Drug Elimination PDF
Document Details
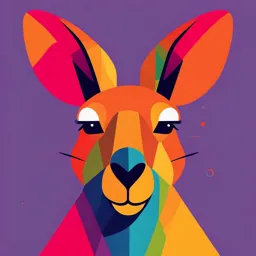
Uploaded by RighteousIguana
Tags
Summary
This document explains the processes involved in drug elimination. The different methods of drug elimination and the factors that affect them are explored. This document also details various methods of drug excretion and related concepts, e.g., glomerular filtration, active tubular secretion.
Full Transcript
Drug Elimination Excretion of drugs is defined as a process through which the drug and its metabolite(s) are permanently transferred to the external environment from the internal milieu. Apart from the kidneys, drug elimination occurs via various “nonrenal” pathways like the liver, intest...
Drug Elimination Excretion of drugs is defined as a process through which the drug and its metabolite(s) are permanently transferred to the external environment from the internal milieu. Apart from the kidneys, drug elimination occurs via various “nonrenal” pathways like the liver, intestine, lungs, skin, salivary glands, lacrimal glands, mammary glands, and semen Renal Drug Elimination Property of the Drug (Metabolite) The drugs or their metabolites that undergo renal route of elimination should be: Water soluble Non-volatile Small molecular size (500 Da) are exceptions. Though the negative charge in the glomerular basement repels anionic drugs, the main driving force for filtration of drugs is hydrostatic pressure of the blood flowing in glomerular capillaries. Creatinine, inulin, mannitol, and sodium thiosulfate are used to estimate the GFR. Majority of the drugs are excreted by glomerular filtration. Macromolecular drugs like biologicals (monoclonal antibodies) cannot be filtered and hence utilize nonrenal pathways for elimination. Glomerular filtration of drugs depends upon renal blood flow and status of protein binding of the drugs. Active Tubular Secretion Around 80% of the reabsorbed drug enters peritubular capillaries of the proximal tubules and is actively secreted into the tubular lumen. Hence, tubular secretion of drugs is the most effective method of renal drug elimination. Active secretion is a carrier-mediated process which requires energy for transportation against a concentration gradient. Therefore, active secretion can achieve maximal drug clearance. Two active tubular secretion mechanisms have been identified: – System for secretion of organic acids or anions (organic anion transport): penicillin, salicylates, furosemide, indomethacin, probenecid, and metabolites conjugated with glucuronic acid, glycine, and sulfates – System for secretion of organic bases or cations (organic cation transport): morphine, mecamylamine, hexamethonium, amiloride, triamterene, quinine, and endogenous amines such as catecholamines, choline, and histamine Para-aminohippurate (PAH) and iodopyracet are used to determine active secretion by the kidneys. Drugs that undergo active secretion have clearance >500 ml/ min. This is due to the occurrence of both glomerular filtration and tubular secretion. Changes in pH and status of plasma protein binding will not affect the active secretion as the bound drug dissociates rapidly once the unbound drug gets excreted. Hence, active secretion is independent of the status of plasma protein binding. Drugs with same ionic charge and carrier-mediated process for excretion compete with each other. Few examples are: – Probenecid competes with penicillin in active tubular secretion of an organic acid. This property can be exploited in conditions where a high dose of penicillins is required (like gonococcal infection), wherein, along with the administration of probenecid, the requirement of a penicillin dose is nearly halved. – Probenecid also competes with para-aminosalicylic acid (PAS), PAH, and 17-ketosteroids secretion doubling their plasma concentrations. – Inhibition of secretion of nitrofurantoin by probenecid is detrimental in the case of urinary tract infection where nitrofurantoin fails to reach the site of action. – Metformin and pyrimethamine compete with each other for active secretion via hepatic organic cation transporter 2 (hOCT2). Tubular Reabsorption Tubular reabsorption can be either an active process or passive process. Active tubular reabsorption is seen with endogenous substances such as electrolytes, glucose, vitamins, amino acids, and uric acid and few drugs like oxipurinol. Passive tubular reabsorption is seen with a large number of drugs and few endogenous substances. The determinants of passive tubular reabsorption are as follows: – Lipophilicity of drugs – pH of urine – pKa of drug – Urine flow rate The passive tubular reabsorption of drugs is based on the polarity of the drug which in turn depends on the pH of urine and pKa of the drug. As most of the drugs are either weak acids or weak bases, the pH of urine and pKa of drug play a major role in influencing the tubular reabsorption of the drug. Physiologically, the pH of human urine will be in between the range of 4.5– 7.5. with this range of pH in urine: – Very weak acidic nonpolar drugs like phenytoin (pKa >8.0) or very weak basic nonpolar drugs like propoxyphene (pKa 12.0) are totally ionized in the entire range of urinary pH. These drugs are never reabsorbed and are excreted at a higher rate and do not depend upon the urinary pH. – Drugs which are polar in their unionized form also will not be reabsorbed and are excreted at a higher rate. Their rate of excretion is the sum of filtration rate and rate of active secretion, e.g., penicillins and gentamicin. acidic drugs with pKa between 3.0 and 8.0 (e.g., NSAIDs) and basic drugs with pKa between 6.0 and 12.0 (e.g., morphine, TCAs) depend greatly on the urinary pH for the extent of reabsorption. Since their pKa values are within the range of human urinary pH, their reabsorption magnitude can be as minimum as 0% and as maximum as 100 When the urinary flow rate is considered, the passive reabsorption of polar drugs is not affected by the urine flow rate. In weak acidic and weak basic drugs, the extent of reabsorption is inversely proportional to the urine flow rate. Since most of the drugs used clinically are weakly basic and weakly acidic in nature, the principle of “forced diuresis” is used in a poisoning situation. Forced diuresis with increased urinary flow rate will decrease the passive reabsorption of drugs. Moreover, based on the nature of polarity and pKa of drugs, urine can be acidified or alkalinized resulting in the formation of ionized drugs in the tubular lumen in order to prevent its passive reabsorption. Nonrenal Drug Elimination Hepatic Drug Elimination The process of biliary drug excretion is similar to active renal secretion because bile secretion is capacity- limited and can be saturated. The capacity of the liver to clear the plasma from drug via bile (biliary clearance) can be expressed as Biliary clearance Bile flow Bile drugconcentration Plasma drugc = ∗ oncentration Biliary clearance of the drug is very less for drugs whose biliary concentration is less than that in plasma, and drugs with higher biliary concentration than plasma will have higher biliary clearance. Based on the bile or plasma concentration, the compounds that are excreted in bile can be divided into: – Group A: the ratio of bile to plasma concentration is approximately 1, e.g., glucose, sodium, and potassium ions. – Group B: the ratio of bile to plasma concentration is >1 (10– 1000), e.g., bile salts, bilirubin, and creatinine. – Group C: the ratio of bile to plasma concentration is – Drugs with highly polar functional groups like – COOH or – NH4 + result in unchanged excretion in bile. – Route of administration (oral route of drug administration has more biliary excretion than the parenteral route of drug administration). – Presence of food (protein- and fat-rich food increases the bile secretion and, thereby, increases the biliary clearance of drugs). Several drugs which are excreted unchanged in bile, and drugs with glucuronide and glutathione conjugation after hydrolyzing the conjugates can be reabsorbed via intestine and undergo enterohepatic circulation. Enterohepatic circulation is observed with endogenous compounds like vitamin B12, vitamin D, folic acid, steroids, and bile salts. Drugs like carbenoxolone, oral contraceptives, indomethacin, mycophenolic acid, rifampicin, and chlorpromazine have prolonged half-life due to the enterohepatic circulation. Any alteration in microbial flora by oral antibiotics will significantly affect the enterohepatic circulation process and thereby causes failure of therapy. The compound sulfobromophthalein is commonly used to assess the active biliary excretion capacity of the liver. Pulmonary Drug Elimination The pulmonary drug elimination is an important aspect in anesthesiology, as gaseous and volatile substances are eliminated by the pulmonary system. Systemic drugs are excreted by simple passive diffusion from plasma with higher concentration into the alveolar space with lower concentration, e.g., anesthetic inhaled agents like halothane, nitrous oxide, fluranes, and ethanol.. Elimination of drugs by the lungs depends upon the respiratory rate, pulmonary blood flow, pathological state of the lungs, solubility of drugs in blood, and physiochemical nature of the drugs. Drugs with high blood solubility like ethanol are excreted slowly by the lungs, and drugs with low blood solubility like nitrous oxide are excreted at the same rate of absorption. Topically administered drugs are eliminated by local mucociliary clearance, macrophage uptake, and enzymatic degradation. The lungs also contribute to the metabolism of drugs (refer to Chap. 7) to some extent. Salivary Drug Elimination The excretion nature of drugs via saliva is by passive diffusion and active secretion process. The pH of saliva varies from 5.8 to 8.4, and hence the salivary excretion of drugs can be predictable by pH-partition hypothesis. Lipid-soluble drugs and unionized drugs are excreted passively in the saliva. Basic drugs are excreted more in saliva as compared to the acidic drugs because the mean salivary pH is around 6.4 which is acidic when compared to the plasma. The ratio of salivary concentration to plasma concentration of drugs is almost constant always for drugs like carbamazepine, theophylline, and caffeine. Hence, based on the salivary concentration, the approximate concentration of drug in the blood can be estimated. Lithium, penicillin, and phenytoin are actively secreted in saliva, and hence, the concentration of drugs is two- to threefold higher than the plasma concentration. Drugs with dye nature are excreted unchanged in the saliva and cause discoloration of the oral mucosa and tongue, e.g., rifampicin. Drug Elimination by Mammary Gland The pH of human milk ranges from 6.4 to 7.6 with a mean pH of 7.0. Majority of drugs are excreted into the breast milk by passive diffusion. However, drugs like cimetidine and ranitidine utilize active transport for the cations. Acidic drugs like nitrofurantoin achieve 26 times higher concentration in milk than the plasma indicating the involvement of the active cationic transport. Benzylpenicillin concentration in the milk can be reduced by coadministration of probenecid suggesting the blockade of active secretion of penicillin by the probenecid in the mammary gland. The ratio between the concentration of drug in milk to This MP ratio is a time-dependent parameter and can be influenced by various parameters like: – Drug ionization and plasma protein binding (drugs that are more likely to be transferred into breast milk are free and unbound to proteins) – Molecular weight (low molecular weight drugs are highly transferred into breast milk) – Solubility of drugs in lipids (lipid-soluble drugs enter into breast milk at a higher rate) – pH of milk and plasma (weakly alkaline drugs achieve a higher concentration in the breast milk than the weak acidic drugs) The property of elimination of drug via breast milk gains significance when lactation for an infant is considered. The exposure of the infant to the drug via breast milk can be estimated by exposure index (%). Exposure index (%) = 100 × MP ratio × (milk intake by infant/infant drug clearance Drugs with low rates of clearance can result in a significant degree of drug exposure in infants via breast milk (>10% of exposure index). Drug Elimination Drug Elimination by the Skin Drug and its metabolite elimination by skin are usually by passive diffusion. In some cases, this route of elimination is responsible for urticaria, dermatitis, and other hypersensitivity reactions due to the drugs. Some drugs excreted via skin are benzoic acid, methamphetamine, rifampicin, salicylic acid, alcohol, and antipyrine and heavy metals like lead, mercury, and arsenic. Palmar-plantar erythrodysesthesia which occurs in doxorubicin therapy could be attributed to excretion of doxorubicin by sweat glands, penetration of the drug into stratum corneum, and forming the free radical-induced damage Methamphetamine can be detected in sweat within 2 h of ingestion and will be excreted more than 1 week after stopping from multiple uses. This property can be used as an alternative method for the detection of abuse of methamphetamine. Moreover, many drugs with abuse potential like ethanol, buprenorphine, cocaine, and cannabinoids are also excreted via sweat glands and can be quantified for abuse activity. Drug Elimination by Gastrointestinal System Water soluble and ionized forms of drugs are excreted in feces. Drugs like codeine and morphine are actively secreted through the gastric mucosa at a pH of 1–3. Gastric lavage is done in parenteral opioid poisoning to prevent reabsorption of morphine from gastric lumen. P-glycoprotein expressed in the intestinal mucosa is responsible for excretion of anticancer drugs like docetaxel. In rats, intestinal secretion by P-glycoprotein is the major route of elimination for the anthelminthic drug ivermectin. Verapamil, an inhibitor of P-glycoprotein, reduces the fecal concentration of ivermectin. Drug Elimination in Semen Various drugs are excreted in the male genital system through the prostate and seminal vesicles and hence can be recovered in semen. Antibiotics like methicillin, cephalexin, cephalothin, tetracycline, ciprofloxacin, norfloxacin, sulfamethoxazole, and clindamycin attain a higher concentration in semen than that of plasma. Antibiotics like ampicillin, oxacillin, erythromycin, chloramphenicol, nalidixic acid, aztreonam, kanamycin, and metronidazole attain very least concentration in the semen. E. Pichai and M. Lakshmanan 125 Though antiepileptic drugs like phenytoin and sodium valproate are excreted in semen, significant changes in sperm motility are not observed even on chronic use. The following are the factors which determine the clearance of the drug: – Physicochemical properties of the drugs: molecular size, pKa, and lipid solubility. – Plasma concentration of the drug: glomerular filtration and passive tubular reabsorption are dependent on the plasma concentration of the drug and show saturation point, while active secretion shows a linear relationship with the plasma concentration. – Plasma protein binding: free drugs are easily excreted in the urine and bound drugs cannot. – Blood flow to the kidneys: glomerular filtration and active secretion are dependent on the renal blood flow. Elimination of the drugs is enhanced by increasing the contact of the drug with the secretory sites which in turn is dependent on the renal blood flow. – Sex: clearance is lesser in females than males by approximately 10–20%. – Age: newborn and elderly population have a clearance of 30–40% lesser than that of the adults. – Drug interactions leading to changes in the active secretion, urine pH, and renal blood flow can alter the drug clearance. – Renal disease status. Clearance is the single most parameter which determines the maintenance dose of any drug. This is because, at steady-state concentration, the dosing rate should be equal to the rate of elimination, i.e., Dosing rate = Rate of elimination = Clearance × Target plasma concentration. Hence, if the target concentration is fixed with the clearance value, the dosing rate can be calculated. Elimination Half-Life (t1/2) or Beta Half-Life The elimination half-life of drug (also called as the biological half-life) can be simply stated as the time interval in which the amount of drug in the body is reduced to one-half from its initial value. The half-life of the drug is commonly misunderstood as the “equivalence of clearance” which is completely inaccurate. The steady-state half-life of the drug markedly differs from terminal half-life in drugs like gentamicin (2–3 h after single administration versus 53 h on continuous infusion), indomethacin (2.4 h versus 120 h), and various anesthetic agents. In the case of radionuclides used in radiotherapy, the half- life of the drug is the combination of both physical (radioactive decay) and the biological half-life. This half-life is called as the effective half-life.