2 -.docx
Document Details
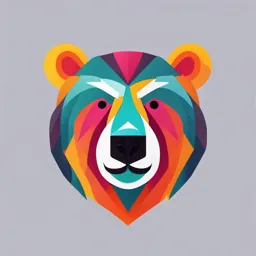
Uploaded by SleekDramaticIrony
Full Transcript
Physical principles of heat Van der Waals Force: weak intermolecular forces dependent on distance between atoms and molecules Heat: energy transfer from higher to lower temperature Measured in Joules or cal 1 cal = 4.19 J Temperature: tendency of object to gain or lose heat Latent heat: energy neede...
Physical principles of heat Van der Waals Force: weak intermolecular forces dependent on distance between atoms and molecules Heat: energy transfer from higher to lower temperature Measured in Joules or cal 1 cal = 4.19 J Temperature: tendency of object to gain or lose heat Latent heat: energy needed to increase the kinetic energy of the molecules to overcome the forces of attraction between them Heat capacity: units of heat per change in temperature Specific heat capacity: amount of heat required to raise the temperature of 1 kg of substance 1 K Heat transfer Conduction: heat energy diffuses from molecule to molecule Convection: currents develop as the result of the rising of warmed gas thereby effecting a loss of het from a system Radiation: rate at which heat energy is radiated? Evaporation Heat of vaporization: number of J required to vaporize a mol/volume of substance without changing its temperature Heat per gram of liquid required to vaporize liquid Vapor pressure: pressure exerted by vapor in thermodynamic equilibrium with its condensed phases at a given temperature in a closed system Condensed state: state at which molecules are closest together A condensed phase in gas is a gas phase that is either condensed to a liquid or is present in negligible amounts. The condensed phase rule states that any gas phase can be condensed to a liquid or is present in negligible amounts. When a gas is cooled, its particles will eventually stop moving about so fast and form a liquid. This is called condensation and occurs at the same temperature as boiling. In theory, all gases can be condensed no matter how low or high the boiling point of the chemical species. This is because by continuously lowering or elevating the temperature (or by altering the pressure), the gas can recover the disrupted intermolecular forces of attraction resulting in the conversion to liquid. Increasing the pressure on a gas may change the state to a liquid. Increasing the pressure on a liquid may change the state to a solid. Saturated vapor pressure: point at which a liquid condenses at a particular pressure Used to build accurate vaporizers Fluids and flow Laminar flow: straightforward flow with greatest flow in the middle Re <2000 flow is laminar Viscosity (resistance to flow) is an important parameter to determine laminar flow Flow is directly proportional to hydrostatic pressure gradient Flow is inversely proportional to fluid viscosity and length of the tube Hagen Poiseuille Equation Turbulent flow: fluid flows unpredictably The dimensionless Reynolds number predicts whether the fluid flow would be laminar or turbulent referring to several properties such as velocity, length, viscosity, and also type of flow. It is expressed as the ratio of inertial forces to viscous forces Re = Velocity x pressure x density / viscosity Re >2000 in turbulent flow Density (measure of spaces between two particles in a given fluids) is an important parameter to determine turbulent flow Characteristics to produce turbulent flow = high flow rates, sharp angles, changes in diameter, branches Density-viscosity e.g. Oil is less dense than water (it floats on top of water) but more viscous than water Tube: length>diameter Orifice: diameter> length Bernouli effect The Bernoulli principle states that when an ideal fluid flows through a constriction, the flow velocity increases and the fluid pressure decreases. In a Venturi mask, oxygen flows through a constriction to create a pressure drop, which entrain ambient air and mixes it with the oxygen flow. The size of the constriction determines the final oxygen concentration for a given gas flow Lower pressure caused by Bernouli effect entrains air to mix with oxygen in Venturi masks Coanada Effect: how a fluid or gas will attach to a surface after passing through an asymmetrical narrowing, exerting force on neighboring fluid or gas The Coanda effect is caused by a similar principle to the Venturi effect. The flowing fluid has a high velocity and a low pressure so entrains surrounding gas into it. On the chamber wall side, this "attempted" entrainment lowers the pressure and "sucks" the fluid against the wall. Clinically, this may give rise to unequal ventilation due to endobronchial mucus. It can also promote coronary ischemia due to atheromatous coronary arterial constrictions. If a constriction occurs at bifurcation because of increase in velocity and reduction in the pressure, fluid (air, blood tends to stick to one side of the branch causing maldistribution LaPlace’s Law Tension: internal force generated by a structure Pressure gradient tube = tension/radius ; pressure gradient sphere = 2 x tension / radius Gas volume measurement Benedict roth spirometer, vitalograph, wright’s spirometer, electronic volume monitor Volume and flow measurement Thorpe tube, wright peak flowmeter, pneumotachograph, Pitot tubes, electronic mass flowmeter Fick Principle: calculation of pulmonary blood flow from oxygen uptake The total uptake of oxygen is equal to the product of the cardiac output and the arterial-venous oxygen content difference Rate of flow to organ = rate of uptake or excretion of substance / difference in conc. of substance in arterial and venous blood Gas Laws STP = standard temperature and pressure 0 degrees C and 760 mmHg/1 atm Always use K when working with gases At OK, motion stops for all substances Boyle’s Law = at constant temperature, pressure is inversely related to volume – less volume = more pressure Charles’ Law = at constant pressure, volume is directly proportional to its absolute temperature - if the pressure remains constant = increased temperature = increased volume Gay-Lussac’s Law = at constant volume, the pressure of an ideal gas is directly proportional to absolute temperature – increased temperature = increased pressure Combination of Boyle’s, Charles’, and Gay-Lussac = Universal Gas Law Avogadro’s Hypothesis: if pressure and temperature constant, then same volumes of gas have same number of molecules Avogadro’s Law: if pressure and temperature constant, then direct relationship between volume and number of moles for an ideal gas At STP, 1 mole of gas occupies 22.4L = molar volume of gas Dalton’s Law: Ptotal = P1+P2+P3 – partial pressures of gas equal the sum of the whole Amagat’s Law: Vtotal = V1+V2+V3 – partial volumes of gas equal the sum of the whole Graham’s Law: diffusion of gas is inversely proportional to the square root of its molecular weight Lighter gases diffuse quickly Heavier gases diffuse slowly A-a gradient = alveolar-arterial gradient: difference between oxygen concentration in the alveoli and arteries A-a Gradient = PAO2 – PaO2 PAO2 = alveolar oxygen pressure, PaO2 = arterial oxygen pressure Normally close to zero (but not zero due to physiological shunt of small amount of blood that bypasses alveoli) Increased gradient in Diffusion defects e.g. pulmonary edema V/Q defects Right-left shunts Won’t see increased gradient in hypoventilation or high altitude. Why? Patients can exhibit hypoventilation for various reasons; some include CNS depression, neuromuscular diseases such as myasthenia gravis, poor chest elasticity, as seen in kyphoscoliosis, or patients with vertebral fractures, and many others. Patients with poor ventilation lack oxygen tension throughout their arterial system in addition to the respiratory system. Thus, the river will have decreased flow throughout both parts. Since both the "A" and the "a" decrease in concert, the gradient between the two will remain within normal limits (even though both values will decrease). Thus patients with hypoxemia due to hypoventilation will have an A-a gradient within normal limits. https://www.ncbi.nlm.nih.gov/books/NBK545153/