Kidney Function - Loop of Henle and Water Reabsorption PDF
Document Details
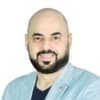
Uploaded by DR.MOATAZ
Tags
Summary
This document explains the process of kidney function focusing on the loop of Henle and water reabsorption. It describes how the loop of Henle acts as a counter-current multiplier, concentrating urine and enabling efficient water conservation. Illustrations highlight the key parts and processes.
Full Transcript
# Extension 14.5: Kidney Function – Loop of Henle and Reabsorption of Water ## The Loop of Henlé - Is a hairpin-shaped tubule that extends into the medulla of the kidney. - Responsible for creating conditions in the surrounding interstitial fluid that lead to the reabsorption of water from the dis...
# Extension 14.5: Kidney Function – Loop of Henle and Reabsorption of Water ## The Loop of Henlé - Is a hairpin-shaped tubule that extends into the medulla of the kidney. - Responsible for creating conditions in the surrounding interstitial fluid that lead to the reabsorption of water from the distal convoluted tubule and the collecting duct. - Results in concentrating the urine so it has a lower water potential than the blood. - The concentration of the urine produced is directly related to the length of the loop of Henlé. - **Short in mammals whose habitats are in or by water** (e.g. beavers) - **Long in those whose habitats are dry regions** (e.g. kangaroo rat) ## The Loop of Henlé Has Two Regions: - **Descending limb**: narrow, with thin walls that are highly permeable to water. - **Ascending limb**: which after a short distance is wider, with thick walls that are impermeable to water. - The loop of Henlé acts as a counter-current multiplier. - To understand how this works, it is necessary to consider the following sequence of events using Figure 1, to which the numbers refer. ### Diagram of the Loop of Henlé: | | | | | | | | :------------------------------------------------------- | :------- | :------- | :------- | :------- | :------- | | **Filtrate from proximal convoluted tubule** (300) | CORTEX | | | | | | **Ascending limb with walls impermeable to water** (200) | | | | | | | **Medulla** | | **Na (600)** | **H<sub>2</sub>O (400)** | **Na (700)** | **H<sub>2</sub>O (900)** | | **Water (3) moves into blood capillaries (900)** | | | | | | | **Descending limb with walls permeable to water** | | | **Sodium ions pumped into interstitial region** | | | | **Values in the loop of Henlé represent the concentration of the filtrate in water** (1100) | | | **Sodium ions move out passively by facilitated diffusion (1200)** | | | | **Water (5) moves into blood capillaries (900)** | | | **Increasing ion concentration** | | **Collecting duct with walls that vary in their permeability to water** | | **Concentrated (hypertonic) urine** (lower water potential) | | | | | | ### Counter-Current Multiplier of the Loop of Henlé: 1. **Sodium and chloride ions are actively pumped out** of the ascending limb of the loop of Henlé using ATP provided by the many mitochondria in the cells of its wall. 2. This creates **a low water potential (high ion concentration)** in the region of the medulla between the two limbs (called the interstitial region). 3. **The walls of the descending limb are, however, very permeable to water** and as the cells have many membrane protein channels known as aquaporins **it passes out of the filtrate, by osmosis, into the interstitial space**. 4. This water **enters the blood capillaries in this region by osmosis and is carried away**. 5. The filtrate progressively loses water in this way as it moves down the descending limb, lowering its water potential. It reaches its minimum water potential at the tip of the hairpin. 6. At the base of the ascending limb, sodium and chloride ions diffuse out of the filtrate and as it moves up the ascending limb these ions are also actively pumped out (see point 1) and **therefore the filtrate develops a progressively higher water potential**. 7. In the interstitial space between the ascending limb and the collecting duct, there is a gradient of water potential with the highest water potential in the cortex and an increasingly lower water potential the further into the medulla one goes. 8. The collecting duct **is permeable to water** and so, as the filtrate moves down it, **water passes out of it by osmosis**. 9. This water passes by osmosis into the blood vessels that occupy this space, and is carried away. 10. As water passes out of the filtrate its water potential is lowered. **However, the water potential** is also lowered in the interstitial space and so water continues to move out by osmosis **down the whole length of the collecting duct.** 11. **The counter-current multiplier** ensures that there is **always a water potential gradient drawing water out of the tubule**. 12. The water that passes out of the collecting duct by osmosis does so through aquaporins (water channels). The hormone ADH can alter the number of these channels and so control water loss. 13. By the time the filtrate, now called urine, leaves the collecting duct on its way to the bladder, **it has lost most of its water and so it has a lower water potential than the blood**. ## The Distal (Second) Convoluted Tubule - The cells that make up the walls of the distal (second) convoluted tubule have microvilli and many mitochondria that allow them to reabsorb material rapidly from the filtrate, **by either diffusion or active transport.** - The main role of the distal tubule **is to make final adjustments to the water and salts** that are reabsorbed and to **control the pH of the blood by selecting which ions to reabsorb**. - To achieve this, **the permeability of its walls becomes altered under the influence of various hormones**. - A summary of the processes taking place in the nephron is given in Figure 2. ## Counter-Current Multiplier - When two liquids flow in opposite directions past one another, the exchange of substances (or heat) between them is greater than if they flowed in the same direction next to each other. - In the case of the loop of Henlé, the counter-current flow means that **the filtrate in the collecting duct with a lower water potential meets interstitial fluid that has an even lower water potential.** - This means that, **although the water potential gradient between the collecting duct and interstitial fluid is small, it exists for the whole length of the collecting duct.** - There is **therefore a steady flow of water into the interstitial fluid, so that around 80% of the water enters the interstitial fluid and hence the blood**. - If the two flows were in the same direction (parallel), less of the water would enter the blood. ## Control of Water and Solute Concentration of the Blood - The quantity of water and salts we take in varies from day to day, as does the quantity we lose. - Table 1 shows the daily balance between loss and gain of salts and water for a typical human. - The blood, however, needs to have a constant volume of water and concentration of salts to avoid osmotic disruption to cells. - The homeostatic control of water and solute concentrations in the blood is achieved by hormones that act on the distal (second) convoluted tubule and the collecting duct. ### Table 1: Daily Water and Salt Balance in a Typical Human | | **Volume of water/cm<sup>3</sup> day<sup>-1</sup>** | | :-------------- | :---------------------------------------------------------- | | **Water gain** | | | **Diet** | 2300 | | **Metabolism, e.g. respiration** | 200 | | **TOTAL** | 2500 | | **Water loss** | | | **Urine** | 1500 | | **Expired air** | 400 | | **Evaporation from skin** | 350 | | **Faeces** | 150 | | **Sweat** | 100 | | **TOTAL** | 2500 | | | **Mass of salt/gday<sup>-1</sup>** | | :-------- | :------------------------------------ | | **Salt gain** | | | **Diet** | 10.50 | | **TOTAL** | 10.50 | | **Salt loss** | | | **Urine** | 10.00 | | **Faeces** | 0.25 | | **Sweat** | 0.25 | | **TOTAL** | 10.50 | - As a result **of facilitated diffusion and active transport by cells in the ascending limb of the loop of Henle,** **sodium** and **chloride ions are concentrated in the interstitial fluid surrounding the distal convoluted tubule and the collecting duct**. - There is a gradient of water potential within this interstitial region, with the highest water potential in the cortex and the lowest in the medulla region closest to the renal pelvis. - Some urea passes out of the filtrate in the collecting duct into the interstitial region, so further increasing the concentration of solutes and decreasing the water potential. - At all points, the interstitial region has a lower water potential than the filtrate passing down the collecting duct. ## The Naming of Antidiuretic Hormone - Diuresis is **the production of large volumes of dilute urine.** - It is a symptom of a disease called **diabetes insipidus**. - The disease was successfully treated with pituitary extract. - Therefore it was suggested that a hormone existed that was given the name 'antidiuretic hormone'. - **ADH increases the permeability of collecting ducts so that more water is reabsorbed into the blood.** - It causes the production of small volumes of concentrated urine. - This is the opposite of diuresis – hence the name antidiuretic hormone. ## Regulation of the Water Potential of the Blood - The water potential of the blood is determined **by the balance of water and salts within it.** - A rise in solute concentration lowers its water potential. - This may be caused by: - too little water being consumed - much sweating occurring - large amounts of salt being taken in (ingested). - **The body responds to this decrease in water potential as follows**: - Sensory cells called **osmoreceptors in the hypothalamus of the brain detect the decrease in water potential.** - It is thought that, when the water potential of the blood is low, water is lost from these osmoreceptor cells by osmosis. - Owing to this water loss, the osmoreceptor cells shrink. - This change stimulates the neurosecretory cells in the hypothalamus to produce a hormone called **antidiuretic hormone (ADH)**. - **ADH passes along the neurones (nerve cells) to the posterior pituitary gland, from where it is secreted into the capillaries.** - **ADH passes in the blood to the kidney, where it increases the permeability to water of the cell surface membrane of the cells that make up the walls of the distal (second) convoluted tubule and the collecting duct.** - **Receptors on the cell surface membrane of these cells bind to ADH molecules**, activating a second messenger system within the cell (cyclic AMP). - This results in the activation of a protein kinase, an enzyme that adds phosphate groups to other proteins to activate them. - The action of protein kinase causes vesicles within the cell to move to, and fuse with, its cell surface membrane. - ADH binding also leads to an increase in transcription of the gene coding for the aquaporin protein, increasing the number of available aquaporins. - When the vesicles fuse with the cell surface membrane, the number of aquaporins in the membrane increases greatly, making the cell surface membrane much more permeable to water. - As it is a small molecule, some urea can cross the phospholipid bilayer of the membrane. - ADH binding also leads to an increase in membrane transport proteins for urea, so that the collecting duct becomes more permeable to urea and some will leave the filtrate to further decreases the water potential of the interstitial region. - The combined effect is that more water leaves the collecting duct by osmosis down a water potential gradient and re-enters the blood. - As the reabsorbed water came from the blood in the first place, this will not, in itself, increase the water potential of the blood, but merely prevent it from decreasing any further. - Therefore the osmoreceptors also stimulate the thirst centre of the brain, to encourage the individual to seek out and drink more water. - The osmoreceptors in the hypothalamus detect the increase in water potential and ADH secretion from the posterior pituitary is reduced. - The decrease in ADH concentration in the blood will lead to a decreased permeability of the collecting duct to water and urea so that the permeability returns to its former state. - This is an example of homeostasis and the principle of negative feedback. ## Summary Test 14.6: - **Summary Test 14.6** 1. A human gains around **1 cm³ of water each day** 2. of which **2 cm³ comes from the diet**, with the remainder being produced in metabolic processes such as (3). 3. More than half this water is lost from the body as **(4)** 4. The same typical human needs around 10.5 g of salt in the diet, of which 10g is lost in the urine and 0.25 g in faeces. The remainder is lost in **(5)**. 5. Despite daily fluctuations in water and salt intake, the water potential of the blood remains relatively constant as a result of **(6)** control achieved by hormones that act on the **(7)** and collecting duct. 6. If too little water or too much salt is consumed, or if **(8)** is excessive, the water potential of the blood will **(9)**. 7. In response to this, osmoreceptors in the **(10)** of the brain detect the change and produce antidiuretic hormone (ADH) that passes to the **(11)** gland from where it is secreted. 8. ADH passes via the blood to the kidney where it increases the **(12)** of the distal convoluted tubule and collecting duct to water and **(13)** 9. As a result more water is reabsorbed and enters the blood. 10. The osmoreceptors also stimulate a thirst response and so more water is drunk and the water potential of the blood therefore **(14)**. 11. When the water potential returns to normal, the osmoreceptors detect this and ADH production is reduced to normal - an example of the principle of **(15)**.