Applications of Spectrophotometry PDF
Document Details
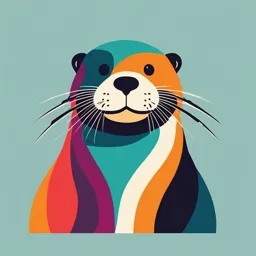
Uploaded by RationalPiccolo2740
Tags
Summary
This document presents different applications of spectrophotometry, covering a range of analytical techniques, from the analysis of mixtures and the concept of isosbestic points to methods of measuring equilibrium constants and calculating molar absorptivity. Concepts of flow and sequential injection methodology are also described.
Full Transcript
19 APPLICATIONS OF SPECTROPHOTOMETRY ANALYSIS OF A MIXTURE Consider two chemical species X and Y whose visible absorption spectra are shown in Figure 19-1a. The absorbance at any wavelength of a solution containing X and Y is the sum of absorbances of X and Y at that wavelength, as s...
19 APPLICATIONS OF SPECTROPHOTOMETRY ANALYSIS OF A MIXTURE Consider two chemical species X and Y whose visible absorption spectra are shown in Figure 19-1a. The absorbance at any wavelength of a solution containing X and Y is the sum of absorbances of X and Y at that wavelength, as shown by the dashed line in Figure 19-1a. For a solution containing any number of species, the absorbance at any wavelength is the sum of absorbances of all species in the solution. where ε is the molar absorptivity of each species at the wavelength in question and b is the cell pathlength (Figure 18-5). If we know the spectra of the pure components, we can mathematically disassemble the spectrum of a mixture into those of its components. WHAT TO DO WHEN THE INDIVIDUAL SPECTRA OVERLAP Figure 19-2 shows individual spectra of the H2O2 complex of 1.32 mM titanium(IV) and the H2O2 complex of 1.89 mM vanadium(V). The spectrum of an unknown mixture of the two components is also shown. We will apply a least-squares analysis of Equation 19-1 with two components to fi nd out how much Ti(IV) and how much V(V) are present. LET’S PRACTICE! WHAT TO DO WHEN THE INDIVIDUAL SPECTRA ARE WELL RESOLVED SOLVING SIMULTANEOUS LINEAR EQUATIONS WITH EXCEL Excel solves systems of linear equations with a single statement. If the following matrix mathematics below is unfamiliar to you, disregard it. The important result is the template in Figure 19-4 for solving simultaneous equations. You can use this template by following the instructions in the last paragraph of this section, even if the math is not familiar. Please practice and submit your excel file ISOSBESTIC POINTS Often one absorbing species, X, is converted into another absorbing species, Y, in the course of a chemical reaction. This transformation leads to an obvious behavior shown in Figure 19-5. If the spectra of pure X and pure Y cross each other at any wavelength, then every spectrum recorded during this chemical reaction will cross at that same point, called an isosbestic point. An isosbestic point is good evidence that only two principal species are present MEASURING AN EQUILIBRIUM CONSTANT To measure an equilibrium constant, we measure concentrations (actually activities) of species at equilibrium. Spectrophotometry can be used for this purpose. We examine a graphical procedure called the Scatchard plot that is widely used in biochemistry and then give a more powerful procedure using Excel Solver. SNATCHARD PLOT SCATCHARD PLOT A MORE GENERAL PROCEDURE WITH EXCEL SOLVER As increments of protein (P) are added to a fixed quantity of ligand (X = neutral red), absorption of X at 450 nm decreases while product (PX) absorption at 545 nm increases. Both absorptions are from the neutral red chromophore free in solution or bound to the protein. Protein P without X has no visible absorption. Cells B16:B26 in Figure 19-8 give the total concentration of ligand (X0 =[X] + [PX]) and cells C16:C26 give the total concentration of protein (P0 = [P] + [PX]). Observed absorbance (Aobs) at the PX peak wavelength of 545 nm appears in cells D16:D26. The final curve in Figure 19-7 represents a mixture of PX, P, and unreacted X. We can calculate the molar absorptivity (εX) for X at 545 nm from the absorbance of pure X shown by the black dot in Figure 19-7. With a pathlength of b = 1.000 cm, εX = A545/bX0 5 (0.0264)/(1.000 cm x 8.4 µM) = 3.143 x 103 M-1 cm-1. For subsequent calculations, we retain extra, insignificant digits. We do not know the molar absorptivity (εPX) of product PX because it is found in an unknown mixture with X. Follow the instruction and submit your result! THE METHOD OF CONTINUOUS VARIATION FLOW INJECTION ANALYSIS AND SEQUENTIAL INJECTION In flow injection analysis, a liquid sample is injected into a continuously flowing liquid carrier containing a reagent that reacts with the sample. Additional reagents might be added further downstream. As sample flows from the injector to the detector, the sample zone broadens and reacts with reagent to form a product to which the detector responds. Advantages of flow injection over “batch processes” in which individual samples are separately analyzed include speed, automation of solution handling, and low cost. It is normal for flow injection to handle 100 samples per hour. Autosamplers holding hundreds of samples are essential for automated analysis. Flow injection is a workhorse in many soil and water analysis labs, which routinely measure large numbers of samples. SEQUENTIAL INJECTION Sequential injection is distinguished from flow injection by flow programming and flow reversal under computer control. Flow is not continuous. Smaller volumes of reagents are required and less waste is generated than in flow injection. Sequential injection has been miniaturized to the point where it is also called a “lab-on-a-valve.” Miniaturization and discontinuous flow reduce the consumption of expensive reagents such as enzymes and antibodies for biochemical assays. Sequential injection is used for continuous monitoring of environmental and industrial processes, even at remote locations. IMMUNOASSAYS An important application of light absorption and emission is in immunoassays, which employ antibodies to detect analyte. An antibody is a protein produced by the immune system of an animal in response to a foreign molecule called an antigen. An antibody recognizes a specific antigen. The formation constant for the antibody-antigen complex is large, whereas the binding of the antibody to other molecules is weak. Figure 19-19 illustrates the principle of an enzyme-linked immunosorbent assay, abbreviated ELISA in biochemical literature. Antibody 1, which is specific for the analyte of interest (the antigen), is bound to a polymeric support. In steps 1 and 2, analyte is incubated with the polymer-bound antibody to form a complex. The fraction of antibody sites that bind analyte increases with increasing concentration of analyte in the unknown. The surface is then washed to remove unbound substances. In steps 3 and 4, the antibody-antigen complex is treated with antibody 2, which recognizes a different region of the analyte. Antibody 2 was specially prepared for the immunoassay by covalent attachment of an enzyme that will be used later in the process. Again, excess unbound substances are washed away. The enzyme attached to antibody 2 is critical for quantitative analysis. Figure 19-20 shows two ways in which the enzyme can be used. The enzyme can transform a colorless reactant into a colored product. Because one enzyme molecule catalyzes the same reaction many times, many molecules of colored product are created for each analyte molecule. The enzyme thereby amplifies the signal in the chemical analysis. The higher the concentration of analyte in the original unknown, the more enzyme is bound and the greater the extent of the enzyme-catalyzed reaction. Alternatively, the enzyme can convert a nonfluorescent reactant into a fluorescent product. Colorimetric and fluorometric enzyme-linked immunosorbent assays are sensitive to less than a nanogram of analyte. The home pregnancy test described at the opening of Chapter 0 is an immunoassay of a placental protein in urine. Aptamers (Box 17-5) can be used in much the same manner as antibodies in chemical analysis.19 IMMUNOASSAYS IN ENVIRONMENTAL ANALYSIS Commercial immunoassay kits are available for screening and analysis of pesticides, industrial chemicals, explosives, and microbial toxins at the parts per trillion to parts per million levels in groundwater, soil, and food.20 An advantage of screening in the fi eld is that uncontaminated regions that require no further attention are readily identified. An immunoassay can be 20–40 times less expensive than a chromatographic analysis and can be completed in 0.3–3 h in the fi eld, using 1-mL samples. Chromatography generally must be done in a lab and might require several days, because analyte must first be extracted or concentrated from liter- quantity samples to obtain a sufficient concentration. TIME-RESOLVED FLUORESCENCE IMMUNOASSAYS TIME-RESOLVED FLUORESCENCE IMMUNOASSAYS The sensitivity of fluorescence immunoassays can be enhanced by a factor of 100 (to detect 10-13 M analyte) with time-resolved measurements of luminescence from the lanthanide ion Eu3+. Organic chromophores such as fluorescein are plagued by background fluorescence at 350–600 nm from solvent, solutes, and particles. This background decays to a negligible level within 100 ms after excitation. Sharp luminescence at 615 nm from Eu31, however, has a much longer lifetime, falling to 1/e (= 37%) of its initial intensity in approximately 700 ms. In a time-resolved fluorescence measurement (Figure 19-23), luminescence is measured between 200 and 600 ms after a brief laser pulse at 340 nm. The next pulse is flashed at 1 000 ms, and the cycle is repeated approximately 1 000 times per second. Rejecting emission within 200 ms of the excitation eliminates most of the background fluorescence. Figure 19-24 shows how Eu31 can be incorporated into an immunoassay. A chelating group that binds lanthanide ions is attached to antibody 2 in Figure 19-19. While bound to the antibody, Eu31 has weak luminescence. After all steps in Figure 19-19 have been completed, the pH of the solution is lowered in the presence of a chelator that extracts Eu31 into solution. Strong luminescence from the soluble metal ion is then easily detected by a time-resolved measurement. SENSORS BASED ON LUMINESCENCE QUENCHING When a molecule absorbs a photon, it is promoted to an excited state from which it can lose energy as heat or it can emit a lower energy photon (Figure 18-16). Box 19-1 describes how absorbed light can be converted into electricity. In this section, we discuss how excited-state molecules can serve as chemical sensors (Figure 19-25). LUMINESCENT INTRACELLULAR O2 SENSOR