Basics of Nuclear Physics PDF
Document Details
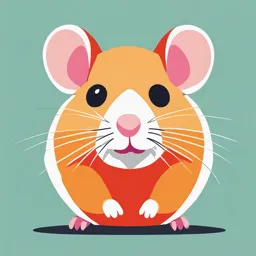
Uploaded by ExtraordinaryPlot8049
2024
Tags
Summary
These lecture notes cover the basics of nuclear physics, including topics on atomic structure and related concepts. The document includes historical context, key principles, and formulas related to nuclear physics to cover fundamental aspects of the subject.
Full Transcript
Basics of Nuclear Physics Ref: “Physics for Scientists and Engineers with Modern Physics” by Raymond A. Serway and John W. Jewett, Jr., 10th edition, 2019 Chapter 41 – Atomic Physics Chapter 43 – Nuclear Physics BME 229 1 Fall 2024...
Basics of Nuclear Physics Ref: “Physics for Scientists and Engineers with Modern Physics” by Raymond A. Serway and John W. Jewett, Jr., 10th edition, 2019 Chapter 41 – Atomic Physics Chapter 43 – Nuclear Physics BME 229 1 Fall 2024 Joseph John Thomson 1856 – 1940 English physicist Received Nobel Prize in 1906 Usually considered the discoverer of the electron Worked with the deflection of cathode rays in an electric field ▪ Opened up the field of subatomic particles 2 Early Models of the Atom, Thomson’s J. J. Thomson established the charge to mass ratio for electrons. His model of the atom ▪ A volume of positive charge ▪ Electrons embedded throughout the volume ▪ The atom as a whole would be electrically neutral. 3 Early Models of the Atom, Rutherford’s Rutherford ▪ Planetary model ▪ Based on results of thin foil experiments ▪ Positive charge is concentrated in the center of the atom, called the nucleus ▪ Electrons orbit the nucleus like planets orbit the sun 4 Niels Bohr 1885 – 1962 Danish physicist An active participant in the early development of quantum mechanics Headed the Institute for Advanced Studies in Copenhagen Awarded the 1922 Nobel Prize in physics ▪ For structure of atoms and the radiation emanating from them 5 Bohr’s Model of Atom, 1 The electron moves in circular orbits around the proton under the electric force of attraction. ▪ The Coulomb force produces the centripetal acceleration. 6 7 Bohr’s Model of Atom, 2 Only certain electron orbits are stable. ▪ Bohr called these stationary states. ▪ These are the orbits in which the atom does not emit energy in the form of electromagnetic radiation, even though it is accelerating. ▪ Therefore, the energy of the atom remains constant and classical mechanics can be used to describe the electron’s motion. ▪ This representation claims the centripetally accelerated electron does not continuously emit energy and therefore does not eventually spiral into the nucleus. 8 Bohr’s Model of Atom, 3 Radiation is emitted by the atom when the electron makes a transition from a more energetic initial stationary state to a lower-energy stationary state. ▪ The transition cannot be treated classically. ▪ The frequency emitted in the transition is related to the change in the atom’s energy. ▪ The frequency is independent of frequency of the electron’s orbital motion. ▪ The frequency of the emitted radiation is given by ▪ E = Ei – Ef = hƒ ▪ h is the Planck’s constant (h = 6.6261×10-34 m2.kg/s) ▪ If a photon is absorbed, the electron moves to a higher energy level. The full understanding of the atomic structure requires theories in Quantum Mechanics. 9 Milestones in the Development of Nuclear Physics 1896: The birth of nuclear physics ▪ Becquerel discovered radioactivity in uranium compounds Rutherford showed the radiation had three main types: ▪ alpha (He nuclei) ▪ beta (electrons) ▪ gamma (high-energy photons) 1911: Rutherford, Geiger and Marsden performed scattering experiments ▪ Established that the nucleus could be treated as a point mass and a point charge ▪ Most of the atomic mass was contained in the nucleus ▪ Nuclear force was a new type of force 10 Some Properties of Nuclei All nuclei are composed of protons and neutrons. ▪ Exception is ordinary hydrogen with a single proton The atomic number Z equals the number of protons in the nucleus. ▪ Sometimes called the charge number The neutron number N is the number of neutrons in the nucleus. The mass number A is the number of nucleons in the nucleus. ▪ A=Z+N ▪ Nucleon is a generic term used to refer to either a proton or a neutron ▪ The mass number is not the same as the mass of the nucleus. 11 12 13 Charge The proton has a single positive charge, +e. The electron has a single negative charge, - e. ▪ e 1.6 x 10-19 C The neutron has no charge. ▪ Made it difficult to detect neutrons in early experiments ▪ Easy to detect with modern devices 14 Mass It is convenient to use atomic mass units, u (or amu), to express masses. ▪ 1 u = 1.660539 x 10-27 kg ▪ Based on definition that the mass of one atom of 12C is exactly 12 u ▪ Mass of one atom of hydrogen 1 u Mass can also be expressed in MeV/c2. ▪ From ER = mc2 ▪ 1 u = 931.494 MeV/c2 ▪ Includes conversion 1 eV = 1.602 176 x 10-19 J 15 Some Masses in Various Units -26 16 Prefixes 17 18 Size of Nucleus, Final Since the time of Rutherford, many other experiments have concluded the following: ▪ Most nuclei are approximately spherical. ▪ Average radius is raA 13 ▪ a = 1.2 x 10-15 m ▪ A is the mass number 19 Density of Nuclei The volume of the nucleus (assumed to be spherical) is directly proportional to the total number of nucleons. This suggests that all nuclei have nearly the same density. Nucleons combine to form a nucleus as though they were tightly packed spheres. 𝑀 𝐴𝑚𝑛 𝜌= = 𝑉 4 𝜋𝑟 3 3 mn = 1.67×10-27 kg r a A13 𝝆 ≅ 2.3×1017 kg/m3 20 Nuclear Stability There are very large repulsive electrostatic forces (coulomb force) between protons. ▪ These forces should cause the nucleus to fly apart. The nuclei are stable because of the presence of another, short-range force, called the nuclear force. ▪ This is an attractive force that acts between all nuclear particles. ▪ The nuclear attractive force is stronger than the Coulomb repulsive force at the short ranges within the nucleus. ▪ Unlike the Coulomb and Newton’s gravitational laws, the fundamental law and constants to describe the nuclear force is not known! Full understanding of it requires quantum mechanics! 21 Features of the Nuclear Force Attractive force that acts between all nuclear particles Very short range ▪ It falls to zero when the separation between particles exceeds about several fermis. Independent of charge ▪ The nuclear force on p-p, p-n, n-n are all the same ▪ Does not affect electrons 22 Nuclear Stability, cont. Light nuclei are most stable if N = Z. Heavy nuclei are most stable when N > Z. ▪ Above about Z = 20 ▪ As the number of protons increases, the Coulomb force increases and so more neutrons are needed to keep the nucleus stable. No nuclei are stable when Z > 83. 23 Binding Energy The total energy of the bound system (the nucleus) is less than the combined energy of the separated nucleons. ▪ This difference in energy is called the binding energy of the nucleus. ▪ It can be thought of as the amount of energy you need to add to the nucleus to break it apart into its components. The binding energy can be calculated from conservation of energy and the Einstein mass-energy equivalence principle. When separate nucleons are combined to form a nucleus, the energy of the system is reduced. The change in energy is negative. The absolute value of the change in energy is the binding energy. 24 Marie Curie 1867 – 1934 Polish scientist Shared Nobel Prize in Physics in 1903 for studies in radioactive substances ▪ Shared with Pierre Curie and Becquerel Won Nobel Prize in Chemistry in 1911 for discovery of radium and polonium A family of Nobel Prize winners 25 Marie Curie, Irene Curie, Pierre Curie Radioactivity Radioactivity is the spontaneous emission of radiation. ▪ Discovered by Becquerel in 1896 ▪ Many experiments were conducted by Becquerel and the Curies. Experiments suggested that radioactivity was the result of the decay, or disintegration, of unstable nuclei. 26 Radioactivity – Types of Decay Three types of radiation can be emitted. ▪ Alpha particles () ▪ The particles are 4He nuclei. ▪ Beta particles () ▪ The particles are either electrons or positrons. ▪ A positron is the antiparticle of the electron. ▪ It is similar to the electron except its charge is +e. ▪ Gamma rays () ▪ The “rays” are high energy photons. ▪ It is an electromagnetic wave. 27 Distinguishing Types of Radiation All three types of radiation enter a region where there is a magnetic field. The gamma particles carry no charge. The alpha particles are deflected upward. The beta particles are deflected downward. ▪ A positron would be deflected FB = qv B upward, but would follow a different trajectory than the α due to its mass. 28 Penetrating Ability of Particles Alpha particles ▪ Barely penetrate a piece of paper Beta particles ▪ Can penetrate a few mm of aluminum Gamma rays ▪ Can penetrate several cm of lead 29 Terminology Notes Radiation is the term used historically for all emanations from a radioactive nucleus. Alpha and beta radiation are actually emissions of particles with nonzero rest energy (kinetic energy). ▪ Although these are not forms of electromagnetic radiation, the term radiation is still used. 30 The Decay Constant The number of particles that decay in a given time is proportional to the total number of particles in a radioactive sample. dN = − λN gives N = Noe − λt dt ▪ λ is called the decay constant and determines the probability of decay per nucleus per second. ▪ N is the number of undecayed radioactive nuclei present at time t. ▪ No is the number of undecayed nuclei at time t = 0. Exponential decay 31 Decay Rate The decay rate R of a sample is defined as the number of decays per second. dN R= = λN = Roe − λt dt ▪ Ro = Noλ is the decay rate at t = 0. ▪ The decay rate is often referred to as the activity of the sample. 32 Decay Curve and Half-Life The decay curve follows the equation N = Noe-λt. The half-life is also a useful parameter. ▪ The half-life is defined as the time interval during which half of a given number of radioactive nuclei decay. 33 Half-Live, cont. During the first half-life, ½ of the original material will decay. During the second half-life, ½ of the remaining material will decay, leaving ¼ of the original material remaining. Summarizing, the number of undecayed radioactive nuclei remaining after n half- lives is N = No (½)n ▪ n can be an integer or a non-integer. 34 Units The unit of radioactivity, R, is the curie (Ci) ▪ 1 Ci ≡ 3.7 x 1010 decays/s The SI unit of radioactivity is the becquerel (Bq) ▪ 1 Bq ≡ 1 decay/s ▪ Therefore, 1 Ci = 3.7 x 1010 Bq The most commonly used units of activity are the millicurie and the microcurie. 35 Applications of Nuclear Physics Processes of Nuclear Energy Generation Fission ▪ A nucleus of large mass number splits into two smaller nuclei. Fusion ▪ Two light nuclei fuse to form a heavier nucleus. Large amounts of energy are released in both cases. 37 Interactions Involving Neutrons Because of their charge neutrality, neutrons are not subject to Coulomb forces. As a result, they do not interact electrically with electrons or the nucleus. Neutrons can easily penetrate deep into an atom and collide with the nucleus. 38 Fast Neutrons A fast neutron has energy greater than approximately 1 MeV. During its many collisions when traveling through matter, the neutron gives up some of its kinetic energy. For fast neutrons in some materials, elastic collisions dominate. ▪ These materials are called moderators since they moderate the originally energetic neutrons very efficiently. ▪ Moderator nuclei should be of low mass so that a large amount of kinetic energy is transferred to them in elastic collisions. ▪ Materials such as paraffin and water are good moderators for neutrons. Elastic collision: An encounter between two bodies in which the total kinetic energy of the two bodies after the encounter is equal to their total kinetic energy before the encounter, i.e. the total kinetic energy is conserved. 39 Fission Example: 235U A typical fission reaction for uranium is 92 U → 56 Ba + 36 Kr + 3 ( 0 n ) n + 235 1 141 92 1 0 Ba: The chemical element Barium. Kr: The chemical element Krypton. 40 Chain Reaction Neutrons are emitted when 235U undergoes fission. ▪ An average of 3 neutrons These neutrons are then available to trigger fission in other nuclei. This process is called a chain reaction. ▪ If uncontrolled, a violent explosion can occur (e.g. atomic detonation). ▪ When controlled, the energy can be put to constructive use (e.g. nuclear power plants). The energy released in the fission process, is primarily in the form of the kinetic energy of the fission fragments (fast neurons, by- product atoms, etc.). It quickly converts into thermal energy (heat) in the surrounding medium (air, water, etc.). 41 Chain Reaction – Diagram Sr: Strontium Xe: Xenon Y: Yttrium I: Iodine Nb: Niobium Sb: Stibium 42 Nuclear Fusion Nuclear fusion occurs when two light nuclei combine to form a heavier nucleus. The mass of the final nucleus is less than the masses of the original nuclei. ▪ This loss of mass is accompanied by a release of energy. ▪ E = m.c2 ▪ c is the speed of light (3×108 m/s). 43 Fusion in the Sun These reactions occur in the core of a star (such as sun) and are responsible for the energy released by the stars. High temperatures are required to drive these reactions. ▪ Therefore, they are known as thermonuclear fusion reactions. 44 Advantages of a Fusion Reactor Inexpensive fuel source ▪ Water is the ultimate fuel source. ▪ If deuterium is used as fuel, 0.12 g of it can be extracted from 1 gal of water for about 4 cents. Comparatively few radioactive by-products are formed. Deuterium: Also known as heavy hydrogen (symbol D or 2H,) is one of three stable isotopes of hydrogen with a mass approximately twice that of the usual isotope, used as a fuel in fusion reactors or thermonuclear bombs. The three most stable isotopes of hydrogen. 7 isotopes exist for hydrogen in nature. 45 Radiation Damage Radiation absorbed by matter can cause damage. The degree and type of damage depend on many factors. ▪ Type and energy of the radiation ▪ Properties of the matter 46 Radiation Damage, cont. Radiation damage in the metals used in the reactors comes from neutron bombardment. ▪ They can be weakened by high fluxes of energetic neutrons producing metal fatigue. ▪ The damage is in the form of atomic displacements, often resulting in major changes in the properties of the material. Radiation damage in biological organisms is primarily due to ionization effects in cells that lead to DNA damage. ▪ Ionization disrupts the normal functioning of the cell. 47 Types of Radiation Damage in Cells Somatic damage is radiation damage to any cells except reproductive ones. ▪ Can lead to cancer at high radiation levels ▪ Can seriously alter the characteristics of specific organisms Genetic damage affects only reproductive cells. ▪ Can lead to defective offspring ▪ Radiation damage in cells is mainly caused by damage to the DNA in the cell’s nucleus. 48 Damage Dependence on Penetration Damage caused by radiation also depends on the radiation’s penetrating power. ▪ Alpha particles cause extensive damage, but penetrate only to a shallow depth. ▪ Due to their charge, they will have a strong interaction with other charged particles. ▪ Neutrons do not interact with material and so penetrate deeper, causing significant damage. ▪ Gamma rays can cause severe damage, but often pass through the material without interaction. 49 Units of Radiation Exposure The roentgen (R) is defined as ▪ That amount of ionizing radiation that produces an electric charge of 3.33 x 10-10 C in 1 cm3 of air under standard conditions. ▪ Equivalently, that amount of radiation that increases the energy of 1 kg of air by 8.76 x 10-3 J. rad (radiation absorbed dose) ▪ One rad is the amount of radiation that increases the energy of 1 kg of absorbing material (biological tissue) by 1 x 10-2 J. 50 More Units The RBE (Relative Biological Effectiveness) ▪ The number of rads of an standard x-radiation (250-keV x-ray) or gamma radiation that produces the same biological damage as 1 rad of the radiation being used. ▪ Accounts for type of particle (radiation) which the rad itself does not The rem (radiation equivalent in man) ▪ Defined as the product of the dose in rad and the RBE factor ▪ Dose in rem = dose in rad x RBE 51 RBE Factors, A Sample 52 Radiation Levels Natural sources – rocks and soil, cosmic rays ▪ Called background radiation ▪ About 0.13 rem/yr Upper limit suggested by US government ▪ 0.50 rem/yr ▪ Excludes background Occupational ▪ 5 rem/yr for whole-body radiation ▪ Certain body parts can withstand higher levels ▪ Ingestion or inhalation is most dangerous 53 Radiation Levels, cont. 50% mortality rate ▪ About 50% of the people exposed to a dose of 400 to 500 rem will die. New SI units of radiation dosages ▪ The gray (Gy) replaces the rad. ▪ The sievert (Sv) replaces the rem. 54 SI Units, Table 55 Other Applications of Radiation Tracing ▪ Radioactive particles can be used to trace chemicals participating in various reactions. ▪ Example, 131I to test thyroid action ▪ Also to analyze circulatory system ▪ Also useful in agriculture and other applications Materials analysis ▪ Neutron activation analysis uses the fact that when a material is irradiated with neutrons, nuclei in the material absorb the neutrons and are changed to different isotopes. 56 Other Applications of Radiation, cont. Radiation therapy ▪ Radiation causes the most damage to rapidly dividing cells. ▪ Therefore, it is useful in cancer treatments. Food preservation ▪ High levels of radiation can destroy or incapacitate bacteria or mold spores. 57 Medical Nuclear Physics The diagnostic and therapeutic applications of x-ray, gamma ray, neutron, electron, and charged-particle beams, and radiation from sealed radionuclide sources. The equipment associated with their production, use, measurement, calibration, and evaluation. The quality of images and effectiveness/safety of treatments resulting from the ionizing radiation production and use. Medical health physics associated with this subfield. 58 Discovery of X-rays On November 8, 1895, Wilhelm Conrad Röntgen (accidentally) discovered an image cast from his cathode ray generator. The first X-ray image (Rontgen’s wife’s hand) 59 Gamma Camera Scan Liver metastasis from prostate carcinoma [administration of Tc99m] Accumulates in areas of increased blood flow due to active bone metabolism, inflammation or the angiogenesis associated with tumors Tc = The chemical element Technetium. 60 Cell Killing By Ionizing Radiation 61 Radiation Therapy Modern Radiation Therapy Using High Energy X-rays and Electrons LINAC 62