IT438 Communication Technology PDF
Document Details
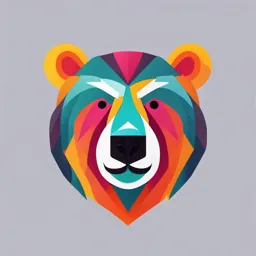
Uploaded by CrisperPixie
EELU
2024
AQA
Kamal Hamza, PhD
Tags
Related
- E-Book : Information And Communication Technology (English).pdf
- Information and Communication Technology (PDF) - SRI VENKATESWARA UNIVERSITY, 2024-2025
- Information and Communication Technology Lesson 1 PDF
- Communication Technology Section 2 PDF
- IT438 Communication Technology Lecture Notes PDF
- IT438 Communication Technology Lecture Notes PDF
Summary
These are lecture notes for the IT438 Communication Technology course in the Fall semester 2024-2025. The notes cover introductory concepts and different issues related to communication systems.
Full Transcript
Fall Semester 2024-2025 IT438 Communication Technology Kamal Hamza, PhD Acknowledgement: This presentation contains some figures and text from Data Communications...
Fall Semester 2024-2025 IT438 Communication Technology Kamal Hamza, PhD Acknowledgement: This presentation contains some figures and text from Data Communications and Networks book by W. Stallings Introduction Communication – Basic process of exchanging information from one location (source) to destination (receiving end). Refers to the process of sending, receiving and processing of information/signal/input from one point to another point. IT438 Communication Technology 1 Communication Systems Components Any communication system consists of three basic blocks: Transmitter Receiver Communication Channel Source Transmitter Channel Receiver Recipient A transmitter prepares the data (information) to be transmitted in the appropriate format in order to be transmitted over the communication channel. IT438 Communication Technology 2 Communication System Components (cont.) Transmitter Receiver IT438 Communication Technology 3 Communication System Components (cont.) Information Source Generates the message(s). Examples are voice, pictures, computer key board, etc.. If the message is not electrical, a transducer is used to convert it into an electrical signal. Source can be analog or digital. IT438 Communication Technology 4 Communication System Components (cont.) Source encoder/decoder The source encoder maps the signal produced by the source into a digital form. The mapping is done so as to remove redundancy in the output signal and also to represent the original signal as efficiency as possible (using as few bits as possible). The mapping must be such that an inverse operation (source decoding) can be easily done. If the message is a voice signal, the source encoder would convert the analog voice signal into a digital form (binary data) and compress it (e.g., MP3 compression for audio). Focus: Efficient representation of the message, removing unnecessary bits. IT438 Communication Technology 5 Communication System Components (cont.) Channel encoder/decoder Maps the input digital signal into another digital signal in such a way that the noise will be minimized. When transmitting binary data, the channel encoder could add extra bits (redundancy) that help detect and correct errors. Focus on Error detection and correction to ensure reliable transmission over noisy channels. Modulator Modulation provides for efficient transmission of the signal over channel. Most modulation schemes impress the information on either the amplitude, phase or frequency of a sinusoid. IT438 Communication Technology 6 Examples of Guided Comm. Channels IT438 Communication Technology 7 8 Examples of Unguided Comm. Channels IT438 Communication Technology 8 9 Why Different Types of Comm. Systems? There are several factors that give rise to the need for different types of communication systems: The nature of the communication channel (undersea communication requires optical fiber cables) The nature of the application (mobile applications needs wireless systems) Required level of quality (performance and quality of the received signal) Cost IT438 Communication Technology 9 Problems that Face Comm. Systems Any communication system can be subject to three main sources of problems: Noise: undesired effect from the communication environment. Usually, it is not under our control (your system has to deal with it). Interference: due to superposition of two or more signals. May result from bad design of communication systems (interference of voice channels in telephone systems, for example) Jamming: intentional interference that aims at destroying the quality of the transmitted signal to prevent transmission. IT438 Communication Technology 10 Problems that Face Comm. Systems (cont.) IT438 Communication Technology 11 Problems that Face Comm. Systems (cont.) IT438 Communication Technology 12 Data versus Signal Differentiate between two terms: data (information) and signal. Data (information): are generated by the application and need to be transmitted to some receiver. Signal: is the representation of the data in the communication system. Data are generated from the source (application) and signals are generated from the transmitter of the communication system we build. Speaking in a microphone: data (information) is what I say, whereas signal is what moves inside the wire of the microphone (electricity). IT438 Communication Technology 13 Types of Data and Signal Depending on the application nature, we have four possible combinations of data and signals: Analog Data, Analog Signal: Traditional telephone system. Analog Data, Digital Signal: Voice over IP (VoIP), digital music streaming. Digital Data, Analog Signal: Modems (used in early internet connections), Optical networks. Digital Data, Digital Signal: Ethernet, Wi-Fi, modern computer networks. Since any communication system deals with signals, we first need to understand the nature and types of signals. IT438 Communication Technology 14 Signal Representation in the Time Domain Viewed as a function of time, an electromagnetic signal can be either continuous or discrete. A continuous signal is one in which the signal intensity varies in a smooth fashion over time. IT438 Communication Technology 15 Signal Representation in the Time Domain (cont.) A discrete signal is one in which the signal intensity maintains a constant level for some period of time and then changes to another constant level. Discrete Signal IT438 Communication Technology 16 Signal Representation in the Time Domain (cont.) Smoothly rises and falls, following a Sine Wave continuous curve. used to represent analog signals in communication systems. Quickly switches between high and low Square Wave values, creating sharp, rectangular steps. used in digital systems to represent binary data (0s and 1s). IT438 Communication Technology 17 Signal Representation in the Time Domain A sine wave is a fundamental mathematical function that describes a smooth, periodic oscillation. Key Features of a Sine Wave: Amplitude (A): This is the peak value (height) of the wave, representing the maximum displacement of the wave from its central position. Higher amplitude means a stronger or louder. Frequency (f): Frequency refers to how many cycles (complete waves) occur per second, measured in Hertz (Hz). A higher frequency means the wave oscillates faster. IT438 Communication Technology 18 Signal Representation in the Time Domain Period (T): The period is the time it takes to complete one full cycle of the wave. It is the inverse of frequency (T = 1/f). A shorter period means a higher frequency. Phase (φ): Phase refers to the horizontal shift of the wave. If two sine waves have different phases, one wave may start at a different point in its cycle compared to the other. Phase differences are crucial in signal modulation and communication. IT438 Communication Technology 19 Signal Representation in the Time Domain (cont.) Sine Wave Amplitude, Frequency, Phase IT438 Communication Technology 20 Signal Representation in the Time Domain (cont.) Sine Wave (cont.) IT438 Communication Technology 21 Fall Semester 2024-2025 IT438 Communication Technology Kamal Hamza, PhD Acknowledgement: This presentation contains some figures and text from Data Communications [email protected] and Networks book by W. Stallings Signal Representation in the Frequency Domain In practice, an electromagnetic signal will be made up of many frequencies. For example, the signal: is made up sine waves of frequencies f1 and 3f1 The spectrum of a signal is the range of frequencies that it contains. IT438 Communication Technology 22 Signal Representation in the Frequency Domain (cont.) + = IT438 Communication Technology 23 Signal Representation in the Frequency Domain (cont.) Fourier analysis IT438 Communication Technology 24 Signal Representation in the Frequency Domain (cont.) If a signal includes a component of zero frequency, that component is a direct current (dc) or constant component. IT438 Communication Technology 25 Noise and Interference In practical communication systems signals are blurred by noise and interference: Time domain Frequency domain IT438 Communication Technology 26 Signal Bandwidth (cont.) Bandwidth is the difference between the upper and lower frequencies in a continuous band of frequencies. It is typically measured in unit of hertz (symbol Hz). IT438 Communication Technology 27 Signal Bandwidth (cont.) Bandwidth in Different Communication Systems: 1. Telecommunications (Audio): In traditional telephone systems, the bandwidth is typically limited to 300 Hz to 3400 Hz, which is sufficient for transmitting the human voice. This 3.1 kHz bandwidth filters out both very low and very high frequencies. 2. Radio Broadcasts: AM radio stations use bandwidths around 10 kHz per channel, while FM radio uses a much larger bandwidth (around 200 kHz) to transmit better quality sound. IT438 Communication Technology 28 Signal Bandwidth (cont.) Bandwidth in Different Communication Systems (Cont.): 3. Video and TV Transmission: Analog TV signals use bandwidths around 6 MHz. Digital video signals (e.g., HD video) use even more bandwidth, which is why they rely on advanced compression techniques. 4. Wi-Fi and 5G: a) Wi-Fi operates in the 2.4 GHz or 5 GHz frequency bands, with bandwidths typically ranging from 20 MHz to 160 MHz. Higher bandwidth means faster internet speeds. b) 5G technology operates at frequencies between 3 GHz and 100 GHz, with very large bandwidths available (up to several GHz), allowing it to carry massive amounts of data. IT438 Communication Technology 29 Signal Bandwidth (cont.) IT438 Communication Technology 30 Signal Bandwidth (cont.) IT438 Communication Technology 31 Fiber Optics Communication Technology Optical Transmission System Main components of an optical transmission system are: Optical fiber links Transmitters Receivers Amplifiers Network medium IT438 Communication Technology 1 Optical Fiber Links Light propagates by total internal reflection IT438 Communication Technology 2 Optical Fiber Links (cont.) Advantages of fiber optical links: 1. High Bandwidth Capacity Fiber optics can carry more data: Unlike copper cables, fiber optic cables have much higher bandwidth, which means they can transmit more data over longer distances without degradation. Speed: Modern fiber systems can easily handle data rates of 100 Gbps and higher. 2. Low Signal Attenuation (Loss) Long-distance transmission: Signals transmitted over fiber optic cables experience much less loss compared to copper cables. Improved efficiency: fiber optics are particularly suitable for large-scale communication networks, including long-distance telecommunication and undersea cables. IT438 Communication Technology 3 Optical Fiber Links (cont.) Advantages of fiber optical links (cont.): 3. Immunity to Electromagnetic Interference (EMI) Interference-free transmission: Fiber optic cables are immune to electromagnetic interference (EMI) because they transmit light instead of electrical signals. No cross-talk: In copper wires, nearby cables can interfere with each other, causing signal distortion. 4. Security Enhanced data security: Fiber optics offer greater security because it is difficult to tap into or intercept the light signals without disrupting the transmission. Tamper detection: Any attempt to physically tap into a fiber optic cable will cause noticeable disruption to the light transmission, which can be detected easily. IT438 Communication Technology 4 Optical Fiber Links (cont.) Advantages of fiber optical links (cont.): 5. Lightweight and Thin 6. Durability and Longevity 7. Reduced Latency 8. Scalability 9. Environmental Benefits 10. High Reliability IT438 Communication Technology 5 Optical Fiber Links (cont.) Types of optical fiber: 1. Single-mode fiber (SMF) 2. Multimode fiber (MMF) Feature Single-mode Fiber (SMF) Multimode Fiber (MMF) Core Size Small (8-10 microns) Larger (50-62.5 microns) Light Propagation Single light mode (direct path) Multiple light modes (bounces inside the core) Distance Long distances (up to 40 km or more) Short distances (up to 550 meters) Bandwidth Higher bandwidth, supports higher data rates Lower bandwidth, supports moderate data rates Cost More expensive to install and maintain Cheaper, simpler installation Application Long-haul telecom, WAN, high-speed data links LAN, data centers, short-range communication Attenuation/Dispersion Lower attenuation and dispersion Higher attenuation and modal dispersion IT438 Communication Technology 6 Attenuation Attenuation in optical fiber leads to a reduction of the signal power as the signal propagates over some distance. Attenuation (dB)= α × L Where: α = Attenuation coefficient (measured in dB/km). L = Length of the fiber (in kilometers). Attenuation must considered when determining the maximum distance that a signal can propagate. Caused by the fiber material, which absorbs and scatters light energy. IT438 Communication Technology 7 Dispersion Dispersion is the widening of a pulse duration as it travels through a fiber. Interference Different components of the light pulse arrive at the destination at different times. Affects the clarity and timing of the signal, limiting the data rate and causing errors when pulses overlap due to broadening. IT438 Communication Technology 8 Effect of Dispersion As a pulse widens, it can broaden enough to interfere with neighboring pulses (bits) on the fiber, leading to ISI. IT438 Communication Technology 9 Types of Dispersion 1. Modal Dispersion: Occurs in multimode fibers when different light paths (modes) travel at different speeds, causing pulse broadening. Mitigated by single-mode fibers or graded-index fibers. 2. Chromatic Dispersion: Affects both single-mode and multimode fibers as different wavelengths of light travel at different speeds, causing pulse spreading. Mitigated by lasers and dispersion-compensating fibers. IT438 Communication Technology 10 Types of Dispersion (cont.) 3. Polarization Mode Dispersion (PMD): Occurs in single-mode fibers when different polarization states travel at different speeds due to fiber imperfections. Mitigated with high-quality fibers or polarization-maintaining fibers. IT438 Communication Technology 11 Nonlinearities in Fiber Nonlinearities in fiber occur when the light intensity in the fiber becomes high enough to cause the fiber's refractive index to change or to induce other non-linear effects. It may lead to attenuation, distortion, and cross-channel interference. E.g.: Four-Wave Mixing (FWM) Cause: When multiple wavelengths of light interact within the fiber, new wavelengths are generated due to the non-linear mixing of signals. Effect: This creates additional wavelengths that interfere with the original signals, causing crosstalk and signal degradation. IT438 Communication Technology 12 Optical Couplers A coupler is a general term that covers all devices that combine light into or split light out of a fiber. Enabling signal sharing between different channels or users without the need for active electronics. A Splitter (1×N coupler) takes an input signal from one fiber and splits it into N output fibers. A Combiner (N×1 coupler) combines optical signals from multiple input fibers into a single output fiber. IT438 Communication Technology 13 Optical Couplers (cont.) Coupling ratio : describes how the input optical power is divided between the output ports of an optical coupler. Formula for Coupling Ratio: For a simple 1x2 optical coupler, the coupling ratio is defined as: 𝑃1 𝑃2 Coupling Ratio ( ) = 𝑜𝑟 𝑃1 + 𝑃2 𝑃1 + 𝑃2 Where: 𝑃1 = Power at output port 1. 𝑃2 = Power at output port 2. IT438 Communication Technology 14 Optical Couplers (cont.) How to design a 1xN splitter? Couplers (2x1) can be used to design an 8-port splitter. IT438 Communication Technology 15 Fall Semester 2024-2025 IT438 Communication Technology Kamal Hamza, PhD Acknowledgement: This presentation contains some figures and text from Data Communications [email protected] and Networks book by W. Stallings Passive Star Couplers (PSC) Passive star couplers: Optical devices used in fiber optic networks to split or combine optical signals. They are passive because they do not require external power to operate; they rely solely on the physical properties of the fiber to distribute light signals. These devices are commonly used in broadcast-and-select networks, where one signal needs to be distributed to multiple receivers or where multiple signals need to be combined. IT438 Communication Technology 1 Passive Star Couplers (PSC) (cont.) An 8x8 PSC is shown below: IT438 Communication Technology 2 Passive Star Couplers (PSC) (cont.) A 16x16 PSC is shown below: IT438 Communication Technology 3 Passive Star Couplers (PSC) (cont.) In the PSC, the optical power that each output receives Pout equals: where Pin is the optical power introduced into the star by a single node and N is the number of output ports of the star. IT438 Communication Technology 4 1-5 6 Transmitters IT438 Communication Technology 5 1-7 The Laser In order to understand optical transmitters we need to understand the basic concepts of lasers. The word laser is an acronym for Light Amplification by Stimulated Emission of Radiation. Stimulated emission: allows a laser to produce intense high-powered beams of coherent light (light that contains one or more distinct frequencies). IT438 Communication Technology 6 1-8 The Laser (cont.) To understand the concept of stimulated emission, we must get some basic idea on the energy levels of atoms. In each atom, there are a number of discrete levels of energy that an electron can have (states). Stable Atoms: are in the ground state. In such a state, atoms have electrons that are in the lowest possible energy levels. To change the level of an electron in the ground state, the atom must absorb energy. IT438 Communication Technology 7 1-9 The Laser (cont.) When an atom absorbs energy, it becomes excited, and moves to a higher energy level. At this point, the electron is unstable, and usually moves quickly back to the ground state by releasing a photon (a particle of light). Quasi-stable states: the substance can stay in the excited state for longer periods of time. Applying enough energy to a substance in quasi-stable states for a long enough period of time causes population inversion. IT438 Communication Technology 8 1-10 The Laser (cont.) Population inversion: a state which means that there are more electrons in the excited state than in the ground state. Population inversion allows the substance to emit more light than it absorbs. IT438 Communication Technology 9 1-11 The Laser (cont.) Lasing medium is made of a quasi-stable substance. IT438 Communication Technology 10 1-12 The Laser (cont.) Excitation device excites electrons in the lasing medium. When an electron drops back to the ground state it emits a photon of light. The photon will reflect off the mirrors at each end of the cavity, and will pass through the medium again. Stimulated emission occurs when a photon passes very closely to an excited electron. IT438 Communication Technology 11 1-13 The Laser (cont.) The photon may cause the electron to release its energy and return to the ground state: a coherent photon is released. The process continues and the light at the selected frequency builds in intensity. The length of the cavity controls the frequency of the emitted light. IT438 Communication Technology 12 1-14 The Laser (cont.) IT438 Communication Technology 13 1-15 Semiconductor Diode Lasers Very simple and useful for optical communication. Can be simply implemented using a p-n junction with mirrored edges perpendicular to the junction. IT438 Communication Technology 14 1-16 Main Characteristics of Lasers Some of the physical characteristics of lasers which may affect system performance are: laser linewidth, Frequency instability, and the number of longitudinal modes. Laser linewidth: is the spectral width of the light generated be the laser. IT438 Communication Technology 15 1-17 Main Characteristics of Lasers (cont.) Frequency instabilities: are variations in the laser frequency. Important types: mode hopping mode shifts The number of longitudinal modes: is the number of wavelengths that a laser can amplify. Unwanted longitudinal modes may result in significant dispersion. It is desirable to implement lasers which produce only a single longitudinal model. IT438 Communication Technology 16 1-18 Tunable Lasers Transmitters used in Wavelength Division Multiplexing (WDM) networks often require the capability to tune to different wavelengths. Tunability characteristics: Tuning range: Wide and continuous range of over 100 nm Tuning time: Rapid (< ms ranges) Tuning capability: continuously vs. discretely tunable Long lifetime and stable over lifetime Easily controllable and manufacturable IT438 Communication Technology 17 1-19 Types of Tunable Lasers Tunable lasers can be implemented using different methods: Mechanically-Tuned lasers Physically adjust the distance between the two mirrors on either end of the cavity Wide tuning range Slow tuning time (order of ms) Acousto-optic and Electro-optic -Tuned lasers Use either sound waves or electrical current Moderate tuning range Moderate tuning time (order of tens of ns) Injection-Current-Tuned lasers Main drawback: mode hopping IT438 Communication Technology 18 1-20 Types of Tunable Lasers (cont.) IT438 Communication Technology 19 1-21 Laser Arrays Instead of using tunable lasers, one can implement a laser array, which contains a set of fixed-tuned lasers. A laser array consists of a number of lasers which are integrated into a single component, with each laser operating at a different wavelength. The advantage of using a laser array is that, if each of the wavelengths in the array is modulated independently, then multiple transmissions may take place simultaneously. A possible drawback: the number of wavelengths is fixed. IT438 Communication Technology 20 Fall Semester 2024-2025 IT438 Communication Technology Kamal Hamza, PhD Acknowledgement: This presentation contains some figures and text from Data Communications [email protected] and Networks book by W. Stallings 2 Optical Modulation IT438 Communication Technology 1 Optical Modulation Optical modulation is specific to systems that use light waves (usually from lasers or LEDs) as the carrier. It involves altering the properties of light (amplitude, phase, frequency, polarization) to carry information over optical fibers. Common Digital Modulation Techniques in Optical Fiber 1. Amplitude Shift Keying (ASK) / Intensity Modulation (IM) 2. Phase Shift Keying (PSK) 3. Quadrature Amplitude Modulation (QAM) 4. Frequency Shift Keying (FSK) IT438 Communication Technology 2 Optical Modulation 1- Amplitude Shift Keying (ASK) / Intensity Modulation (IM) ASK, often referred to as Intensity Modulation (IM) in optical communication, is the simplest and most widely used technique in optical fiber systems. In IM, the intensity of the light wave (usually from a laser or LED) is modulated to carry data. Binary 1: High intensity (light "on"). Binary 0: Low intensity (light "off"). 2- Phase Shift Keying (PSK) PSK, particularly for long-distance and high-speed transmission. Since PSK uses phase changes to represent data, it can provide more reliable communication over noisy and long optical links. Binary Phase Shift Keying (BPSK): Encodes data using two phases (0° and 180°). Quadrature Phase Shift Keying (QPSK): Encodes data using four phases (0°, 90°, 180°, 270°), allowing two bits per symbol. IT438 Communication Technology 3 Optical Modulation 3- Quadrature Amplitude Modulation (QAM) QAM is increasingly used in high-speed optical communication systems due to its efficiency in transmitting large amounts of data over a given bandwidth. QAM modulates both the amplitude and phase of the light wave to encode multiple bits per symbol. 16-QAM: Transmits 4 bits per symbol (16 amplitude/phase combinations). 64-QAM: Transmits 6 bits per symbol (64 amplitude/phase combinations). 4- Frequency Shift Keying (FSK) FSK can be used in optical systems, but it is not as common as IM or PSK due to its lower spectral efficiency. Minimum Shift Keying (MSK), a variation of FSK, has been implemented in some optical systems for its bandwidth efficiency and lower noise sensitivity. IT438 Communication Technology 4 1-6 Summary of Digital Modulation in Optical Fiber Modulation Method Used in Optical Fiber? Comments Simple, widely used in short-distance systems ASK (IM) Yes (e.g., OOK). Common in long-distance/high-speed systems PSK Yes (QPSK, BPSK, DPSK). Highly efficient, used in high-speed coherent QAM Yes systems (e.g., 16-QAM, 64-QAM). Less common, sometimes used in niche FSK Rarely applications (e.g., MSK). IT438 Communication Technology 5 7 Receivers/Filters IT438 Communication Technology 6 1-8 Optical Receivers: Basic Idea Optical receiver is responsible for converting the received optical signal (light) back into an electrical signal. It consists of several components that work together to ensure the signal is properly detected, amplified, and decoded. Two types of optical receivers: 1. Direct detection 2. Coherent detection IT438 Communication Technology 7 1-9 Optical Receivers 1. Direct Detection Receiver It converts the optical signal directly into an electrical signal using a photodetector (e.g., a photodiode). The optical signal is incident on a photodetector which converts the incoming light into a corresponding electrical current (proportional to the intensity of light). The electrical signal is then amplified and processed to recover the transmitted data. Used in low- and medium-speed optical communication systems like fiber-to-the- home (FTTH), data centers, and short-range communication. IT438 Communication Technology 8 1-10 Optical Receivers 1. Direct Detection Receiver (cont.) Advantages: Simple and cost-effective. Suitable for Intensity Modulation/Direct Detection (IM/DD) systems, like On-Off Keying (OOK). Limitations: Limited performance in terms of sensitivity and data rate compared to coherent detection. Exposed to noise and dispersion for long-distance communication. IT438 Communication Technology 9 1-11 Optical Receivers 2. Coherent Detection Receiver It is an advanced type of optical receiver that uses both the amplitude and phase of the optical signal, allowing for more complex modulation formats and higher sensitivity. The optical signal is combined with a local oscillator (a reference laser) using a device called a hybrid, then detected by the photodetector. By comparing the phase and amplitude of the received signal to the local oscillator’s reference, the receiver can detect not just the intensity but also the phase and frequency shifts of the signal. Used in long-haul optical networks, and high-speed optical systems. IT438 Communication Technology 10 1-12 Optical Receivers 2. Coherent Detection Receiver (cont.) Advantages: Higher sensitivity than direct detection. Supports advanced modulation formats like QPSK and QAM, increasing data rates and spectral efficiency. Can compensate for impairments like chromatic dispersion and polarization mode dispersion (PMD) using digital signal processing (DSP). Limitations: More complex and expensive than direct detection. Requires precise synchronization with the local oscillator, making the receiver design more challenging. IT438 Communication Technology 11 1-13 Optical Filters Used to selectively transmit or block certain wavelengths of light while allowing others to pass In multi-channel systems, requires careful control over which wavelengths are allowed to reach the receiver and which should be filtered out. This is where optical filters come in. Key Characteristics of Optical Filters 1. Center Wavelength 2. Bandwidth 3. Rejection Band 4. Insertion Loss 5. Out-of-Band Rejection IT438 Communication Technology 12 1-14 Optical Filters (cont.) Types of Optical Filters: Optical filters are classified based on their function, design, and the type of filtering mechanism they use. 1- Bandpass Filters It allows light of a certain wavelength range (the passband) to pass through, while rejecting or attenuating wavelengths outside this range (the stopband). 2- Fiber Bragg Gratings (FBG) It is a periodic variation in the refractive index of the fiber core, which reflects specific wavelengths and transmits others. 3- Tunable Optical Filters It allows the user to adjust the center wavelength of the passband or stopband, making them highly flexible for dynamic optical systems. IT438 Communication Technology 13 1-15 Optical Filters (cont.) Filter Type Typical WDM Application Advantages High selectivity, commonly Used to isolate and pass specific Bandpass Filters used in wavelength channels multiplexing/demultiplexing Reflects specific wavelengths, Fiber Bragg Gratings Compact, effective for channel used for channel separation and (FBG) filtering and management dispersion compensation ROADMs, dynamic WDM Flexibility to tune different Tunable Optical Filters systems channels, dynamic operation IT438 Communication Technology 14 1-16 Gratings IT438 Communication Technology 15 1-17 Mach-Zehnder Filter/Interferometer (MZI) In a Mach-Zehnder (MZ) interferometer, a splitter splits the incoming wave into two waveguides, and a combiner recombines the signals at the outputs of the waveguides An adjustable delay element controls the optical path length in one of the waveguides, resulting in a phase difference between the two signals when they are recombined. Wavelengths for which the phase difference is 180° are filtered out. By constructing a chain of these elements, a single desired optical wavelength can be selected. IT438 Communication Technology 16 1-18 Mach-Zehnder Filter/Interferometer (MZI) (cont.) Low-cost device Tunability: by varying temperature (~ few ms) [slow and complex] IT438 Communication Technology 17 19 WDM Network Technology IT438 Communication Technology 18 Typical DWDM Network DWDM SYSTEM DWDM SYSTEM VOA EDFA DCM DCM EDFA VOA IT438 Communication Technology 19 DWDM Components 850/1310 15xx Transponder Optical Multiplexer (MUX) Optical De-multiplexer (DEMUX) Optical Add/Drop Multiplexer (OADM)IT438 Communication Technology 20 DWDM Components (Count.) Optical Amplifier Optical Attenuator (EDFA) Variable Optical Attenuator Dispersion Compensator (DCM / DCU) IT438 Communication Technology 21 Fall Semester 2024-2025 IT438 Communication Technology Kamal Hamza, PhD Acknowledgement: This presentation contains some figures and text from Data Communications [email protected] and Networks book by W. Stallings Typical DWDM Network DWDM SYSTEM DWDM SYSTEM VOA EDFA DCM DCM EDFA VOA IT438 Communication Technology 1 3 Transponders IT438 Communication Technology 2 Transponders 850/1310 15xx Transponder Critical component in DWDM systems, typically placed between the Terminal side equipment (e.g., routers or switches) and the DWDM system. Its primary functions are: Signal Conversion: It converts the client signal (which may be electrical or optical but at a non-DWDM wavelength) into a DWDM-compliant optical signal. Regeneration: In some cases, it also regenerates the signal to improve quality for long-distance transmission. Wavelength Assignment: It assigns a specific wavelength to each incoming signal. IT438 Communication Technology 3 Transponders (Cont.) Terminal Side (850/1310/1550 nm) The most common wavelengths on the terminal side are: 850 nm: Used in multimode fiber systems, typically for short-distance connections. 1310 nm: Common for single-mode fiber over medium distances (up to 10 km). 1550 nm: Also used in single-mode fiber, especially for longer-distance connections (10-40 km). DWDM Side (15xx nm) Where the signals are converted into DWDM wavelengths for multiplexing onto a single optical fiber. DWDM systems operate in the C-band (1530-1565 nm) and sometimes in the L-band (1565-1625 nm) of the optical spectrum. These bands are chosen for their low attenuation and efficient transmission over long distances. IT438 Communication Technology 4 Transponders (Cont.) IT438 Communication Technology 5 7 Isolators and Circulators IT438 Communication Technology 6 8 Isolators allows light to pass in one direction but not in the opposite direction. used in the front and end of optical components such as amplifiers and laser diode modules. IT438 Communication Technology 7 9 Circulators Light entering at any particular port exits at the next port IT438 Communication Technology 8 Multiplexers/Demultiplexers IT438 Communication Technology 9 DWDM Components (cont.) Optical Multiplexer (MUX) Optical De-multiplexer (DEMUX) Multiplexer (Mux) Combines multiple optical signals (each with a different wavelength) into a single beam that consists of all these wavelengths. Demultiplexer (Demux) Performs the reverse functionality of multiplexers; Separates the combined optical signals at the receiver end into individual wavelengths, which are sent to the appropriate receiver. IT438 Communication Technology 10 12 DWDM DWDM Mux Demux Wavelength Wavelength Multiplexed Multiplexed Signals Signals Wavelengths Converted via Wavelengths Transponders separated into individual ITU Specific lambdas IT438 Communication Technology 11 13 Circulator with FBG (De)Multiplexer MUX DeMUX IT438 Communication Technology 12 Add/Drop Multiplexers IT438 Communication Technology 13 DWDM Components (cont.) Optical Add-Drop Multiplexer (OADM) Adds or removes specific wavelengths from the DWDM signal while allowing other wavelengths to pass through. This is useful for routing specific channels to different destinations without disrupting the entire signal. Optical Add/Drop Multiplexer (OADM) IT438 Communication Technology 14 16 IT438 Communication Technology 15 17 Four channel OADM IT438 Communication Technology 16 Attenuators IT438 Communication Technology 17 DWDM Components (cont.) Optical Attenuator Variable Optical Attenuator (VOA) Attenuator Used to control the power level of the optical signal in a DWDM system. It adjusts the signal strength to prevent overloading of components (such as optical amplifiers or receivers) and ensures optimal signal quality across different wavelengths. Aid testing of optical system performance under varying optical power conditions. IT438 Communication Technology 18 20 Attenuators Types 1. Fixed Optical Attenuators Provide a predetermined amount of attenuation. Commonly used when a specific level of signal reduction is needed, such as in testing or in long-haul communication links. 2. Variable Optical Attenuators (VOAs) Allow for adjustable attenuation levels. Used in applications where the required level of attenuation may change, such as in dynamic optical networks or during testing. IT438 Communication Technology 19 Fall Semester 2024-2025 IT438 Communication Technology Kamal Hamza, PhD Acknowledgement: This presentation contains some figures and text from Data Communications [email protected] and Networks book by W. Stallings Dispersion Compensation Module (DCM) DCM Dispersion Compensation Module (DCM) Chromatic dispersion (the spreading of different wavelengths of light) causing signal degradation as it travels through the fiber. DCM Dispersion Compensating Fiber (DCF) DCF is engineered with opposite dispersion characteristics, allowing it to reverse this effect when placed in series with regular fibers. In SMF, pulses broaden due to positive chromatic dispersion. As different wavelengths spread out, the pulse loses its sharp shape. DCF has negative chromatic dispersion, meaning that it compresses the pulse back by reversing the broadening. By carefully designing DCF with the right length and dispersion value, it compensates for the dispersion accumulated in the SMF. DCM 80 Km of regular fiber compensating with 15 Km of dispersion compensating fiber. Optical Amplifiers 6 Optical Amplifier 7 Types of Optical Amplifier An optical amplifier is a device that amplifies an optical signal directly without converting it to an electrical signal. 1. Erbium-Doped Fiber Amplifier (EDFA) 2. Raman Amplifier 3. Semiconductor Optical Amplifier (SOA) 8 EDFA - Erbium-Doped Fiber Amplifier EDFA - Erbium-Doped Fiber Amplifier EDFAs use a fiber doped with erbium and a pump laser to increase signal strength. When a weak signal in the C-band or L-band passes through the erbium-doped fiber, it stimulates the excited erbium ions to release additional photons at the same wavelength, amplifying the original signal. High gain with low noise, making them ideal for long-distance communication. Compatible with standard single-mode optical fibers. 9 EDFA - Erbium-Doped Fiber Amplifier Length of fiber: core doped with erbium ions Er3+ Fiber is pumped with a laser at 980 nm or 1480nm. Pump is coupled (in- and out-) using a -selective coupler An isolator is placed at the end to avoid reflections (else this will convert into a laser!) Submarine optical cable EDFA - Erbium-Doped Fiber Amplifier 10 OEO Regenerator 11 Optical Amplifiers vs Regenerators 40-80 km Terminal Terminal Regenerator - 3R (Reamplify, Reshape and Retime) 120 km Terminal Terminal EDFA - 1R (Reamplify) Terminal Terminal Terminal Terminal Terminal Terminal EDFA amplifies all s Optical Amplifiers vs Regenerators Feature Optical Amplifier Regenerator (O-E-O) Converts signal to electrical, cleans it, and Mechanism Amplifies signal directly in optical form reconverts to optical Signal Processing Amplifies signal and noise together Removes noise, reshapes, and retimes signal Signal Quality Degrades with cumulative noise over distance Provides a clean, fully restored signal Application Long-haul, DWDM systems Ultra-long-haul, high-integrity systems Higher cost and complexity due to O-E-O Cost and Complexity Lower, simpler setup conversion Other Technologies 14 15 Wavelength Converters ▪ Wavelength Converters (WC): converts the wavelength of an input signal to another wavelength. 16 WC Technology 1. Opto-Electronic Wavelength Conversion (OE-WC) Incoming optical signal → photodetector converts to electrical signal. Electrical signal is amplified, reshaped, or retimed as needed. Laser tuned to the target wavelength retransmits the processed electrical signal as a new optical signal. 2. Wavelength Conversion Using Coherent Effects Relies on nonlinear optical effects, typically in specialized fibers or semiconductor devices. These effects create new wavelengths by combining or modulating existing wavelengths within the fiber, with no electrical conversion involved. 17 WC Technology Comparison of OE-WC and Coherent Wavelength Conversion Opto-Electronic Wavelength Conversion Wavelength Conversion Using Coherent Feature (OE-WC) Effects Conversion Mechanism Optical to electrical to optical All-optical, nonlinear interactions Signal Regeneration Yes, reshapes and retimes signal No, only transfers wavelength Speed Limited by electronic components Ultrahigh-speed processing Long-haul, metro networks needing Applications DWDM, optical packet-switched networks regeneration Depends on power management and nonlinear Signal Quality High quality due to regeneration effects High (requires photodetectors, lasers, Moderate to high (requires nonlinear media, Implementation Complexity electronics) careful tuning) Typical Devices Used O-E-O transponders, photodetectors, lasers SOAs, highly nonlinear fiber, Kerr-based devices Cost High Lower to moderat 18 Optical Switching ▪ An optical switch takes an input “optical” signal and direct it to the desired output port. ▪ As in conventional switching, optical switching can be either: circuit (wavelength-routed) switching, or packet switching (OPS and OBS). ▪ Currently, WDM optical networks employ circuit switching in the optical domain (instead of routes, we have a route and a wavelength for each connection). ▪ Packet switching is still facing many challenges. Fall Semester 2024-2025 IT438 Communication Technology Kamal Hamza, PhD Acknowledgement: This presentation contains some figures and text from Data Communications [email protected] and Networks book by W. Stallings Network Generations Network Network Transmission Transmission Node Current Status Generation Type Medium Technology Technology G1 Electronic Copper cables Electronic Electronic Still in use: e.g. Network access networks, LANs, and end-user telephone networks G2 Optical Optical fiber Optical (single Electronic Still in use for Network wavelength) small-scale optical systems and LANs. G3 Optical Optical fiber WDM Electronic and Currently deployed Network Hybrid in backbone networks (WANs), MANs, and LANs. G4 (All) Optical Optical fiber WDM Optical In research Network Laboratories All Optical Network All-Optical Networks: Needs and Challenges Fiber/Processor Bottlenecks Fiber Capacity Increase Outstrips Electronic Microprocessors will Dissipate Increasing Power Switching Capacity Increase with Today’s Technology 5 WDM Networks Evolution WDM Network Evolution The three main generations of WDM networks are: 1- Point-to-Point WDM systems, 2- Wavelength Add/Drop Multiplexer (WADM) or Optical Add/Drop Multiplexer (OADM), and 3- Fiber and Wavelength Crossconnects (passive star, passive router, and active switch). 1- Point-to-Point WDM System 1- Point-to-Point WDM System The first generation of WDM networks (early to mid 90’s) was deployed as a cost-effective mean to upgrade the transmission capacity of point-to-point communication links. WDM point-to-point systems have been commercially available for more than a decade. Channel counts of 64 are common. The maximum channel count today is 160, and this number will reach 320 in the near future. 2- Wavelength Add/Drop Multiplexers 2- Wavelength Add/Drop Multiplexers (count.) A WADM consists of a DEMUX, followed by a set of switches , one per wavelength, followed by a MUX. WADM provides a great flexibility to WDM transmission links by allowing the capability of adding/dropping data streams at an intermediate points in the transmission link. 3- Fiber and Wavelength Cross-Connects The introduction of WADM increased the flexibility of the point-to-point WDM system, however, it does not provide the capability of interconnecting a set of multiwavelength fiber links, i.e., a true “WDM networking” infrastructure. The evolution of optical “interconnection” devices in the mid 90’s has marked the birth of the third generation of WDM systems — WDM networks. Based on the interconnection capability, we can distinguish three categorizes of optical interconnection devices: Passive Star Passive Router Active Switch Passive Star The basic functionality of a passive star is as follows: the power of an input signal on a given wavelength from an input fiber port is equally split among all output fibers. Note that, in a passive star, a collision will occur when two or more signals from the input fibers are simultaneously launched into the star on the same wavelength. Passive Router manage the routing of optical signals by utilizing predetermined paths or configurations. They can combine or split signals based on specific wavelengths without any active signal processing or amplification. Passive Router (cont.) Note the following about passive routers: The connectivity pattern between wavelengths on input fibers and the output fibers is fixed and is defined by the “routing matrix” of the router. The routing matrix is determined by the internal connections between the DEMUX and MUX stages inside the router Passive routers are commercially available under several names such as: waveguide grating routers (WGRs), arrayed waveguide grating (AWG), wavelength routers (WRs), and Latin routers. Active Switch Active switches are commonly known as wavelength-routed switch (WRS), wavelength-selective crosseconnect (WSXC). Active Switch The concept of active switches is similar to that of passive routers. However, unlike passive routers in which routing matrix is fixed, active switches have a reconfigurable routing matrix. The principle of operation of an active switch is simple. Similar wavelengths from all input fibers are connected to the same “space” switching fabric. A given switching fabric can be configured to direct the same wavelength from different fibers to any of the output fibers. Active Switch (cont.) Note that, even though active switches provides a routing flexibility for input wavelengths, such switches can be “blocking” under some configurations. For instance, it is impossible to configure the active switch in the figure in the previous slide to connect the input signals on the same wavelength to the same output fiber. This limitation can be overcome by employing wavelength converters. Optical Interconnection Devices Comparison Feature Passive Star Passive Router Active Switch Passive; does not require Passive; relies on optical Operation Active; requires external power power components No signal processing; simply No signal processing; directs Performs signal processing; Signal Processing splits signals signals based on fixed paths dynamically routes signals Fixed configuration; no Fixed paths; limited routing Dynamic configuration; can Configuration dynamic reconfiguration capability reconfigure paths in real time Low; relies on passive Higher due to complexity and Installation Cost Generally low due to simplicity components active components Highly scalable; can manage a Limited scalability; adds fixed Limited scalability; designed for Scalability larger number of connections connections only specific routing needs dynamically Fiber-to-the-home (FTTH), WDM systems, fixed routing Data centers, telecommunications, Applications local area networks applications and metro networks Low; fixed paths limit Low; limited to predetermined High; can adapt to changing traffic Flexibility adaptability paths conditions and reroute dynamically Fall Semester 2024-2025 IT438 Communication Technology Kamal Hamza, PhD Acknowledgement: This presentation contains some figures and text from Data Communications [email protected] and Networks book by W. Stallings Wavelength-Routed Networks Wavelength-Routed Networks Also known as Optical Circuit Switching (OCS) Networks. Similar to the conventional Circuit Switching networks: A circuit (path) is established in a set-up phase between the sender and the receiver Once established, data is streamed on the circuit Stations are connected via a set of nodes. Networks nodes are interconnected with optical fiber cables, each carries a set of wavelengths. In optical networks, the circuit is commonly called a lightpath (wavelength) Wavelength-Routed Networks Wavelength Continuity Constraints Wavelength-Routed Networks wavelength routing and assignment problem (WRA) Given: 1. A set of lightpaths that need to be established (requests) 2. A constraint on the number of wavelengths Compute: 1. The routes over which these lightpaths should be set up, and 2. The wavelengths which should be assigned to these lightpaths so that the maximum number of lightpaths may be established. Wavelength-Routed Networks Solution Strategies: Routing Problem Fixed Routing (shortest path using Dijkstra's) Fixed-Alternate Routing Adaptive Routing Fault-Tolerant Routing Wavelength Assignment Problem Random Wavelength Assignment (R) First-Fit (FF). Least-Used (LU) and Most-Used (MU) Optical Packet Switching (OPS) Networks Speed-Mismatch Problem The increasing mismatch between switching capability and optical transmission capacity has rendered WDM electronic switches into bottlenecks (Size and Speed). Optical Packet Switching Processes packets (e.g., IP packets) in the optical domain without conversion to the electronic domain (no OEO processing in the middle). Design of a truly OPS architecture requires advances in: Optical Memory Optical Computing Recall the amount of processing on IP packets in the Internet (header processing/editing, look-up tables and addressing, etc.) Contention occurs at a switching node whenever two or more packets try to leave the switch fabric on the same output port, on the same wavelength, at the same time. In electrical packet-switched networks, contention is resolved with the store- and-forward technique (using RAM). There is no equivalent optical RAM technology; therefore, the optical packet switches need to adopt different approaches for contention resolution. Contention Resolution: Optical Buffers Control Unit DMUX MUX Passive Splitter Coupler Fiber 1 Fiber 1 FDL λ1 Fiber 2 Fiber 2 FDL FDL = Fiber Delay Line Contention Resolution: Wavelength Converters Control Unit Passive DMUX MUX Coupler Splitter Fiber 1 Fiber 1 WC λ1 Fiber 2 Fiber 2 WC WC = Wavelength Converter Optical Burst Switching (OBS) Networks Motivation for OBS OPS represents a long-term strategy for the network evolution by providing flexible and efficient resource utilization, and finer switching granularity. However, switching at the wavelength granularity is very difficult in optical due to the lack of: an optical equivalent of the random access memory (RAM), and efficient logic devices for optical signal processing. Motivation for OBS (cont.) Optical Burst Switching (OBS) presents a compromise between OCS and OPS, by avoiding some of their key shortcomings. Key idea: direct bursts through an optical network without the need to pass these bursts through electronics whenever a routing decision is necessary. Basic Structure of OBS Assembled from multiple data packets (e.g. IP packets) B. Mukherjee Optical WDM Networks, Springer, 2006. Burst Assembly Burst assembly is the procedure for aggregating data packets from various sources, such as an IP router, into bursts at the edge of an OBS network. Assembly algorithms can be classified as: timer-based, threshold-based, and mixed timer/threshold-based. Burst Assembly: Timer-Based Scheme A timer starts at the beginning of each new assembly cycle. After a fixed time, all the packets that arrived during this period are assembled into a burst. The timeout value should be set carefully. Value is too large, the packet delay at the edge might become intolerable. Value is too small, too many small bursts will be generated, resulting in higher control overhead. Burst Assembly: Threshold-Based A threshold is used as a limiting parameter to determine when to generate a burst. The threshold specifies the number of packets to be aggregated into a burst, or the (minimum or maximum) burst length. Until the threshold condition is met, the incoming packets will be stored in prioritized packet queues in the ingress node. Once the threshold is reached, a burst is created and sent. Burst Assembly: Mixed-Based Scheme Timer-based schemes might result in undesirable burst lengths, threshold-based assembly algorithms do not provide any guarantee on the assembly delay that packets will experience. A mixed timer/threshold-based assembly algorithm may provide better performance, but at the cost of a higher operational complexity. Burst Scheduling A burst-scheduling algorithm at an ingress node is responsible for: 1. Adjusting the offset time for each burst. 2. Scheduling bursts on each output link. 3. Forwarding the bursts and their control packets to the OBS network. Common scheduling techniques: First Fit (FF) and Latest Available Unused Channel (LAUC). Signaling Protocols for OBS A signaling protocol is the procedure through which services are provisioned. In a signaling protocol, service provisioning includes switching-path establishment, deletion and modification. By using a signaling protocol, a control packet can reserve resources for the corresponding data burst by guiding it through a routing path. one-way or two-way Signaling Protocols for OBS (cont.) There are three typical signaling protocols: 1. Just Enough Time (JET), 2. Just In Time (JIT), and 3. Tell and Wait (TAW).