18_Fundamentals of Spectrophotometry.pptx
Document Details
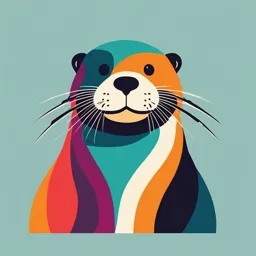
Uploaded by RationalPiccolo2740
Tags
Full Transcript
18 FUNDAMENTALS OF SPECTROPHOTOMETRY UV-visible spectrophotometry Infrared spectroscopy KEYWORD Spectrum/Spectra (spectro- (prefix)) The word was first used scientifically in optics to describe the rainbow of colors...
18 FUNDAMENTALS OF SPECTROPHOTOMETRY UV-visible spectrophotometry Infrared spectroscopy KEYWORD Spectrum/Spectra (spectro- (prefix)) The word was first used scientifically in optics to describe the rainbow of colors in visible light after passing through a prism A spectrum (plural spectra or spectrums) is a condition that is not limited to a specific set of values but can vary, without steps, across a continuum. As scientific understanding of light advanced, it came to spectrum The spectrum in a rainbow apply to the entire electromagnetic spectrum. It thereby became a mapping of a range of magnitudes (wavelengths) to a range of qualities, which are the perceived "colors of the rainbow" and other properties which correspond to wavelengths that lie outside of the visible light spectrum. KEYWORD Spectro-scopy scopy: observe, examine, view Spectroscopy is the study of the interaction between matter and electromagnetic radiation as a function of the wavelength or frequency of the radiation. In simpler terms, spectroscopy is the precise study of color as generalized from visible light to all bands of the electromagnetic spectrum Spectroscopy: the study of Electromagnetic spectrum of atoms (the study of Electromagnetic interaction in - Type of atoms - Amount of atom PROPERTIES OF LIGHT Light is describe as both particles and waves Light waves consist of perpendicular, oscillating electric and magnetic fields. For simplicity, a plane-polarized wave is shown in Figure 18-1. The electric field is in the xy plane, and the magnetic field is in the xz plane. Wavelength, l, is the crest-to-crest distance between waves. Frequency, n, is the number of complete oscillations that the wave makes each second. The unit of frequency is 1/second. One oscillation per second is called one hertz (Hz). A frequency of 106 s-1 is therefore said to be 106 Hz, or 1 megahertz (MHz). One photon Electro-magnetic Radiation Motion detection Night vision LIGHT IS FASTER THAN SOUND The speed of light, c = 3 × 108 m/sec You see lightning before hearing the thunder LIGHT CAN BOUNCE (REFLECTION) AND BEN (REFRACTION) VISION AND COLOR What is color? Why does some light appear red and other light appear blue? Color is how we perceive the energy of light. This definition of color was proposed by Albert Einstein. All of the colors in the rainbow are light of different energies. Red light has the lowest energy we can see, and violet light the highest energy. As we move through the rainbow from red to yellow to blue to violet, the energy of the light increases. HOW THE HUMAN EYE SEES LIGHT HOW WE SEE COLORS? The additive color process HOW THINGS APPEAR TO BE DIFFERENT COLORS ABSORPTION OF LIGHT What happens when a molecule absorb light? When a molecule absorbs a photon, the energy of the molecule increases. We say that the molecule is promoted to an excited state (Figure 18-3). If a molecule emits a photon, the energy of the molecule is lowered. The lowest energy state of a molecule is called the ground state. Figure 18-2 indicates that microwave radiation stimulates rotation of molecules when it is absorbed. Infrared radiation stimulates vibrations. Visible and ultraviolet radiation promote electrons to higher energy orbitals. WHEN BEER’S LAW FAILS Intermolecu lar interaction MEASURING ABSORBANCE FIGURE 18-6 Common cuvets for visible and ultraviolet spectroscopy. Flow cells permit continuous fl ow of solution through the cell. In the thermal cell, liquid from a constant temperature bath flows through the cell jacket to maintain a desired temperature. [Information from A. H. Thomas Co., Philadelphia, PA.] SINGLE BEAM VS DOUBLE BEAM INSTRUMENT SINGLE BEAM SPECTROPHOTOMETER SINGLE BEAM VS DOUBLE BEAM INSTRUMENT SINGLE BEAM SPECTROPHOTOMETER DUAL BEAM SPECTROPHOTOMETER DIFFUSED REFLECTANCE SPECTROSCOPY For solid sample OLD VS MODERN INSTRUMENT New instruments are most precise (reproducible) at intermediate levels of absorbance (A≈ 0.3 to 2). SERUM IRON DETERMINATION Potential issues: interference of Cu+ SPECTROPHOTOMETRIC TITRATION WHAT HAPPENS WHEN A MOLECULE ABSORBS LIGHT? When a molecule absorbs a photon, the molecule is promoted to a more energetic excited state (Figure 18-3). Conversely, when a molecule emits a photon, the energy of the molecule falls by an amount equal to the energy of the photon. For example, consider formaldehyde in Figure 18-12. In its ground state, the molecule is planar, with a double bond between carbon and oxygen. From the electron dot description of formaldehyde, we expect two pairs of nonbonding electrons to be localized on the oxygen atom. The double bond consists of a sigma bond between carbon and oxygen and a pi bond made from the 2py (out-of-plane) atomic orbitals of carbon and oxygen. Atomic Orbital (AO) Atomic Orbital (AO) Molecular Orbital Theory (MO) Hybridizati on sp2 MOLECULAR ORBITAL P-P INTERACTION VIBRATIONAL AND ROTATIONAL STATES OF FORMALDEHYDE Absorption of visible or ultraviolet radiation promotes electrons to higher energy orbitals in formaldehyde. Infrared and microwave radiation are not energetic enough to induce electronic transitions, but they can change the vibrational or rotational motion of the molecule. MOLECULAR VIBRATIONS Vibration mode 52 VIBRATIONS OF A LINEAR MOLECULE 53 VIBRATION OF A METHYLENE GROUP (–CH2-) 54 VIBRATION OF A METHYLENE GROUP (–CH2-) Symmetrical stretching Asymmetrical stretching Scissoring (bending) Rocking Wagging Twisting ROTATION AND VIBRATION OF FORMALDEHYDE Vibration Rotation COMBINED ELECTRONIC, VIBRATIONAL, AND ROTATIONAL TRANSITIONS In general, when a molecule absorbs light having sufficient energy to cause an electronic transition, vibrational and rotational transitions—that is, changes in the vibrational and rotational states— occur as well. Formaldehyde can absorb one photon with just the right energy to cause the following simultaneous changes: (1) a transition from the S0 to the S1 electronic state; (2) a change in vibrational energy from the ground vibrational Ban Ban d state of S0 to an excited vibrational state of S1; and d (3) a transition from one rotational state of S0 to a different rotational state of S1. Electronic absorption bands are usually broad (Figure 18-9) because many different vibrational and rotational levels are available at slightly different energies. A molecule can absorb photons with a wide range of energies and still be promoted from the ground electronic state to one particular excited electronic state. Broad spectrum observed in XPS spectroscopy WHAT HAPPENS TO ABSORBED ENERGY? Suppose that absorption promotes a molecule from the ground electronic state, S 0, to a vibrationally and rotationally excited level of the excited electronic state S 1 (Figure 18-16). Usually, the first process after absorption is vibrational relaxation to the lowest vibrational level of S1. In this nonradiative transition, labeled R1 in Figure 18-16, vibrational energy is transferred to other molecules (solvent, for example) through collisions, not by emission of a photon. The net effect is to convert part of the energy of the absorbed photon into heat spread throughout the entire medium. At the S1 level, several events can happen. The molecule could enter a highly excited vibrational level of S0 having the same energy as S1. This is called internal conversion (IC). From this excited state, the molecule can relax back to the ground vibrational state and transfer its energy to neighboring molecules through collisions. This nonradiative process is labeled R2. If a molecule follows the path A–R1–IC–R2 in Figure 18-16, the entire energy of the photon will have been converted into heat. Alternatively, the molecule could cross from S1 into an excited vibrational level of T1. Such an event is known as intersystem crossing (ISC). After the nonradiative vibrational relaxation R3, the molecule fi nds itself at the lowest vibrational level of T 1. From here, the molecule might undergo a second intersystem crossing to S0, followed by the nonradiative relaxation R4. All processes mentioned so far simply convert light into heat. A molecule could also relax from S1 or T1 to S0 by emitting a photon. The radiational transition S1 → S0 is called fluorescence (Box 18-2), and the radiational transition T1 → S0 is called phosphorescence. The relative rates of internal conversion, intersystem crossing, fluorescence, and phosphorescence depend on the molecule, the solvent, and conditions such as temperature and pressure. The energy of phosphorescence is less than the energy of fluorescence, so phosphorescence comes at longer wavelengths Fluorescence than fluorescence and phosphorescence are (Figure relatively 18-17). rare. Molecules generally decay from the excited state by nonradiative transitions. The lifetime of fluorescence is 10-8 to 10-4 s. The lifetime of phosphorescence is longer (10-4 to 102 s) because phosphorescence involves a change in spin quantum numbers (from two unpaired electrons to no unpaired electrons), which is improbable. A few materials, such as strontium aluminate doped with europium and dysprosium (SrAl2O4:Eu:Dy) phosphoresce for hours after exposure to light. One application for this material is in signs leading to emergency exits when power LUMINESCENCE RELATION BETWEEN ABSORPTION AND EMISSION SPECTRA Absorption and emission spectra and why the spectra are roughly mirror images of each other The l0 transitions in Figure 18-19 (and later in Figure 18-23) do not exactly overlap. In the emission spectrum, l0 comes at slightly lower energy than in the absorption spectrum. The reason is explained in Figure 18-21. A molecule absorbing radiation is initially in its electronic ground state, S0. This molecule possesses a certain geometry EXCITATION AND EMISSION SPECTRA An excitation spectrum is measured by varying the excitation wavelength and Be careful with Raman, Rayleigh, Mie Scattering measuring emitted light at one particular wavelength (λem). An excitation spectrum is a graph of emission intensity versus excitation wavelength (Figure 18-23). An excitation spectrum looks very much like an absorption spectrum because, the greater the absorbance at the excitation wavelength, the more molecules are promoted to the excited state and the more emission will be observed. In emission spectroscopy, we measure emitted radiation, rather than the fraction of incident radiation striking the detector SCATTERING LUMINESCENCE INTENSITY the irradiance (W/m2) incident on the sample cell is P0. Some is absorbed over the pathlength b1, so the irradiance striking the central region of the cell is: Equation 18-14 says that, at low concentration, emission intensity is proportional to analyte concentration. Data for anthracene in Figure 18-24 are linear below 1026 M. Blank samples invariably scatter light and must be run in every analysis. Equation 18-14 tells us that doubling the incident irradiance (P0) will double the emission intensity (up to a point). In contrast, doubling P0 has no effect on absorbance, which is a ratio of two intensities. The sensitivity of a luminescence measurement can be increased by more than a factor EXAMPLE: FLUORIMETRIC ASSAY OF SELENIUM IN BRAZIL NUTS To measure selenium in Brazil nuts, 0.1 g of nut is digested with 2.5 mL of 70 wt% HNO3 in a Teflon bomb (Figure 28-7) in a microwave oven. Hydrogen selenate (H2SeO4) in the digest is reduced to hydrogen selenite (H2SeO3) with hydroxylamine (NH2OH). Selenite is then derivatized to form a fluorescent product that is extracted into cyclohexane. Maximum response of the fluorescent product was observed with an excitation wavelength of 378 nm and an emission wavelength of 518 nm. In Figure 18-25, emission is proportional to concentration only up to ~ 0.1 mg Se/mL. Beyond 0.1 mg Se/mL, the response becomes curved, eventually reaches a maximum, and finally decreases with increasing concentration as self-absorption dominates. Equation 18-12 predicts this behavior. LUMINESCENCE IN ANALYTICAL CHEMISTRY THANKS