial pressure difference driving diffusion – higher gradient speeds diffusion Rate of diffusion of a gas is inversely proportional to the: 4. (T) Thickness of the membrane 5. Molecular weight of the gas Anesthesia applications: - Fick is used to calculate cardiac output - Diffusion hypoxia that occurs after N2O has been discontinued - COPD patient has reduced alveolar surface for diffusion 10 Fick’s Law of Diffusion – Surface Area qThe area available for diffusion = effect gas exchange surface Includes only surface area of alveoli that are both ventilated and perfused Decreased surface area available for diffusionà decreases the rate of diffusion: eg Emphysema patients have a loss of alveolar septae (walls) Increased surface area available for diffusion increases the rate of diffusion: Increased cardiac output such as exercise increases the surface area Increased cardiac outputà increased pulmonary blood flowà recruitment Greater expansion of lungsà increased number alveoli expanding As many as half of pulmonary capillaries may not be perfused at rest 11 Fick’s Law of Diffusion – Partial Pressure Gradient q Pressure gradient = the difference in partial pressures of the gas across the barrier The initial pressure gradient is important – – – – Mixed venous blood entering a capillary has a PO2 of 40 mmHg Arterial blood exiting that capillary has a PO2 of 104 mmHg Smaller gradient produces a slower rate of diffusion; PAO2 of 50 mmHg diffuses more slowly Giving 100% O2 (FiO2 1.0) increases the pressure gradient and speeds rate of transfer Blood stays in pulmonary capillary ~ 0.75 seconds – – At rest, gases equilibrate in 0.25 sec During the last ~0.5 sec in the capillary, the PO2 is 104 mmHgà NO O2 exchange 12 Fick’s Law of Diffusion – Barometric Pressure Gradient q Individual partial pressures of O2 contribute to the total barometric pressure – – Gas pressure determines the availability of gas molecules Gas pressure gradient is responsible for movement of gas molecules Comparison of O2 in ambient air at one atmosphere (760 mmHg) – 760 mmHg x 0.21 = 160 mmHg At high altitudes (hypobaric) - atmospheric pressure is less than at sea level O2 in air remains 21% – 20,000’ Atmospheric Pressure = 349 mm Hg -47 mmHg= 302 mmHg x.21= 63 mmHg – – – – A low PAO2 causes a decreased PAO2-PaO2 gradient Equilibration takes even longer Altitude can limit the transfer (diffusion) of oxygen Low barometric pressure before storms decreases PAO2 Hyperbaric involves gas at a pressure greater than normal: O2 in compressed air at two atmospheres (1520 mmHg) – 1520 mmHg x 0.21 = 320 mmHg – – At great depths, gas density becomes a problem Increases airways resistance and work of breathing Work of breathing through nose increased – Helium may be used in deep dives in place of Nitrogen Helium has a low molecular weight and lipid solubility Reduces narcotizing effect of N and O2 toxicity 13 Fick’s Law of Diffusion – Membrane Thickness qMembrane thickness = the distance a gas must diffuse Rate of diffusion is inversely proportional to the length of the alveolar-capillary unit This distance includes the fluid and the respiratory membrane A normal alveolar-capillary diffusion barrier is ~ 0.2 to 0.5 um thick. Examples of increases in barrier thickness slow diffusion: Interstitial fibrosis Interstitial pulmonary edema Membrane thickness likely decreases at high lung volumes when alveoli are stretched The diffusion distance decreases slightly 14 Fick’s Law of Diffusion – Diffusion Coefficient q Diffusion coefficient: the rate of diffusion of a gas is directly proportional is a measure of ease by which a gas is able to move from one area to another – D (diffusion coefficient ) µ Solubility √ molecular weight q Two factors affecting diffusion coefficient: Solubility coefficient of a gas – – – Rate at which a gas diffuses is directly proportional to the solubility of the gas ie A more soluble gas will diffuse faster in a liquid than a lesser soluble gas Solubility of CO2 is 21x greater than that of O2 Molecular weight of a gas – Graham’s law: the relative rates of diffusion of two gases are inversely proportional to the square roots of their molecular weights if all else is equal – – – The heavier the molecule the slower its rate of diffusion; CO2 has a heavier molecular weight than O2à per Grahamà O2 should be faster But the solubility of the gas must also be considered Diffusion coefficient (molecular weight + solubility coefficient) for CO2 is 20 x greater than O2 15 II. Limitations of Gas Transfer 16 Limitations Of Gas Transfer q Important partial pressures of a gas when determining the rate of diffusion include: Alveolar Mixed venous blood Pulmonary capillary blood q Perfusion-limited transfer equilibrates The amount of gas transferred across the alveolar-capillary unit is limited by the rate of pulmonary blood flow If a gas equilibrates between alveoli and pulmonary capillary blood plasmaà it is perfusion limited because it is limited by the flow rate of blood q Diffusion-limited transfer does not equilibrate The amount of gas transferred across the alveolar-capillary unit is limited by the ability of that gas to diffuse If a gas does not equilibrate between alveoli and pulmonary capillary blood plasmaà it is diffusion limited because its pressure gradient across the alveolar-capillary unit is maintained the entire time blood is flowing through the pulmonary capillary. Its diffusion is solely dependent on the condition of the respiratory membrane 17 Examples of Gases and Their Limitations q N2O is an example of a perfusion-limited gas It equilibrates very quickly between pulmonary capillary blood plasma and alveolar air Equilibration time is ~0.15 s The transfer of N2O can only be increased by increasing cardiac output q O2 is normally perfusion-limited Equilibrates slower than N2O It combines with Hb prior to reaching PO2 q CO is diffusion-limited It binds readily to Hb & is removed from solution Doesn’t create tension since there is minimal dissolved C CO’s affinity for hemoglobin is 210 x greater than O2 It is only limited by properties of the respiratory membrane Used in small amounts to determine diffusing capacity 18 III. Diffusion of O2 and CO2 19 Oxygen Is Normally A Perfusion Limited Gas qO2 does not have difficulty with diffusion Under normal resting conditions diffusion of oxygen is solely related to perfusion Blood remains in pulmonary capillaries for ~ 0.75 seconds O2 and CO2 tensions equilibrate within 0.25 seconds During increased cardiac output such as exercise, PaO2 equilibrates with PAO2 0.25 seconds is still sufficient for transfer of gas to occur Total oxygen transfer is normally increased in exercise Recruitment of previously unperfused capillaries (increases the surface area) for diffusion And better matches ventilation and perfusion 20 Changes when Diffusion Path is Increased q Oxygen can become diffusion limited when diffusion path from alveolar gas to RBC is increased This graph shows the effect of a low PAO2 on O2 transfer from the alveolus to the capillary The low PAO2 sets the upper limit for the Pend-capillaryO2; PaO2 and Mixed venous PO2 are decreased q Factors that limit O2 diffusion: Loss of surface area available for diffusion of oxygen Reduced alveolar partial pressure of oxygen (eg at altitude) limits O2 transfer Decreased time available for exchange due to increased velocity of flow Any hypoxemia that occurred at rest will be exaggerated with reduced contact time Increased thickness of the alveolar-capillary membrane from multiple pathologies: Fibrosis Sarcoidosis Interstitial pneumonia Connective tissue diseases (scleroderma, lupus) The 6 Minute Walk Test assesses lung function by having the patient walk 100 feet and assessing SpO2 21 CO2 Is Normally Perfusion Limited qSimilar to O2 the time course of CO2 transfer from the pulmonary capillary blood to the alveolus CO2 equilibrates in slightly more than 0.25 of a second 20 x more soluble than O2 But- the partial pressure difference is only 5 mmHg qConditions of respiratory membrane impairment are going to be reflected as hypoxia instead of hypercapnia due to the diffusion coefficient of CO2 There is normally not diffusion limitation of the exchange of CO2 because this gas is more soluble than oxygen in the alveolar–capillary membrane, which facilitates CO2 exchange. The complex reactions involved in carbon dioxide transport proceed with sufficient rapidity to avoid being a significant limiting factor in exchange. 22 Diffusing Capacity Or Transfer Factor q Diffusing capacity measures the lungs ability to exchange gas This is the rate at which a volume of O2 or CO2 diffuses through the respiratory membrane per minute and per mmHg The diffusing capacity of the lung is determined both by 1. 2. The diffusing capacity of the membrane (alveolar–capillary and RBC) Reaction with hemoglobin Diffusion through the alveolus is normally very rapid; but can change with edema or pneumonia q Measurement of DLCO; CO is used in nonlethal amounts The rate at which oxygen or carbon monoxide (CO) is absorbed from alveolar gas into the pulmonary capillaries (ml/min) per unit of partial pressure difference (mmHg) DLO2 = Diffusing capacity of the lung for oxygen – Must be calculated since too complex to measure directly DLCO = CO often measured since pulmonary capillary CO = 0 mmHg – – – A small amount of CO is inhaled for a brief time and volume absorbed/unit time measured The diffusing capacity for O2 is 1.23 x that of COCO measurement multiplied by 1.23 23 Oxygen Consumption q At rest, humans consume about 250 ml O2/minute This consumption can increase 10 x in a healthy subject; 20 x in trained athlete (to 5000 ml O2/min) Cardiac output can increase 4-5 x above resting VE can increase 20 x above resting Oxygen consumption is limited by: Maximal cardiac output Mitochondrial density Respiratory limitations q Increased diffusing capacity of O2 during exercise is accomplished by: Mainly by an increase in cardiac output by an increase in pulmonary blood flow Increased effective gas exchange areaà recruitment of dormant pulmonary capillaries Increased blood volume due to distention of pulmonary capillaries And by Increased ventilation à increased pressure gradient Increased O2 consumption of exercising skeletal muscleà Mixed venous PO2 is lower (40 mmHg at rest)- 35 mmHg exercising Mixed venous PCO2 is higher than at rest à. Helps to maintain diffusion 24 Altered Rates Of Ventilation- Hyperventilation qWith hyperventilation- the composition of alveolar air begins to approach that of tracheal or ambient air (PO2 ambient air = 160 mm hg; PAO2 = 130 mm2Hg) Composition of arterial blood partial pressures change: PO2 increases PCO2 decreases - hypocapnia Causes of hyperventilation: Hypoxia Emotional stress Early respiratory failure or early CHF Effects of hyperventilation and hypocapnia: Cerebral vasoconstriction Respiratory alkalosis - due to loss of CO2 Tetany- In alkalosis Ca+2 binds to plasma proteinsà decreased plasma Ca+2 affects Na+ voltage-gated channelsà Na+ leaks more easily into muscle cells Causes hypopolarization à raises resting potential closer to threshold Allows muscles to contract in spasmsà tetany or carpopedal spasms 25 Altered Rates Of Ventilation- Hypoventilation qComposition of arterial blood partial pressures change: PO2 decreases PCO2 increases - hypercapnia Causes: Hypoventilation syndromes, pharmacologic agents Effects of hypoventilation: Leads to hypoxia and hypercapnia Increased PaCO2 Respiratory acidosis develops due to increased PaCO2 Hypercapnia causes pulmonary vasoconstriction / pulmonary hypertension Decreased PaO2 Hypoxia stimulates erythropoietin à results in polycythemia - increased RBC production Causes increased viscosity of blood Increases peripheral resistance Increases MAP Alveolar hypoxia à active vasoconstriction of pulmonary circulation causes HPV 26 IV. Pulmonary Function Tests 27 Pulmonary Function Tests (PFTs) q PFTs Measure: Static lung volumes Dynamic lung volumes of how quickly air can be moved in/out of lungs Diffusing capacity- how well lungs can transfer gas across the respiratory membrane q Pulmonary function tests are used to: Diagnose disorders Evaluate severity of a pulmonary disorder Evaluation of the course of therapy Preoperative screening to predict likelihood of postoperative pulmonary complications q Testing: Normal standard values from nomograms for age, gender and height Compare predicted to recorded values Due to lower sensitivity, a value of + 20% of predicted value is taken as normal Utilizes a spirometer or plethysmograph to acquire data Done in a seated or upright position and require cooperation Tests are repeated and highest value taken Observed value x 100 = value + 20% predicted value 28 Interpreting PFTs q Method of Evaluation: – Review lung volumes - FRC, TLC, RV If >120% predicted, consider hyperinflation secondary to obstruction If < 80% predicted, consider restriction secondary to lung/chest stiffness – Look at spirometry - FEV1/FVC, FEF25-75% Reduced values confirm obstruction Normal values confirm restriction q Common PFTs Maximum voluntary ventilation (MVV) : Patient breaths as rapidly and deeply as possible Gives information regarding: Respiratory muscle strength and endurance Compliance Resistance related to breathing Closing volume: Most sensitive test in the detection of early airway closure Detects closure of small airways Increases with age and obstructive disease FRC: Changes will be primarily due to an increased or decreased RV 30 Restrictive Diseases q Restrictive diseases result in difficulty inspiring Decreased compliance Limited lung expansion Volumes/capacities lower Compliance curves shifted to the right FEV1/FVC > normal 31 Obstructive Diseases q Obstructive disease are divided into: Fixed obstructions Both inspiration/expiration limbs flat eg Tracheal stenosis or mass in large airways Increased airways resistance both I&E Variable inspiratory obstructions Extrathoracic upper airways problems (eg OSA) Affect inspiration more than expiration FEV1/FVC is usually normal Variable expiratory obstructions Intrathoracic airway problems FEV1/FVC low with a low peak expiratory flow Asthma- FEV1/FVC improves with bronchodilation or decreases with bronchoprovocation Chronic bronchitis- copious sputum, normal DLCO, Low PaO2, high PaCO2 Emphysema- alveolar destruction, low DLCO, slightly decreased PaO2, slightly increased PaCO2 32 Application Indicate how the parameters are affected in obstructive versus restrictive disease Obstructive Disease FVC FEV1 FEV1/FVC Ratio FEF25-75% RV FRC TLC Restrictive Disease Sample PFT 35 36 37 38 39 40 1. Most of the CO2 transported in the blood is: A. Dissolved in plasma B. In carbamino compounds formed from plasma proteins C. In carbamino compounds formed from hemoglobin D. Bound to chloride E. In HCO32. Which of the following has the greatest effect on the ability of blood to transport oxygen? A. Capacity of the blood to dissolve oxygen B. Amount of hemoglobin in the blood C. pH of plasma D. CO2 content of red blood cells E. Temperature of the blood 3. Which of the following should increase the P50 of the oxyhemoglobin dissociation curve? A. Hypercapnia B. Acidosis C. Increased blood levels of 2,3- BPG D. Increased body temperature E. All of the above 41