Fluid Compartments PDF - Introduction to Medical Sciences 1
Document Details
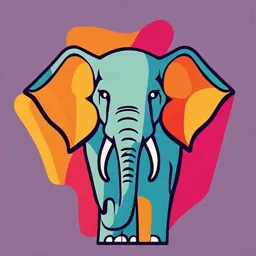
Uploaded by ArdentFourier8221
Brunel University London
2024
Mike Ferenczi
Tags
Summary
This document is a lecture on fluid compartments in medical sciences. It explains the significance of water, solutes, and homeostasis. Key concepts like diffusion, osmosis, and permeability are also explored.
Full Transcript
Introduction to Medical Sciences 1 Cell Signalling and Movement Fluid Compartments Copyright © Brunel University London v.3 2024. All rights reserved. Cell Signalling and Movement – Fluid Compartments Professor Mike Ferenczi Version 3 2024 Michael.Ferenczi@Br...
Introduction to Medical Sciences 1 Cell Signalling and Movement Fluid Compartments Copyright © Brunel University London v.3 2024. All rights reserved. Cell Signalling and Movement – Fluid Compartments Professor Mike Ferenczi Version 3 2024 [email protected] Copyright © Brunel University London V.3 2024. All rights reserved. Fluid Compartments The adult body consists of approximately 60% water The water is found in a number of separate compartments which we shall examine here There are important differences in the solutes dissolved in the water of each compartment Thus each compartment is quite distinctive, and the solutes are functionally important Homeostasis mechanisms maintain the solute distribution in the compartments Copyright © Brunel University London V.3 2024. All rights reserved. Fluid Compartments A number of important concepts need to be understood, such as: Membranes Permeability Diffusion and Brownian motion Osmosis Osmolarity and Osmolality Tonicity Transport and exchange across membranes Exchange across capillary walls pH Electrical charge Electrolytes Copyright © Brunel University London V.3 2024. All rights reserved. Biological membranes: Usually lipid bilayer Phosphatidylcholine: a glycerophospholipid. Glycolipid. Sterol. The lipid bilayer is about 5nm thick (5x10-9m) Signalling proteins traverse through thickness of the bilayer Watson, 2015 https://www.ncbi.nlm.nih.gov/pmc/articles/PMC4626904/ Copyright © Brunel University London V.3 2024. All rights reserved. Permeability Lipid membranes are semi-permeable Some types of molecules can diffuse easily across the membrane, e.g. travel from the intracellular space to the extracellular space, or vice versa. Lipid membranes are hydrophobic: water and charged or polar molecules do not diffuse freely through the membrane Fat-soluble molecules can diffuse through biological membranes, but their solubility in plasma, extracellular fluid or interstitial fluid is limited Large, charged molecules are trapped, in or out All molecules do cross the membranes – it is all a matter of timescales. e.g. water Copyright © Brunel University London V.3 2024. All rights reserved. Permeability (for information only) in units of cm/s Gases travel very fast through membranes Ethanol travels faster than water Water diffuses 100 times slower than CO2, but is present at a huge concentration (55Mol/L) Na+ and K+ have extremely low permeability coefficients Yang and Hinner, 2015 https://www.ncbi.nlm.nih.gov/pmc/articles/PMC4891184/ Copyright © Brunel University London V.3 2024. All rights reserved. Brownian motion and diffusion Molecules dissolved in a solvent move in a random 3-dimensional fashion driven by Brownian motion The random forces generated by the thermally excited water molecules can account for the motion The spontaneous random movement will lead to equilibration: all solvent volumes will end up with the same concentration of solutes This is a simulation of the Brownian motion of a big particle (dust particle) that collides with a large set of smaller particles (molecules of a gas) which move with different velocities in different random directions. https://commons.wikimedia.org/wiki/File:Brownian_moti on_large.gif#/media/File:Brownian_motion_large.gif Copyright © Brunel University London V.3 2024. All rights reserved. Brownian motion and diffusion The spontaneous random movement will lead to equilibration: all solvent volumes will end up with the same concentration of solutes Solutes will diffuse to reach the diffusion equilibrium Helmenstine, 2022 https://sciencenotes.org/osmosis-vs-diffusion-definition-and-examples/ Copyright © Brunel University London V.3 2024. All rights reserved. Osmosis Spontaneous movement or diffusion of water (or other solvent) molecules through a selectively permeable membrane The movement is spontaneous, driven by Brownian motion The movement is directional: to equilibrate the concentration of solvent and solute molecules on both sides of the membrane No energy is required. Process is driven by the concentration gradient and the kinetic energy of the molecules When pure water is added to blood, plasma becomes dilute (hypotonic) compared to the inside of the red blood cells, and water will flow into the red blood cell to reduce the difference in tonicity, causing the red blood cells to swell, and possibly even burst Copyright © Brunel University London V.3 2024. All rights reserved. Osmolarity and osmolality Osmolality is the concentration of all solutes in a liquid such as plasma, measured as osmoles (Osm) per Kg of solvent (usually water) whereas Osmolarity is: the concentration measured as osmoles (Osm) per L of solvent. In biological systems, the difference is not important. Osmolarity is affected by temperature and pressure, but not osmolality. Slightly different because of weight of solutes, but below concentration of 0.5M/L, values are very similar Calculations are based on [Na+], the major solute of extracellular liquid, blood glucose and BUN (Blood Urea Nitrogen). Clinical osmolarity = 2x[Na+] + [Glucose]/18 + [BUN]/2.8 18 and 2.8 because Glucose and BUN are measured in mg/dL and need conversion to mmol/L Copyright © Brunel University London V.3 2024. All rights reserved. Tonicity Tonicity is similar to osmolarity, but osmolarity is the property of a solution containing solutes, whereas tonicity is the osmotic property of this solution compared to the osmotic property of another solution. Dilute plasma may be hypotonic compared to the intracellular content of red blood cells if it has fewer solute molecules than in the intracellular space. The solvent, water will diffuse into the red blood cells to make the tonicity equal on both sides of the membrane. That will cause the red blood cells to swell, and possibly burst. Hypertonic plasma will make the red blood cells shrink Copyright © Brunel University London V.3 2024. All rights reserved. Fluid Compartments The extracellular compartments plasma, lymph and interstitial fluids have similar composition, but plasma has more protein than interstitial fluid Intracellular fluid is quite different because of low sodium Solute In plasma (mMol/L) In muscle cells (mMol/L) Na+ 140 10 K+ 5 150 Ca2+ 2 10-4 Cl- 105 5 Organic phosphates1- 10 100 Proteins17- 1 2 pH 7.4 7.1 Osmolarity 263 mOsmol/L 267 mOsmol/L Copyright © Brunel University London V.3 2024. All rights reserved. Fluid Compartments Proteins carry multiple charges which are on balance negative, at normal pH Solute In plasma (mMol/L) In muscle cells (mMol/L) Na+ 140 10 K+ 5 150 Ca2+ 2 10-4 Cl- 105 5 Organic phosphates1- 10 100 Proteins17- 1 2 pH 7.4 7.1 Osmolarity 263 mOsmol/L 267 mOsmol/L Copyright © Brunel University London V.3 2024. All rights reserved. Fluid Compartments but it is not the charge that contributes to osmolarity. It is the number of particles Solute In plasma (mMol/L) In muscle cells (mMol/L) Na+ 140 10 K+ 5 150 Ca2+ 2 10-4 Cl- 105 5 Organic phosphates1- 10 100 Low concentration Proteins17- 1 2 But highly charged pH 7.4 7.1 anions Osmolarity 263 mOsmol/L 267 mOsmol/L Copyright © Brunel University London V.3 2024. All rights reserved. Fluid Compartments Electric charges determine the electrical gradient or electrical field across the cell membranes. Charged solutes are called electrolytes. They allow water to conduct electricity Solute In plasma (mMol/L) In muscle cells (mMol/L) Na+ 140 10 K+ 5 150 Ca2+ 2 10-4 Cl- 105 5 Organic phosphates1- 10 100 Proteins17- 1 2 pH 7.4 7.1 [H+] 3.98x10-5 mMol/L 7.94x10-5 mMol/L Copyright © Brunel University London V.3 2024. All rights reserved. Factors affecting cell volume Tonicity controls cell volume Concentration of each species of solute determines osmolarity and concentration gradients move solutes and water across semi-permeable membranes Both cations (e.g. Na+) and anions (e.g. Cl-) contribute to osmolarity as they are each species of solutes Concentration of unbalanced electric charges determines the electric field and membrane potential pH controls ionisation state of molecules Changes in cell volume depend on osmolarity in and out of cell Tonicity also depends on whether the membrane is impermeable to some solutes. The permeability consideration may depend on the time-scale Copyright © Brunel University London V.3 2024. All rights reserved. Permeability of real cells and cell volume control Water moves through cell membranes, slowly Proteins and phosphates are impermeant and are at higher concentration inside cells than out. Water tends to move into the cells and make them swell to dilute the impermeant anions Cells don’t burst because the Na+/K+ pump maintains Na+ lower inside the cells than outside (pump makes membrane effectively impermeant to Na+; so net movement of Na+, accompanied by water), so Na+ is effectively an impermeant external solute which balances internal impermeant solutes. Red blood cells don’t have mitochondria, so do not produce ATP to drive the Na+/K+ pump. So they burst when placed in hypotonic solution Copyright © Brunel University London V.3 2024. All rights reserved. Membrane potential The membrane potential is a measure of the imbalance of electric charge across a cell membrane. This is the voltage recorded between the intracellular space and the extracellular space, where the reference electrode is placed, shows the membrane potential The membrane potential is usually -60 to -90 mV, with the inside negative compared to the outside There is imbalance of ions: K+ is high inside Khan Academy, 2018 the cell and Na+ is high outside the cell https://www.khanacademy.org/science/biology/hu man-biology/neuron-nervous-system/a/the- membrane-potential Copyright © Brunel University London V.3 2024. All rights reserved. Membrane potential The membrane potential is a measure of the imbalance of electric charge across a cell membrane. This is caused by the negative charge of the non- diffusible intracellular proteins and phosphates, and the non-equilibrium ionic distribution of Na+ and K+ maintained by active pumps, in particular the Na-K pump. Outside: [Na+] = 150 mM/L; [K+] = 5 mM/L; [Cl-] = 110 mM/L Inside: [Na+] = 10 mM/L; [K+] = 150 mM/L; [Cl-] = 5 mM/L The Na-K pump is responsible for maintaining the charge imbalance The Na-K pump is electrogenic: it creates a difference in electric charge by pumping 3 positive charges out (Na+) in exchange for only two positive charges in (K+) The pump requires energy, available by hydrolysis of ATP to ADP Copyright © Brunel University London V.3 2024. All rights reserved. Membrane potential The Nernst equation can be used to calculate the membrane potential, depending on the charge imbalance. Em is the potential difference of an ion between membranes R is the universal gas constant; R = 8.314 J mol-1 T is the thermodynamics temperature, in Kelvin; 0 K = -273.15oC and 37oC corresponds to 310.15 K z is the number of moles of electrons transferred between Pivovarov, Calahorro and Walker (2018) membranes (defined by the valency of ion; z=1 for Na+) https://pubmed.ncbi.nlm.nih.gov/30488358/ F is the Faraday's constant; F = 96,485 C mol-1 [Na+]o is the concentration of ion outside the membrane [Na+]i is the concentration of ion inside the membrane 2.303 is the factor to convert ln to log10. RT/zF = 0.0267 at 37oC [Na+]o = 0.15 M [Na+]i = 0.01 M Em = 0.0723 V = 72.3 mV The equation can be modified to include all permeable ions Copyright © Brunel University London V.3 2024. All rights reserved. Membrane potential The Nernst equation can be used to calculate the membrane potential, depending on the charge imbalance. The Nernst equation describes how a difference in electrolyte concentration on both sides of a semi-permeable membrane results in a membrane potential. For biological cell Pivovarov, Calahorro and Walker (2018) https://pubmed.ncbi.nlm.nih.gov/30488358/ membranes, the equation shows that the membrane potential is proportional to the logarithm of the ratio of the impermeant cation concentrations inside and outside the cell. Copyright © Brunel University London V.3 2024. All rights reserved. Fluid Compartments Considering a 70Kg man The largest compartment is intracellular fluid, that is water contained within cells, confined by the cell membranes. 55% of body water (23L for 70Kg man containing 60% water) Water which is not intracellular is extracellular. The extracellular compartment can be broken down into several spaces: Interstitial fluid: water in-between cells, including lymph; 36% or 15L Blood plasma: 7% or 3L of body water Transcellular fluid: specialised fluid compartments: cerebrospinal fluid (spinal cord, choroid plexus and brain ventricles), aqueous and vitreous humour in the eyeball, synovial fluid in joints (2% or 1L body fluid), saliva, gastric juice, urine Total fluid: 23 + 15 + 3 + 1 = 42L; 42/70 = 60% of total body weight Copyright © Brunel University London V.3 2024. All rights reserved. Fluid Compartments Men vs women Women body water is slightly less than in men because of the higher adipose tissue content in women (~55% vs 60% water) Age Water content of the body decreases with age. This particularly noticeable in the skin, hence the moisturiser industry. Older people have a tendency to drink less, with adverse effects. Clinically, this is called dehydration Dehydration can de measured from the osmolality of blood, or from the specific gravity or osmolality of urine Definition: hypertonicity of serum (Na+ > 145 mmol/L Sport medicine: urine osmolality > 700 mOsmol/kg H2O or urine specific gravity >1.02, but urine measurements are less reliable as they depend on timing of exercise, drinking etc Copyright © Brunel University London V.3 2024. All rights reserved. Dehydration Chronic or repeated dehydration leads to Urinary tract infections Lung infections Kidney stones Kidney failure Seizures Particularly dangerous for babies, children and older adults Caused by: Diarrhoea and vomiting (infections, fevers) Intensive exercise in hot weather, excessive sweating Medication Diabetes Not drinking enough Copyright © Brunel University London V.3 2024. All rights reserved. Movement of water and molecules across the capillary wall Dee Unglaub et al, 2009 Thoracic Key, 2016 https://thoracickey.com/capillary-function-and- the-lymphatic-system/ Copyright © Brunel University London V.3 2024. All rights reserved. Movement of water and molecules across the capillary wall Oncotic pressure, also called colloid osmotic Plasma proteins generally pressure is the pressure exerted by large molecules cannot cross the endothelial that do not cross the capillary wall easily. interstitial fluid cell membranes & cannot get through the pores between Understanding oncotic Water-filled pore cells pressure is key to membrane understand why water endothelial cell plasma gets back into the blood stream. lipid-soluble substances cytoplasm pass through the endothelial cells Exchangeable proteins are moved across by Small water-soluble vesicular transport substances pass through the pores between cells https://quizlet.com/139463981/physio-1-cell-membrane-and-transport-flash-cards/ Copyright © Brunel University London V.3 2024. All rights reserved. Movement of water and molecules across the capillary wall The diagram represents the connection between arterioles and venules through the capillary bed. The labels are as follows: Pc = hydrostatic pressure in the arterial or venous vessels From: https://en.wikipedia.org/wiki/File:StarlingEquation.svg Pi = hydrostatic pressure in the interstitial space Πc = oncotic pressure in the arterial or venous vessels. Oncotic is the osmotic pressure caused by the proteins which do not flow freely through the blood vessel walls – mainly albumin). Πi = oncotic pressure in the interstitial space Pressure in the diagram is in mm of Hg. Copyright © Brunel University London V.3 2024. All rights reserved. Movement of water and molecules across the capillary wall Hydrostatic pressure and oncotic pressures change across the capillary bed, from the arterial to the venous sides. On the arterial side, hydrostatic pressure pushes From: https://en.wikipedia.org/wiki/File:StarlingEquation.svg water out of the capillaries. On the venous side, the hydrostatic pressure is lower, but maintained oncotic pressure brings water back into the blood stream Copyright © Brunel University London V.3 2024. All rights reserved. Oedema Swelling of a tissue because of excess interstitial fluid caused by: imbalance of forces causing fluid to move between the blood plasma, interstitium and lymphatic vessels which return excess interstitial fluid to the circulation. NHS, 2022 https://www.nhs.uk/conditions/oedema/ Usually a consequence of increased capillary wall permeability to plasma proteins The forces include hydrostatic pressure from the cardiac cycle Osmotic pressure due to proteins, mainly albumin (colloid osmotic pressure) Copyright © Brunel University London V.3 2024. All rights reserved. Thank you Copyright © Brunel University London V.3 2024. All rights reserved. Please feel free to contact me using the following address: [email protected] Copyright © Brunel University London V.3 2024. All rights reserved.