Virology - Section 5 Slides PDF
Document Details
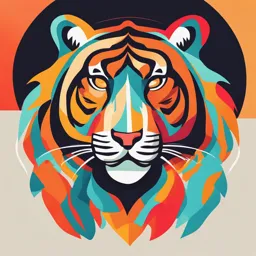
Uploaded by IngeniousCentaur3308
Tags
Summary
These slides cover virology, including chapters 5, 11, 30, and 31. They detail viruses as obligate intracellular parasites/symbionts, their life cycle stages, shapes and structures, and replication methods. The document also discusses virus classification, origins, diversity, and different types of viruses which infect various hosts.
Full Transcript
Virology Chapters 5, 11, 30, 31 Viruses obligate intracellular parasites/symbionts infectious agents that require host cells for replication 1 or more nucleic acid molecule(s) make up the genome no inherent metabolism, but greatly affect metabolism inside the host cell masters of genetic “ec...
Virology Chapters 5, 11, 30, 31 Viruses obligate intracellular parasites/symbionts infectious agents that require host cells for replication 1 or more nucleic acid molecule(s) make up the genome no inherent metabolism, but greatly affect metabolism inside the host cell masters of genetic “economy” – maximize functionality with limited genetic material aent as couldhave enes everg ↳ States of Viruses Extracellular: inactive state > - particle is called a virion capsid (usually protein) surrounds nucleic acid some have phospholipid envelopes outer layer important for protection of genome but also recognition of host cell Intracellular: state Are genome released inside the cell (usually) virus exists as nucleic acid (different viruses have different types) some can remain “silent” inside host cells for long periods ↳ could be millions of. yrs Origins of Viruses Hypothesized that viruses originated within primitive RNA-based cells - “virocells” - and then became - capable of moving from one cell to another as virions Origins of Viruses Did viruses invent DNA? Only viruses have the enzyme that can convert RNA to DNA Viruses are known that contain DNA with uracil (U, usually only found in RNA) Maybe infection by a virus allowed conversion of cellular genomes from RNA to DNA? Virus classification/systematics viruses can be categorized in many different ways type of genetic material hosts, or kinds of cells infected nature of capsid size and shape of virion presence or absence of envelope Genetic Material of Viruses may be DNA or RNA doubranded stranded sing dsDNA, ssDNA, dsRNA, ssRNA (even a combination of both ds and ssDNA in one genome) seem some viruses transition between RNA and DNA forms can be linear or circular single molecule or multiple pieces/segments Genetic Material of Viruses Viral genomes are typically small, but they vary by >3 orders of magnitude Can be very small, only a few thousand nucleotides Can also be larger than bacterial genomes Classification of Viruses Baltimore classification: 7 classes based on genome structure Class I and VII have same genome structure but have important differences in their replication that put them in different classes Same with classes IV and VI Viral diversity every cellular organism on the planet is infected by viruses viruses are the most diverse of all forms of life on the planet Viral diversity for every host species, there are many different types of viruses 7 of these are different bacteriophages (= bacterium eaters) that infect E. coli humans are infected by 10 of the types of viruses in this figure Viral diversity the distribution of types of viruses infecting different hosts is very different Archaea: only DNA viruses known Bacteria: dominated by viruses with dsDNA genomes Eukarya: more different types, with RNA viruses being very common Viral structures there are many different viral shapes and structures Naked vs. Enveloped Viruses nucleocapsid: complex of nucleic acid and protein enveloped viruses have a membrane outside the nucelocapsid The Viral Envelope phospolipid bilayer usually comes from host membrane and wrapped around the nucleocapsid as the virus leaves the cell (but there are exceptions to this) envelope contains proteins that are encoded by virus The Viral Envelope Ebola virus red = envelope proteins orange = lipid bilayer green = proteins associated with inner surface of membrane blue/purple = nucleocapsid Helical Virus Structures TMV, tobacco mosaic virus first virus discovered a very simple structure many copies of a single protein coating the RNA genome - forms a tight helical structure will spontaneously form virions if mix the RNA and protein together in a tube Icosahedral Structures icosahedraon: structure with 20 faces, particles appear spherical very efficient way to make a capsid because it uses the fewest protein subunits possible (smallest = 60 proteins in capsid) e.g. human papilloma virus Tailed viruses some viruses have very complex structures tailed phages are the most common structure found for viruses that infect bacteria e.g. E. coli phage T4: >20 different proteins to make the tail + also several head proteins E. coli phage l Observing and counting viruses viruses do not grow independently and form colonies frequently counted by their detrimental effects on the host zones of lysis or growth inhibition in lawn of host cells - called plaques caused by an original infection of 1 cell by 1 virus, and the viruses produced keep infecting neighbouring cells count the “plaque-forming units” (PFU) Quantification by Plaque Assay mix host cells and viruses to be counted in melted agar and pour mixture onto the surface of a plate Quantification by Plaque Assay this plate has ~50 plaques, so the original mixture had ~50 infectious virus particles Plaques assays in cell culture can do the same with animal and plant viruses if you can grow them in cultured cells Observing and counting viruses some DNA-containing viruses can be counted using fluorescence microscopy possible with the development of new chemicals/stains that bind to DNA and fluoresce very brightly led to the realization that viruses are very abundant in natural environments e.g. 106 viruses per ml in the ocean, 108 per gram of soil Lytic Replication lytic cycle results in death (lysis) of host after replication of the virus particles, the host cell is lysed to release viruses distinct stages in viral infection Burst Size = number of viruses released per cell (e.g., this figure shows a burst size of 4) Attachment and Entry virus-host specificity is partially controlled by virus attachment to receptors on the surface of the cell different viruses use different receptors: can be proteins, glycoproteins, lipids, lipoproteins, LPS, etc. a cell with the appropriate receptor for virus entry is susceptible after entry, virus can replicate only if the cell is also permissive Attachment and Entry after attachment, nucleic acid needs to get inside the cell viruses infecting hosts with cell walls have special challenges for getting in tailed phages are common in bacteria because the tail can help them get the DNA across the cell wall e.g., phage T4 has a lysozyme enzyme at the bottom of its tail that makes a hole in the peptidoglycan Attachment and Entry some viruses only inject nucleic acid ener others also inject proteins me others enter the cell completely and the nucleic acid is & uncoated inside the cell adeared - eic inside e.g., retroviruses: envelope fuses with cell’s membrane & to release the inner “core” isholipid layer T core contains the genome (RNA) and several enzymes needed after infection Lytic replication and the virus growth curve just like for prokaryotic cells growing in a batch culture, there are distinct phases in the growth curve of a lytic virus in a is culture of cells 4like a Curve" lysis and virus release as a single large event produces a “one-step growth curve” structure, which is common for lytic viruses in Bacteria and Archaea - Lytic replication and the virus growth curve eclipse phase: after entry of the viral genome, virus begins process of replication, nucleic acid is free within the cells and non-infectious if released from the cell attachment and nucleic entry See Exist only in no infectious viruses are present anywhere during the eclipse period - Lytic replication and the virus growth curve maturation phase: assembly of new virus particles from replicated nucleic acids and new capsid structures there is a short period of time when there are infectious particles inside the - - cells, but none outside the cells see -increasing #s of infectious particles outside the cells after they begin to break open to release the new virions - Lytic replication and the virus growth curve latent period: no infectious particles OUTSIDE of cells - * includes the eclipse phase - and the first part of the - maturation phase, before - the cells begin to lyse - most lytic phages have proteins that act as molecular clocks to synchronize ~ the time of lysis with completion of new particle assembly Animal viruses unlike for Bacteria and Archaea, there is not a particular bias towards any one type of genome structure in animal viruses enveloped viruses are very common Human viral diseases We mostly think about our interactions with viruses as negative because some viruses cause disease The human virome ~5-10% of our genome is made up of viral genes We are continually infected by viruses that do not (usually) cause any signs of disease (e.g., anelloviruses, adenoviruses) Our intestines are full of viruses that came in with our food The bacteria in our intestines are infected by phages Animal virus replication eukaryotic cells: some viruses replicate in the nucleus, some in the cytoplasm - - differences in viral genetic complexity affect how much of the cell’s machinery is needed for their life cycle some enter the host genome and become a - provirus E - - S can have other effects on the host, such as - assymptimatic causing cancer be ↳ ex · symptomatic or could Effects of animal viruses Transformation: infection converts the cell into a tumor cell - Lysis: infected cell lyses and - releases progeny viruses - Persistent infection: infected cell continually - - releases viruses but doesn’t lyse ↳ doesn't die Tumor viruses > Cancer - some viruses can cause tumors in the organisms they infect - e.g. polyomavirus SV40 (from monkeys) small circular dsDNA genome if the virus infects a - permissive cell, replication suitable for virus replication proceeds as normal if the virus infects a non-permissive cell, it can result in transformation virus integrates into host cell genome and produces proteins that “transform” the cell into a tumor cell /antigens Effects of animal viruses Latent infection: virus is inside - the cell but not actively - replicating some viruses integrate into host genome, others remain as independent nucleic acids Latent viruses some viruses stay dormant for long periods of time and then - get (re)activated to cause an outburst of disease often triggered by stress or modulation of immune system - important feature of herpes viruses (“herpes is forever”): cold sores, caused by Herpes Simplex Virus 1 shingles is caused by the chickenpox virus (varicella zoster is virus, VZV) when it gets reactivated later in life and causes a new Animal RNA viruses We will focus on 4 types of RNA viruses that infect animals/humans: examples * Not expected Retroviruses (HIV) – Baltimore class VI to remember Baltimore Class Picornaviruses (poliovirus) – Baltimore class IV Coronaviruses (SARS-CoV2) – Baltimore class IV Orthomyxoviruses (influenza virus) – Baltimore class V RNA Virus Nucleic Acid Synthesis How do RNA viruses make mRNA and replicate their genomes? RNA viruses produce a special enzyme that is not made by cells: RNA-dependent RNA polymerase (RdRp) RNA Virus Nucleic Acid Synthesis /Iv some RNA viruses need to package RdRp in the capsid with the genome because the genomic RNA cannot act as a mRNA after infection genome = mRNA only for class IV +ve sense ssRNA viruses Retroviruses – breaking all the rules - Retroviruses have +ve sense ssRNA genomes, but it cannot be used as mRNA dsDNA RNA needs to be converted to DNA and then mRNA is produced from the DNA version after integration into the host genome Retroviruses first viruses discovered that cause cancer can be oncogenic or m r ~ immunosuppressive includes Human Immunodeficiency Virus (HIV) that causes AIDS (Acquired Immunodeficiency Syndrome) 40-year long global pandemic: >80 million infected >35 million deaths transmitted via sex or blood (e.g., contaminated needles) Retroviruses – breaking all the rules characterization of nucleic acid processing in retroviruses led to the discovery of reverse transcriptase, which overturned our understanding of information flow in biology retroviruses convert their RNA genome to DNA after entering the cell cannot produce any proteins from the RNA genome – mRNA is transcribed from the dsDNA version integrated into the host cell’s genome Retroviruses – breaking all the rules characterization of nucleic acid processing in retroviruses led to the discovery of reverse transcriptase, which overturned our understanding of information flow in biology scientists found evidence the virus was being passed through cell divisions as part of the cell’s genetic material – how was this possible when the virus had an RNA genome? Reverse transcriptase has 3 distinct enzymatic activities: 1. RNA-dependent DNA polymerase (makes ssDNA from ssRNA) 2. DNA-dependent DNA polymerase (makes dsDNA from the ssDNA) 3. Ribonuclease (degrades the original genomic ssRNA after it has been copied to DNA) Retrovirus virions virus particle contains 2 copies of the +ve ssRNA genome and the enzymes needed for initial stage of infection: reverse transcriptase to make the dsDNA version and integrase: recombines the dsDNA version of the genome into the host’s genome Reverse transcription conversion of the ssRNA into dsDNA is a very complicated process requires all 3 enzymatic functions of the RT a tRNA is used as the primer for starting DNA synthesis all takes place in the cytoplasm within the core structure from the virion – the genomic RNA is never released out of the core in the cell Retrovirus gene expression integration into the host cell’s genome (by the viral integrase) can happen ~anywhere in the genome viral mRNA made from the provirus, transcribed by cellular RNA polymerase sequences at the end of the provirus act as a strong promoter for transcription, so integration of retroviruses can affect expression of neighbouring host genes, which can lead to cancer depending on the genes affected Retrovirus genomes all retroviruses have at least these 3 genes: gag: structural proteins and protease pol: RT and integrase env: envelope proteins also have terminal repeats that are essential for genome replication Retrovirus protein synthesis all retroviruses have at least the 3 genes gag, pol, env transcribed together on a mRNA (not normal for eukaryotic mRNAs) otherwise, the mRNA has “typical” structures: cap and poly-A tail Retrovirus protein synthesis 3 genes: gag, pol, env but they make more than 3 proteins: each gene encodes more than 1 protein first translated as polyproteins that are processed into the individual mature proteins protein processing done by a virus protease that is also part of the GAG polyprotein - cuts itself out of the polyprotein and then cuts up the rest (protease is a target for anti-HIV drugs) Retrovirus protein synthesis there is a stop codon between gag and pol genes (*) ribosome sometimes translates through the stop codon to make a GAG-POL double polyprotein not every time, so GAG>>GAG-POL: more of the capsid proteins made, which they need more of this then gets cut into the individual protein units Retrovirus protein synthesis ENV protein is not translated from the original mRNA, but from a processed shorter version the original ENV polyprotein is also processed to produce the 2 envelope proteins Release by budding many animal enveloped viruses get released by budding, including retroviruses nucleocapsid is assembled inside the cell and then wrapped in the envelope, containing the envelope proteins, as it is released from cell Picornaviruses viral family called Picornaviridae (pico=small and they have RNA genomes) contains many viruses that infect people: e.g., poliovirus, rhinoviruses, hepatitis A, enterovirus D68 +ve ssRNA genomes ~25 nm in size icosahedral structure Poliovirus poliovirus was once endemic in the human population most infections affect only the gastrointestinal tract and the virus is shed in feces and spreads by contact with contaminated feces some infected individuals can develop poliomyelitis (1/200), which affects the nervous system and can result in paralysis currently a world-wide effort to eradicate poliovirus, but there are several hurdles: opposition to vaccines use of live attenuated virus for some vaccines leads to vaccine- derived outbreaks live oral vaccine: vaccinated individuals release virus in their feces and the high mutation rate of this virus changes some of the viruses back to the virulent form – these can then cause new outbreaks of poliomyelitis currently in North America only the inactivated virus vaccine is used http://polioeradication.org/news-post/the-two-polio-vaccines/ every October 24th is “world polio day” Global Polio Eradication Initiative: a huge global effort to completely eradicate polio, started in 1988 >$15 billion spent so far polio cases are down by 99.9% since started down to 22 cases (wild polio) in 2017 and in only 2 countries, but was >500 in 2020 and 2021 and in >30 countries current situation variant poliovirus is derived from the live vaccine strain Picornaviruses poliovirus genome is +ve ssRNA and contains only 1 gene (~8,000 bases) the viral genome has a protein covalently attached the 5’ end that is required for translation Picornaviruses translation produces a single polyprotein and then protease proteins within this polyprotein cut themselves out and cut up the polyprotein into the individual proteins Picornaviruses synthesis of more +ve strands (mRNAs) and replication of the genome for packaging into new particles is done by the viral RNA replicase (=RNA- dependent RNA polymerase, RdRp) because the RNA that is packaged inside the capsids can be translated after entering the host cell, these viruses do not need to package RdRp in the virions Picornaviruses the RNA has the Vpg protein attached at the 5’ end for initiating translation, and this means it does not need the cellular cap-dependent translation machinery of the cell, which is the mechanism used for translating most cellular mRNAs in addition to cleaving the viral polyprotein, the viral proteases degrade the cell’s proteins used for translating capped mRNAs allows all of the cell’s resources to be directed to producing the viral proteins has a b Coronaviruses Envelope proteins o corona = crown particles are enveloped +ve ssRNA genome largest known RNA genomes (~30,000 bases) several coronaviruses regularly circulate in humans, causing mostly mild disease (e.g., common cold) Early 2000's Severe Acute Respiratory Syndrome (SARS-CoV1), Middle East - Respiratory Syndrome (MERS), -COVID-19 (SARS-CoV2) are all O examples of emerging zoonotic diseases - ↳ not from humans 6 "New " Coronaviruses SARS-CoV1 emerged in 2003 in China and then spread to ~30 different countries, including Canada ~8000 infected and ~800 deaths primary reservoir = bats, transmitted to humans via an intermediate host (palm civets) SARS-CoV2 emerged in 2019 in China and then spread to the entire world primary reservoir = bats, transmitted to humans via an intermediate host (several possibilities) Coronaviruses even though they also have +ve ssRNA genomes, they use a different approach for gene expression compared with picornaviruses and retroviruses spike protein interacts with receptor - on cell and membranes fuse - released RNA genome is capped and can act as mRNA directly after infection, but only for translation of 1 - protein: the replicase protein (RdRp), - which is encoded at 5’ end of the genome Coronaviruses replicase protein then makes a - ve sense copy of the genome -ve strand then used to replicate more of the +ve strands and synthesize monocistronic mRNAs for expression of the other proteins capped monocistronic mRNAs no polyprotein virus is assembled inside the cell, including the envelope (does not bud out and get envelope at CM like retroviruses) Influenza A viruses enveloped, ~60-100 nm diameter -ve sense ssRNA, segmented genome & then piece more of RNA one 8 segments: total ~13,000 bases 8 segments = 8 different pieces of RNA in each viral particle to make up the whole genome, each makes different proteins Replication of –ve ssRNA viruses particles must contain RdRp to make +ve sense versions of all the segments to be used as mRNAs RNA RNA dependent polymerase Influenza A viruses each RNA segment is bound by proteins and has the replicase/RdRp attached (complex of 3 proteins: PB2, PB1 and PA) Influenza A viruses & envelope proteins viral release by assembly at the membrane and budding the virus enters into the host cell in a vesicle and then the virion components are released into the cytoplasm (fusion and uncoating does not happen at the CM like we saw for retros and coronas) the viral RNAs (along with the attached RdRp) go to the nucleus for mRNA synthesis and replication Influenza A viruses (IAVs) IAVs infect a wide range of species - mammals and birds in birds it usually causes a gastrointestinal disease, transmitted via feces in humans it is a respiratory illness, infection through inhalation IAVs are originally from birds, but they have become endemic - in humans and human strains circulate in the population with yearly epidemics around the world occasionally, get the evolution of new strains that are especially dangerous (for birds and/or humans) “The flu” versus a “cold” getting the true “flu” (= infection by influenza virus) is ~7X less common than getting infected by viruses that cause a “common cold” IAV evolution influenza viruses evolve by 2 distinct processes: -u antigenic drift antigenic shift s in replication mutation normal antigenic drift: changes in genome sequence caused by mutations introduced during replication (error-prone replicase enzyme) RdRp enzyme has a high error - rate and does not correct - - mistakes - IAV envelope proteins IAV strains are named based on their envelope proteins: hemagglutinin (HA) neuraminidase (NA) e.g., H1N1, H3N2, H2N7 there are 18 H subtypes and - 11 N subtypes - for humans, antigenic drift is most important for changes that happen to HA, which is the major target of the immune system changes in that protein can allow viruses to re-infect people who ne were immune to a previous version - IAV vaccines only 2 strains of IAV currently circulate in humans: H1N1 and H3N2 mixtures of viruses put into the vaccine changed every year to match what is (expected to be) circulating in humans you need to get re-vaccinated every year to ensure the best chance of not getting infected also: to prevent infection by IAV, you need to have high levels of antibodies circulating in your blood and this decreases over time after vaccination so the annual shot also serves to boost these levels IAV evolution influenza viruses evolve by 2 distinct processes: antigenic drift antigenic shift a Evendramin more antigenic shift: caused by new reassortment of segments (mixed packaging) after co-infection of a cell by 2 different strains reassortment can generate viruses with very different properties compared to the parental viruses IAV evolution some of the most important IAV evolutionary events have occurred from reassortment that involved viruses from different host species e.g. bird and human, bird and pig, or all 3 bird viruses usually don’t infect people and vice-versa, but pigs are readily infected with human and bird viruses and are thought to be a frequent “mixing vessel” for generating new strains Influenza pandemics & influenza circulates around the globe every year every 10-40 years, we get an influenza pandemic (= world-wide epidemic) all pandemic viruses that have been characterized have contained at least some portion of genes from bird IAVs 1918 “Spanish flu” - estimated - to have killed ~50 million people may have originated in the US? spread across US in ~3 weeks was most deadly for young and - healthy people - Influenza pandemics 1918 “Spanish flu” - estimated to have killed ~50 million people the virus has been resurrected: scientists dug up dead bodies from 1918 that had been buried in permafrost in Alaska - ↳ perminately frozen ground determined the genome sequence and recreated the virus in the lab Body's never decomposed so they recovered the RNASequence Drs. Jeff Taubenberger (the boss) and Ann Reid (one who did the lab work to sequence the 1918 virus) 2009 pandemic: “swine flu” seasonal influenza usually shows a predictable pattern of spread across the globe (in part due to climate): starts in Asia and works it’s way west through Europe and Africa, then to North America and finally South America – so, our flu season is late fall-early winter 2009 pandemic virus showed very different timing of emergence: first outbreak in humans happened in Mexico and USA in spring 2009 and then the pandemic was full-on by the fall e more likelytodiefromSeasona this strain was very transmissible but not very lethal (less % deaths compared to a “typical” seasonal non-pandemic strain) we were VERY LUCKY that this virus did not have the same properties as the 1918 virus! “Bird flu” there have also been a few different bird IAVs over the past bunch of years that have infected people e.g. H5N1, H7N9, H10N8 strains an H5N1 strain appeared in the 1990s in birds in Asia, resulted in the deaths of millions of domestic birds (poultry), and has been transmitted to at least ~600 people still present in birds and causing human cases seems very lethal (possibly ~50% death rate) but does not transmit from human to human concern that it will gain human transmission capability or reassort with another virus to generate a virulent pandemic strain “Bird flu” a huge problem for agriculture 2014-2015 “Bird flu” a huge problem for agriculture and wild birds 2021-2022 birds were sick and dying at a farm in St. John’s: ~350 died and then the remaining ~60 were killed subsequently found in wild birds in the area then found in mainland Canada and the USA - in wild birds and farm outbreaks “Bird flu” a huge problem for agriculture and wild birds 2021-now farm outbreaks essentially impossible to know how many wild birds have been killed but it is in the 10s of thousands just in Canada Animal RNA virus comparisons Virus genome mRNA proteins only made from provirus, Retroviruses +ve ssRNA need RT + INT in capsid, polyproteins, cleaved (HIV; enveloped) splicing to make env by internal protease mRNA Picornaviruses (polio; +ve ssRNA protein attached to 5’ end, polyprotein, cleaved not enveloped) genomic RNA=mRNA by internal protease capped, genomic Coronaviruses +ve ssRNA RNA=mRNA for RdRp 1 per mRNA (SARS; enveloped) only, others monocistronic Orthomyxoviruses -ve ssRNA, RdRp in capsid, attached (influenza A; segmented to each segment 1 mRNA per segment enveloped) “Bird flu” a huge problem for agriculture and wild birds 2021-today farm outbreaks essentially impossible to know how many wild birds have been killed but it is in the 10s of thousands just in Canada Viruses of protists protist viruses have been discovered that are similar to some of the known animal viruses, e.g. picornaviruses also some very different viruses, such as mimiviruses Mimivirus: was mistaken for a bacterium inside its host amoeba “mimicking microbe” virions are ~0.5 um wide (~1/2 the width of a ”typical” bacterial cell genome is 1.2 Mb, larger than some bacterial genomes Viruses of protists discovery of mimivirus was another changing moment in virology: viruses could have more genetic complexity than cells! more recently, Pandoravirus was discovered (also infects amoebae) genome is >2X larger than Mimivirus some scientists have used these “giant viruses” to argue that they represent a 4th domain of life Viruses of protists “virophages” are another interesting discovery that came from studying these giant viruses small “viruses” that require the host cell to be infected by a giant virus because they use its machinery (VF=virus factory) for their replication interfere with the giant virus’ replication, reducing its production of new virions a virus that ”infects” another virus Phage diversity for every host species, there are many different types of viruses 7 of these are some of the different phages that infect E. coli “bacteriophage” = bacterium eater tailed phages with dsDNA genomes are the most common type Phage T4: lytic replication a model lytic virus - replicates and lyses E. coli host cells in ~25 minutes relatively large genome (for phages, ~170,000 bp) and contains many genes (~250) completely reprograms infected cell to devote all resources to phage replication Phage T4: lytic replication Interacts with LPS as the receptor and contraction of the tail pushes an inner tail tube structure through the outer membrane Lysozyme enzyme on the end of this structure chews a hole through the peptidoglycan and DNA enters into the cytoplasm Phage T4: lytic replication within 1 minute of DNA injection, host DNA and RNA synthesis have been completely stopped and all of the cell’s resources are redirected to T4 replication regulated, temporal gene expression pattern – early, middle and late genes with different types of functions, and then cell lysis and release of new particles Phage T4: lytic replication early: host cell takeover middle: DNA replication late: new virus particles Phage T4 DNA structure and replication genome in virus particles is linear dsDNA, but each particle contains more than one entire genome and therefore has terminal repeats DNA from 1 phage T4 particle: complete genome genes 1 2 3 4 5 6 7 8 9 10 11 12 13 1 2 terminal repeats Phage T4 DNA structure and replication each virus particle contains a slightly different piece of DNA inside capsid 1 inside capsid 2 genes genes 1 2 3 4 5 6 7 8 9 10 11 12 13 1 2 5 6 7 8 9 10 11 12 13 1 2 3 4 5 6 called “circularly permuted” because if you sequence the 13 1 2 genome using DNA collected from 12 3 many different individual virus 11 4 5 particles the sequence and genetic 10 9 6 map comes together as a circle – 8 7 there is no single start or end Phage T4 DNA replication and packaging these features arise because of the way the DNA is replicated and packaged: 1. replicates many copies of the DNA, but cannot make the ends double- stranded (the “end problem”) 2. recombines these individual copies together to make a concatemer 3. performs headful packaging from the concatemer and each head holds more than 1 genome length of DNA Phage T4 DNA packaging DNA is packaged into a partially assembled structure there is a packaging motor, which requires energy (ATP) to “pump” the DNA into the head on Education, Inc. DNA enters through a specific structure called the “portal” Phage T4 DNA packaging DNA is packaged into a partially assembled structure there is a packaging motor, which requires energy (ATP) to “pump” the DNA into the head motor leaves after DNA packaging is completed and on Education, Inc. the structure is finished by adding the tail, etc. Some phage T4 tricks T4 DNA contains an unusual base, 5- hydroxymethylcytosine, which makes the DNA resistant to restriction enzymes many Bacteria encode restriction enzymes that cut up foreign DNA as a defense against viral infection as part of its early takeover of the host cell, T4 produces nucleases that break the cell’s genome into nucleotides that it uses for replication of its DNA Phage replication strategies Phage T4 is only capable of lytic replication, but temperate phages can choose between lytic and lysogenic pathways lysogenic infection = phage genome becomes part of the host genome phage genome is passed to daughter cells during replication and division phage can enter into lytic pathway later (= induction) Immunity and phage conversion Integrated temperate phage is called a prophage Cell is called a lysogen (because these cells can “generate lysis” later) and is immune to infection by the same virus some viruses carry genes that modify the host’s phenotype = phage conversion e.g. toxins, virulence genes Phage Lambda (l) another tailed phage that infects E. coli genome ~48,000 bp (~1/3 the size of the T4 genome) DNA in the virus particles is linear dsDNA not circularly permuted, no terminal repeats every particle contains the exact same piece of DNA Phage Lambda (l) has 12 nucleotide single- stranded sequences on the ends cohesive ends: complementary and come together inside the cell and the genome forms a circle then it senses the condition of the host cell and makes a “decision”: things good for replication? = lytic things not so good for replication? = lysogenic Lambda: temperate infection lysogeny: replication genes are not expressed, and the DNA integrates into the cell’s genome integration done by lambda integrase protein happens by recombination between specific sequences called att sites (for attachment) attP (phage) and attB (bacterium) Induction the integrated prophage can then be induced for the lytic pathway later, when the virus senses it is a good time to replicate so, prophages are like time-bombs sitting in the genome because if they get induced the cell will be killed however, prophages can also provide beneficial functions to the host cells, such as immunity to related phages, toxin genes, etc. and some prophages stay in the “silent” lysogenic state for millions of years Lambda DNA replication and packaging DNA is replicated by “rolling circle replication”, which generates a long concatemer packages DNA from this concatemer, but unlike T4, lambda does not use headful packaging packages everything between specific sites in the genome, cos (= the cohesive end sites) called pac site packaging Lambda DNA replication and packaging for phages that use pac site packaging: genome is not circularly permuted no terminal repeats every virion contains the exact same piece of DNA Lambda: specialized transduction lambda always integrates in the same location in the E. coli genome, between the gal and bio genes if it makes a mistake during induction and brings the sequences from these genes along, the virions can then perform specialized transduction and transfer the gal or bio genes to a subsequent host cell dsDNA tailed phage comparisons Phage genome DNA replication packaging T4 linear dsDNA, terminal recombination of headful (lytic) repeats, nearly complete circularly permuted, linear genomes into each particle has different concatemer start and end Lambda linear dsDNA, lytic pathway in pac site (temperate) cos ends, circular form; rolling all particles contain the circle to produce same DNA (except concatemer transducing) Phage therapy phages were independently discovered in 1915-1917 by Frederick Twort and Felix d’Herelle (Canadian) even though he could not see the phages to know what they were, d’Herelle saw a potential value of this “thing” that was killing the bacteria so, he tried to use the phages to treat bacterial infections (tested it on his own child!) = phage therapy Phage therapy >100 years later scientists are still working to use phages as treatments for some bacterial infections has shown promise for some diseases, but also has some problems: some phages carry genes that make bacteria more pathogenic, including toxins immune reaction against the phage? lysis of bacteria can release toxins (e.g., endotoxin) into the body Phage therapy phages used for the Mycobacterium infection were isolated by undergraduate students Viruses in Archaea some viruses that have been found in Archaea are like the dsDNA tailed viruses in Bacteria such as T4 - they look similar and replicate in similar way however, most archaeal viruses are completely different than anything ever found in Bacteria: very different morphologies most are released from host cells without lysis like in Bacteria, most viruses of Archaea have genomes made of linear dsDNA environments where archaea are the dominant organisms (e.g. acidic hot springs) are full of archaeal viruses Anti-viral defenses: CRISPR-Cas one way bacteria (and archaea) can fight off phage infection is with CRISPR-Cas systems + host cells mix cells with a lytic phage and put onto an agar plate: almost all cells killed but some survive and form colonies on the plate = bacteriophage-insensitive mutants (BIMs) researchers (Canadian!) studied these BIMs and found they contained extra DNA sequences in their CRISPR-Cas regions, and the extra DNA matched sequences from the phage they were now resistant to Anti-viral defenses: CRISPR-Cas CRISPR: Clustered Regularly Interspaced Short Palindromic Repeats Cas: CRISPR-associated (genes that encode proteins) Immunization: some of the cells pick up a piece of the phage DNA and add it into their CRISPR region done by Cas proteins most likely to happen if the cell gets infected by a defective phage (has a genetic flaw so that its DNA gets into the cell, but it cannot actually replicate) Anti-viral defenses: CRISPR-Cas CRISPR: Clustered Regularly Interspaced Short Palindromic Repeats Cas: CRISPR-associated (genes that encode proteins) Interference: the CRISPR sequences are transcribed to RNA and these short RNAs target the Cas proteins to the matching phage DNA, and it gets cut open at that location and phage is inactivated CRISPR-Cas and genome editing This is now being used as a tool to make specific changes to genes and genomes Design an RNA that matches the desired target gene and will also be recognized by the Cas protein Cas protein cuts the DNA at that location Add new DNA with some matching overlapping sequences to allow recombination to occur: modified genome! CRISPR-Cas and genome editing modification of pig genome so the animals are more lean so the meat is more healthy modification of removal of endogenous human genome retroviruses from pig to cure sickle genome to improve cell disease pigs as sources of organs for human transplants