Chapter 11: Introduction to the Nervous System and Nervous Tissue PDF
Document Details
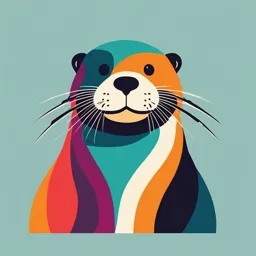
Uploaded by GallantPolonium
Tags
Summary
This document provides an introduction to the nervous system and nervous tissue. It covers the structural organization, functions, and types of neurons in a biological context.
Full Transcript
Chapter 11 Introduction to the Nervous System and Nervous Tissue Structural Organization of the Nervous System CNS PNS Functions of the Nervous System Principal Functions of the Nervous System 1. Sensory - detecting changes in the internal and external...
Chapter 11 Introduction to the Nervous System and Nervous Tissue Structural Organization of the Nervous System CNS PNS Functions of the Nervous System Principal Functions of the Nervous System 1. Sensory - detecting changes in the internal and external environments Vision, hearing, taste, smell, pain Blood pH, blood gases, blood pressure 2. Integrative - analyzing sensory information and deciding how to respond Mental activity (consciousness, thinking, memory, emotion 3. Motor - sending instructions (nerve impulses) to muscles and glands Major body movements Some smooth muscle contraction Secretion from sweat glands, salivary glands, digestive glands -Structural & functional units of the nervous system - PROPERTIES 1. Excitability – ability to respond to stimuli (changes in the body and external environment) 2. Conductivity – produce traveling electrical signals 3. Secretion – when electrical signal reaches end of nerve fiber, a chemical neurotransmitter is secreted Neuron Structures (1) Cell Body (perikaryon or soma) – Membrane is receptive to stimuli from other neurons – Structures characteristic of neurons: Chromatophilic Substance (Nissl bodies) – rough endoplasmic reticulum Neurofibrils – filaments providing support and shape for the cell body (2) Dendrites – Highly branched processes that extend out from the cell body – Specialized for receiving stimuli from the environment, specialized sensory organs, or from other neurons (3) Axon – Single process specialized for conducting nerve impulses to other cells (nerve, muscle, and gland cells Special Structures of the Axon Axon Hillock – cone-shaped region where the axon joins the cell body Initial Segment – the first portion of the axon Trigger Zone – the junction of the axon hillock and the initial segment; where nerve impulses start Axon Collateral – a side branch along the length of an axon Axon Terminals – the branched end portions of an axons Synaptic End Bulb – enlarged region at the end of an axon terminal; contains synaptic vesicles Synaptic Vesicle – a membrane-enclosed sac filled with molecules of neurotransmitter (e.g., acetylcholine, norepinephrine) Types of Neurons Neurons based on structure (the number of processes extending from the cell body) Figure 12–3 A Structural Classification of Neurons. 1. Anaxonic neurons – many dendrites/no axon – help in visual processes 2. Bipolar neurons – Have one dendrite and one axon – Found in the retina of the eye, the internal ear, and the olfactory mucosa 3. Unipolar neurons – Have a single process extending from the cell body – Sensory neurons from skin and organs to spinal cord are this type 4. Multipolar neurons – Have several dendrites and one axon – Most common – Motor neurons and most association neurons are this type Figure 12.3 Types of Neurons (based on function) Sensory (afferent) neurons – detect changes in body and external environment – carry impulses toward the CNS Interneurons (association neurons) – lie between sensory and motor pathways – process, store and retrieve information – confined to the CNS – 90% of our neurons are interneurons Motor (efferent) neurons – Carry instructions from the CNS to muscles and glands – organs that carry out responses called effectors The Synapse Synapse: the point of contact between two neurons 2 Types of Synapses Electrical Chemical Electrical Synapses Found in: – Cardiac muscle – In many types of smooth muscle Chemical Synapses Found in skeletal muscle, gland cells, and between neurons 3 essential components of a chemical synapse: 1. Presynaptic terminal formed from the end of an axon 2. Synaptic cleft – space separating end of axon and cell with which it synapses 3. Postsynaptic membrane of cell opposed to the presynaptic terminal – First neuron releases neurotransmitter onto second neuron that responds to it 1st neuron is presynaptic neuron 2nd neuron is postsynaptic neuron – Synapse may be axodendritic, axosomatic, or axoaxonic Supporting Cells - Cells in the nervous system in addition to neurons - 10-50 times as many neuroglia as neurons - Much smaller than neurons – In the CNS – Neuroglia (or glia): Astrocytes Ependymal cells Microglia Oligodendrocytes – In the PNS Schwann cells Satellite cells Myelinated & Unmyelinated Axons Myelinated Nerve Fibers – Wrapped in many layers of plasma membrane As many as 500 neurolemmocytes may be involved in the myelination of one long axon Each neurolemmocyte forms a sheath consisting up to 100 layers of plasma membrane – Plasma membrane has an unusually high percentage of fat – excellent insulating material – allows the axon to transmit nerve impulses more rapidly Unmyelinated axons rest in the invaginations of oligodendrocytes or Schwann cells White Matter vs. Gray Matter White matter – nervous tissue dominated by myelinated axons – White color due to the presence of myelin sheaths Gray matter – nervous tissue dominated by unmyelinated axons – Cortex - gray matter on the outer surface of much of the brain – Nuclei - collections of gray matter within the brain Electric Signals Electrical Potentials and Currents Nerve pathway is a series of separate cells. Neural communication = mechanisms for producing electrical potentials and currents – Electrical potential – different concentrations of charged particles in different parts of the cell – Electrical current – flow of charged particles from one point to another within the cell Living cells are polarized – Resting membrane potential is -70 mV with a negative charge on the inside of membrane Ionic Basis of Resting Membrane Potential Na+ concentrated outside of cell (ECF) K+ concentrated inside cell (ICF) Resting Membrane Potential Neuron is not being stimulated A typical value is -70 mV Unequal electrolytes distribution between ECF/ICF due to 1. Diffusion of ions down their concentration gradients 2. Selective permeability of plasma membrane 3. Electrical attraction of cations and anions Membrane very permeable to K + – leaks out until electrical gradient created attracts it back in Cytoplasmic anions can not escape due to size or charge (PO43-, SO42-, organic acids, proteins) Membrane much less permeable to Na + Na+/K+ pumps out 3 Na+ for every 2 K+ it brings in – works continuously and requires great deal of ATP Ion Channels The plasma membranes of all cells contain proteins that function as ion channels – Only the plasma membranes of excitable cells (nerve and muscle cells) have ion channels that can respond to certain stimuli by producing impulses (action potentials) – When the ion channels of excitable cells are open, the provide conduction pathways for the flow of ions (just as an electrical wire connected to two terminal of a battery allows the flow of electrons if the switch is “on”) Two Basic Types of Ion Channels 1. Leakage Channels – always open 2. Gated Channels – open or close when activated by specific types of stimuli – Chemically-Gated Activated by chemicals (i.e. neurotransmitters & hormones) Found in neuron cell bodes and dendrites; receptors for taste & smell in the mouth – Mechanically-Gated Activated by mechanical vibration & pressure Found in touch & pressure receptors in the skin – Light-Gated Activated by light Found in rods and cones in the retina of the eye – Voltage-Gated Activated by changes in the voltage (membrane potential) Found highly concentrated at the axon trigger zone Local Potentials Local disturbances in membrane potential – occur when neuron is stimulated by chemicals, light, heat or mechanical disturbance – depolarization decreases potential across cell membrane due to opening of gated Na+ channels Na+ rushes in down concentration and electrical gradients Na+ diffuses for short distance inside membrane producing a change in voltage called a local potential The ion channels remain activated as long as the stimulus persists. Vary in magnitude and duration The greater the stimulus the more gated ion channels open or close, and the greater the change in the membrane potential Chemical Excitation Action Potentials More dramatic change in membrane produced where high density of voltage-gated channels occur Triggered when a graded potential reaches a certain critical level (or threshold) and activate voltage-gated ion channels A segment of membrane depolarizes (-70mV to 0 mV), reverses polarization (0 to +30 mV), and then repolarizes (+30 to – 70 mV) Four characteristics distinguish local potentials from action potentials 1. Local potentials are graded; they vary in magnitude depending on strength of stimulus. 2. Local potentials are decremental; they get weaker as they spread from the point of stimulation. 3. Local potentials are reversible if stimulation ceases. 4. Local potentials can be either excitatory or inhibitory; the result of inhibitory local potentials is hyperpolarization (increase in negative potential). Action Potential 1. When Na+ ions arrive at the axon hillock, they depolarize the membrane at that point. This appears as a steadily rising local potential. 2. For anything more to happen, the local potential must rise to a critical voltage called the threshold (~-55 mV). This is the minimum needed to open the voltage-regulated gates. 3. The neuron now “fires,” or produces an action potential. At threshold, voltage-regulated Na+ gates open quickly, while K+ gates open more slowly. As Na+ enters the cell, it further depolarizes the membrane, which stimulates still more voltage- regulated Na+ gates to open and admit more Na+. A positive feedback cycle is created that makes the membrane voltage rise quickly. 4. As the rising membrane potential passes 0 mV, Na+ gates are inactivated and begin closing. By the time they all close and the Na+ inflow ceases, the voltage peaks at ~+35 mV. The membrane is now positive on the inside and negative on the outside – its polarity is reversed. 5. By the time the voltage peaks, the slow K+ gates are fully open. K+ exits the cell and their outflow repolarizes the membrane and shifts the voltage back into negative numbers. 6. Potassium gates stay open longer than Na+ gates, so the amount of K+ that leaves the cell is greater than the amount of Na+ that entered. Therefore, the membrane voltage drops to 1 or 2 mV more negative than the original RPM, producing a negative overshoot called hyperpolarization. 7. Na+ and K+ switch places across the membrane during an action potential. During hyperpolarization, the original RPM is gradually restored. Action Potentials Action Potentials Sometimes called spikes Characteristics of action potentials – follow an all-or-none law voltage gates either open or don’t – nondecremental (do not get weaker with distance) – irreversible (once started goes to completion and can not be stopped) The Refractory Period Period of resistance to stimulation Absolute refractory period – as long as Na+ gates are open – no stimulus will trigger AP Relative refractory period – as long as K+ gates are open – only especially strong stimulus will trigger new AP Refractory period is occurring only to a small patch of membrane at one time (quickly recovers) Impulse Conduction - Unmyelinated Fibers Impulse Conduction in Unmyelinated Fibers Threshold voltage in trigger zone begins impulse Nerve signal (impulse) - a chain reaction of sequential opening of voltage-gated Na+ channels down entire length of axon Nerve signal (nondecremental) travels at 2m/sec Signal Conduction in Myelinated Fibers Saltatory Conduction – Propagation of an action potential along the exposed portions of the fiber – Action potentials seem to jump from one node of Ranvier to the next Voltage-gated channels needed for action potentials – fewer than 25 per m2 in myelin-covered regions – up to 12,000 per m2 in nodes of Ranvier Fast Na+ diffusion occurs between nodes Postsynaptic Potentials Excitatory postsynaptic potentials (EPSP) – a positive voltage change causing postsynaptic cell to be more likely to fire result from Na+ flowing into the cell – glutamate and aspartate are excitatory neurotransmitters – ACh and NE may excite or inhibit depending on cell Inhibitory postsynaptic potentials (IPSP) – a negative voltage change causing postsynaptic cell to be less likely to fire (hyperpolarize) result of Cl- flowing into the cell or K+ leaving the cell – glycine and GABA are inhibitory neurotransmitters – ACh and NE may excite or inhibit depending upon cell Neural Integration The more synapses a neuron has the greater its information-processing capability – cells in cerebral cortex with 40,000 synapses – cerebral cortex estimated to contain 100 trillion synapses Chemical synapses are decision-making components of the nervous system – ability to process, store and recall information is due to neural integration Based on types of postsynaptic potentials produced by neurotransmitters Summation of Postsynaptic Potentials Net postsynaptic potentials in trigger zone – firing depends on net input of other cells typical EPSP voltage = 0.5 mV and lasts 20 msec 30 EPSPs needed to reach threshold – Temporal summation single synapse receives many EPSPs in short time – Spatial summation single synapse receives many EPSPs from many cells Neurotransmitters: Chemical Classification (1) Amino Acids Inhibitory: GABA, Glycine Excitatory: Aspartate, Glutamate (2) Biogenic Amines (produced in axon terminals Serotonin Histamine Catecholamines: Norepinephine, Epinephrine, Dopamine (3) Neuropeptides (produced in neuron cell bodies) Endorphins (4) Acetylcholine ( produced in axon terminals) Synaptic Transmission 3 kinds of synapses with different modes of action 1. Excitatory cholinergic synapse = Ach – Nerve signal opens voltage-gated calcium channels in synaptic knob – Triggers release of ACh which crosses synapse – ACh receptors trigger opening of Na+ channels producing local potential (postsynaptic potential) – When reaches -55mV, triggers AP in postsynaptic neuron 2. Inhibitory GABA-ergic synapse = GABA – Nerve signal triggers release of GABA which crosses synapse – GABA receptors trigger opening of Cl- channels producing hyperpolarization – Postsynaptic neuron now less likely to reach threshold 3. Excitatory adrenergic synapse = NE – NE acts through 2nd messenger systems (cAMP) receptor is an integral membrane protein associated with a G protein, which activates adenylate cyclase, which converts ATP to cAMP cAMP has multiple effects – binds to ion gate inside of membrane (depolarizing) – activates cytoplasmic enzymes – induces genetic transcription and production of new enzymes Cessation and Modification of Signal Mechanisms to turn off stimulation 1. diffusion of neurotransmitter away into ECF astrocytes return it to neurons 2. synaptic knob reabsorbs amino acids and monoamines by endocytosis 3. acetylcholinesterase degrades ACh choline reabsorbed and recycled Neuromodulators modify transmission – raise or lower number of receptors – alter neurotransmitter release, synthesis or breakdown Removal of Neurotransmitter Excitatory Excitatory Cholinergic Adrenergic Synapse Synapse