Synthetic Biology Notes PDF
Document Details
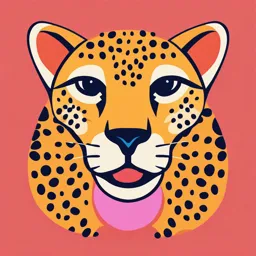
Uploaded by AffluentSanAntonio
Eduvos
Tags
Summary
This document provides notes on synthetic biology, including its introduction, applications in various fields, limitations, and future implications. It highlights the use of synthetic biology in medicine, agriculture, and manufacturing, particularly in diagnostic tools, crop domestication, and 3D bioprinting. It touches on the advantages and challenges of the technology, including its applications to conservation and ethical concerns.
Full Transcript
18/11/2024, 08:31 14.1. Notes | Eduvos 3. Introduction As human needs change, so can bioprocessing enable humanity to adapt to these needs. Techniques such as synthetic biology and bioprinting have already and will in future divers...
18/11/2024, 08:31 14.1. Notes | Eduvos 3. Introduction As human needs change, so can bioprocessing enable humanity to adapt to these needs. Techniques such as synthetic biology and bioprinting have already and will in future diversify the space for bioprocessing development in various sectors such as biopharmaceuticals, bioremediation and the food and beverage industry. 4. Synthetic Biology What is it? → The design and construction of novel, nucleic acid encoded biological parts, devices, systems machines and organisms, and their application for useful purposes. How is it different from genetic engineering? → Genetic engineering refers to the direct manipulation of an organism’s DNA which results in a desired phenotype, whilst synthetic biology looks at biological systems and applies engineering principles to create entire synthetic DNA molecules, parts of an organism or organisms itself. In short, traditional genetic engineering makes small alterations to genetic code, whilst synthetic biology gives a specific bioproduct a complete overhaul or creates a new one from scratch (hence https://mylms.vossie.net/mod/book/tool/print/index.php?id=776277 3/18 18/11/2024, 08:31 14.1. Notes | Eduvos the term synthetic). Used globally to solve problems in medicine, manufacturing and agriculture. Table 1: Comparison of typical characteristics of genetic engineering versus synthetic biology Table source: https://eduinput.com/difference-between-synthetic-biology-and-genetic-engineering/. Applications: Figure 1: Potential applications of synthetic biology in four sectors. Source: https://www.gao.gov/products/gao-23-106648. The following sub-sections will further explore the application of synthetic biology in these four industries, as well as its advantages and limitations. https://mylms.vossie.net/mod/book/tool/print/index.php?id=776277 4/18 18/11/2024, 08:31 14.1. Notes | Eduvos 4.1. Application in Biopharmaceuticals / Medicines Diagnostic tools Early detection of disease crucial for increasing patient survival and reducing burden on healthcare industry. Conventional diagnostic tools rely on antibody-based strategies which are costly to produce, and slow. General strategy: design RNA molecule → can bind to a sequence of pathogenic nucleic acid sequence, which then results in the generation of a detectable signal (e.g., fluorescence or colour change). Example: Toehold switches → rely on conformation change in the structure of this RNA device following binding to the target sequence (in this case a specific disease-causing antigen) which triggers translation of a downstream reporter gene (e.g., gfp gene à causes fluorescence which can be detected to identify whether antigen has binded). Here is a video link that describes what a toehold switch is → https://www.youtube.com/watch?v=qk5Gma-RLoI. Has been used in diagnostic tools for wide range of viral pathogens, including SARS-CoV-2 and other coronavirueses, West Nile Virus, Zika virus, and Ebola virus. Living therapeutics Use of RNA devices allows for engineering of living therapeutics, such as cell therapies. Have ability to sense and respond to environmental factors that provide information about their location, relevant disease states, and the therapeutic window timing. Such cell therapies include circulating cells (cells that circulate in the blood like white blood cells, red blood cells and cancer cells), implantable / transplantable cells, and tissue resident human cells (cells that reside in specific tissues). Example: using RNA devices to control engineered T cells (i.e., CAR (chimeric antigen receptor) T cells) to improve the safety profile and therapeutic outcomes of CAR-T therapy. CAR-T cells → form of immunotherapy where CARs engineered to recognize and eliminate cells expressing tumor antigens. Approach demonstrated profound clinical efficacy against hematological cancers (cancers that begin in blood forming tissues like bone marrow) → leukemia, lymphoma, myelomas. RNA vaccines Designed to introduce synthetic RNA encoding for pathogen-specific antigens (such as the SARS-CoV-2 spike protein) into cells to trigger an immune response against target pathogens. Compared to conventional vaccines (like virus-, viral-vector-, and protein-based) synthetic nature of RNA vaccines offer advantages: https://mylms.vossie.net/mod/book/tool/print/index.php?id=776277 5/18 18/11/2024, 08:31 14.1. Notes | Eduvos Speed of development. Scalability. Safety. Immunogenicity. What does this mean? → their synthetic, cell-free production method enable mRNA vaccines to be produced quickly and at a large scale making them particularly well-suited for addressing emerging infectious diseases, such as COVID-19. In fact, mRNA vaccines from Pfizer/BioNTech and Moderna were conceived of, designed, clinically tested, and granted Emergency Use Authorization in under a year. Not limited to COVID-19. Recent studies show potential to useful against variety of infectious diseases, such as influenza, dengue virus, herpes simplex virus type 2 (HSV-2), rabies, and Zika virus. Other efforts have tried to develop mRNA vaccines against bacterial diseases such as Mycobacterium tuberculosis (M.tb), one of the world’s leading infectious killers (especially also in South Africa). Using host synthetic machinery to produce bacterial proteins can lead to issues such as → Difficulties in folding, transport, and post-translational modifications. mRNA vaccines being investigated for potential use in cancer therapy. Initial studies show ability of mRNA vaccines to induce immune response against cancer cells → enabling body’s own defense system to recognize and attack. Promising results observed for breast cancer and prostate cancer. 4.2. Application in Agriculture Future agricultural development important for food security crisis caused by the global food shortage and the environmental demand for green and sustainable technology. Synthetic biology has brought new opportunities for modern agriculture: Crop domestication and breeding Traditional methods still laborious and lengthy process, often taking years which cannot always meet the demand of the current food supply chain. Synthetic biology can help when it comes to creating / writing novel plant genomes. Example: controlled, large, heritable chromosome rearrangements (megabases) has been successful in Arabidopsis thaliana using CRISPR-Cas9. In future, this application could be used for https://mylms.vossie.net/mod/book/tool/print/index.php?id=776277 6/18 18/11/2024, 08:31 14.1. Notes | Eduvos improved crop domestication and breeding. Photosynthesis and nitrogen fixation Photosynthesis → source of energy for crops and primary determinant of crop yield. Calvin cycle (part of photosynthesis): absorbed CO2 fixed to ribulose-1,5-bisphosphate (RuBP) by enzyme reaction. Enzyme responsible: RuBP carboxylase (Rubisco). Rubisco activity improved using synthetic biology techniques. Design of a synthetic / new carbon fixation pathway also been demonstrated by creating a synthetic chloroplast (in vitro). Improving biological nitrogen fixation pathway and increasing utilization rate of nitrogen sources by crops also important area for applying synthetic biology in agriculture. Gene cluster constructed synthetically based on the nif cluster found in nitrogen fixing bacteria, Klebsiella oxytoca, and inserted into plant. Improving these aspects can positively improve the impact of agricultural practices on the environment, whilst increasing crop yield. Microorganism utilization Biofertilizers → Currently, one-third of the land surface in the world is degraded to varying degrees, and 24 billion tons of fertile soil lost every year, posing significant threat to ecosystems and agricultural processes. Soil microorganisms can restore degraded lands, improving soil hydraulic properties, and reducing soil hydrophobicity. Regarding their impact on soil remediation → most valuable microbiotas can be divided into three categories: Plant growth promoting rhizobacterias (PGPRs). Nitrogen-fixing bacteria. Arbuscular mycorrhizal fungi (AMF) together with ectomycorrhizal fungi (EMF). These microorganisms also have broad application space in the field of soil remediation, such as: Stimulating plant growth. Preventing evaporation. Pollutant degradation. Disease elimination. https://mylms.vossie.net/mod/book/tool/print/index.php?id=776277 7/18 18/11/2024, 08:31 14.1. Notes | Eduvos Current synthetic biology studies demonstrated that engineered microbial consortia composed of multiple interacting microbial populations can perform complex tasks and withstand variable environmental influences. Some studies already constructed synthetic microbial communities can repair diseased soils so crops could resist the attack of plant pathogens through disease-suppressive soils. Synthetic microbial communities → provide solution for the use of microorganisms to remediate soil and improve the survival rate of microorganisms by reshaping the structure of soil microbial communities, which is also a direction of application in the future. Figure 2: Application of Synthetic Biology in agriculture. Source: Wang et al. 2022. DOI: https://doi.org/10.1016/j.aac.2022.08.005. 4.3. Application in Manufacturing Silk production → Spider silks → well known for extraordinary mechanical properties. This is because of the interplay of composition, structure and self-assembly of spider silk proteins (spidroins). https://mylms.vossie.net/mod/book/tool/print/index.php?id=776277 8/18 18/11/2024, 08:31 14.1. Notes | Eduvos Advances in synthetic biology → enabled the design and production of spidroins with the aim of biomimicking the structure-property-function relationships of spider silks in silkworms. How? → Using CRISPR-Cas9, genes responsible for spider silk inserted into silk worms, then the protein is purified either as a powder or a thread that can be spun. Can be used to make several products such as: Bullet-proof vests Ropes Nets Seatbelts Parachutes Bandages Surgical thread 4.4. Application in Conservation Due to the climate crisis, coral reefs are at risk. Increasing ocean temperatures are leading to coral bleaching. Repeated bleaching events can kill off a reef entirely. Synthetic biology been suggested to help can re-design DNA structures of the coral itself, making it more resilient to threats, such as increasing water temperatures. Proposed introduction of heat shock genes, which would provide such protection. This type of approach is still in the research phase but shows great promise for the future. Thus far, most of human genetic engineering has been in domesticated plants and animals. Interfering with wild populations holds significant risks, both practically and morally. 4.5. Advantages of Using Synthetic Biology https://mylms.vossie.net/mod/book/tool/print/index.php?id=776277 9/18 18/11/2024, 08:31 14.1. Notes | Eduvos Widely adaptable → Holds potential to help diagnose, and treat diseases, improve industrial processes, and address some environmental challenges. More equitable access to biotechnology → Some of the tools needed are low-cost and widely available, which could make access to beneficial applications more equitable. Conservation efforts → Could support endangered species conservation, for example, by altering the genes of endangered plants to make them resilient to diseases and climate change. 4.6. Limitations of Synthetic Biology Safety and security concerns: Could be threat to national security if used for nefarious purposes, such as developing new biological or chemical weapons. Computational tools used could be vulnerable to cyberthreats such as automation hacking. Environmental effects: Organisms made using synthetic biology, then released into the environment could have unknown, unintended, and potentially irreversible effects on ecosystems. Could be widespread if, for example, these organisms negatively affected food or water systems. Public acceptance and access: The public may hesitate to accept certain applications to concerns about interfering with nature and about unintended effects. Some medical applications could be inaccessible for some patients due to cost or location of treatment centres. 5. 3D Bioprinting What is it? → An additive manufacturing process that uses bio-inks to print living cells, developing structures layer-by-layer which imitate the behaviour and structures of natural tissues. Bio-inks → used as base material in bioprinting, made of natural or synthetic biomaterials, can be mixed with living cells. Can be cell-laden, scaffold-free, or cell-free. https://mylms.vossie.net/mod/book/tool/print/index.php?id=776277 10/18 18/11/2024, 08:31 14.1. Notes | Eduvos GrowInk™ made by UPD Biomedicals for example → customizable hydrogel-based bioink made of nanofibrillar cellulose and water. This technology and bioprinted structures enable researchers to study functions of the human body in vitro. 3D bioprinted structures are more biologically relevant compared to in vitro studies performed in 2D. Increasingly used for pharmaceutical development and drug validation, such as: 3D printed skin and bone grafts. Implants. Full 3D printed organs. Biomanufacturing of tissues/organs in vitro driven by two needs: Organ transplantation. Accurate tissue models. The following sub-sections will unpack the process, applications, advantages and disadvantages of 3D bioprinting. 5.1. Process of 3D Bioprinting The process of 3D bioprinting can be classified into four steps: 1. Data acquisition: 3D models obtained by using X-ray, computed tomography (CT), magnetic resonance imaging (MRI), etc. techniques to scan and reconstruct, or directly using computer-aided design (CAD) software to establish. Then divided into 2D horizontal slices (with customizable size and orientation) by specific software. These data are processed into particles or filaments according to different bioprinting approaches. 2. Material selection: Materials (including cells, growth factors, hydrogels, etc.) chosen according to the requirement of printed structures and approaches. The combination of these biomaterials is called bio-inks, while they could also be simply regarded as cell-laden hydrogels in most cases. Selection of bio-inks crucial to guarantee biocompatibility, printability and mechanical property. https://mylms.vossie.net/mod/book/tool/print/index.php?id=776277 11/18 18/11/2024, 08:31 14.1. Notes | Eduvos 3. Bioprinting: Before bioprinting, appropriate configuration of printing parameters. Observation during printing process is necessary to make adjustment when encounters any problems. 4. Functionalization: After printing, target is making dispersed cells that are forming connections and generating some functions of natural tissue/organ through physical and chemical stimulation. Figure 3: Four steps of 3D bioprinting. Source: Gu et al. (2020). DOI: https://doi.org/10.1016/j.ajps.2019.11.003. Extrusion-based bioprinting: Also called direct ink writing. Derived from inkjet printing. Most widely used approach of 3D bioprinting because of its versatility and affordability. Instead of single droplet, extrusion-based bioprinting produces ongoing filaments through continuous extrusion force. Can be used for printing wide range of viscosities of biomaterials and different concentrations of cells. For this reason, researchers prefer extrusion-based bioprinting to build tissue structures with sufficient mechanical property. How does it work? Theoretically, it extrudes bio-ink (usually from a syringe) through a nozzle by means of mechanical or pneumatic (via gas pressure) driven. https://mylms.vossie.net/mod/book/tool/print/index.php?id=776277 12/18 18/11/2024, 08:31 14.1. Notes | Eduvos Forms continuous micro filaments onto specific structure and are subsequently stacked into desired structures. Substrate can be solid (e.g., culture dish), liquid (e.g., growth medium) or material derived from gel. Path of nozzle usually generated by software according to digital models after configuration. Parameters such as temperature, nozzle diameter, extrusion pressure, movement speed, extrusion speed, path interval, etc. influence final bioprinted structures. According to the different actuating modes of liquid dispensing system, common extrusion-based bioprinting can be classified into pneumatic, piston and screw-driven. 5.2. Applications Can be divided into four categories: Cytobiology → fabricating single cell or multicellular combination, including research on basic issues about cell growth, intercellular relationship, transgenosis. Drug research → refers to pharmacokinetics, drug screening, ancillary drug development Tumor model → mainly about setting up various kinds of tumour pathological models, researching tumorigenesis mechanisms, targeted therapy, etc. Regenerative medicine → The main use of bioprinting, includes manufacturing of artificial tissue and organ, fabrication of neural tissue, cardioid and liver, vascularization of scale-up tissue and cell therapy. Applications based on cell-laden extrusion-based bioprinting: Skin Previous studies were successful by printing 10 layers of collagen hydrogel precursor in a layer-by- layer fashion. Fibroblasts was printed in the second layer and keratinocytes was printed in the eighth layer separately → to give a multi-layered engineered tissue composite to mimic natural skin layers. A different study isolated two major skin cell types found in the skin’s outer (epidermis) and middle (dermis) layers from a small biopsy of healthy skin Then expanded number of cells in the lab and delivered the cells directly into a skin injury (such as burn wound) using a specialised bioprinter. Using integrated imaging technology to scan a wound, computer software “prints” cells right into an injury, mimicking two of our skin’s three natural layers. https://mylms.vossie.net/mod/book/tool/print/index.php?id=776277 13/18 18/11/2024, 08:31 14.1. Notes | Eduvos Figure 4: Artificial skin can be printed by layering specific cell mixtures to mimic human skin: epidermis (top), dermis (middle) and hypodermis (bottom). Source: https://directorsblog.nih.gov/2023/10/17/can-bioprinted- skin-substitutes-replace-traditional-grafts-for-treating-burn-injuries-and-other-serious-skin-wounds/. Bone/Cartilage Previous studies found success using bio-ink consisting of gellan, alginate and a clinical product called BioCartilage (cartilage extracellular matrix particles), to bioprint cartilage grafts. This bio-ink proven to support proliferation of chondrocytes and strong deposition of cartilage matrix proteins (in the presence of transforming growth factor beta-3) through MRI and histological evaluation after 8 weeks in vitro. Another study → Figure 5: Steps in bioprinting a bone scaffold for repair in vivo: Pre-processing: prepare a mixture using hydrogel, stem cells, and growth factors. Bioprinting: successful 3D bioprinting of biomaterials with physiological cell density in a designed way. Post-processing: crosslinking of bioprinted constructs by UV-ray. Figure 5: Schematic illustration of 3D bioprinting of hydrogels scaffold for repair of bone and cartilage defect. Source: Yang et al. (2022). DOI: https://doi.org/10.3389/fbioe.2022.865770. https://mylms.vossie.net/mod/book/tool/print/index.php?id=776277 14/18 18/11/2024, 08:31 14.1. Notes | Eduvos Nerves Scientists have pioneered 3D printing of nerve networks using bio-inks packed with living neurons. Technique allows for creation of 3D neural circuits that emulate the intricate connections seen in a living brain. These bioprinted networks, bridging gray and white matter, exhibit spontaneous nerve activity and respond to stimuli. How? → Utilized two distinct bio-inks: one teeming with living cells and the other without, replicating the brain’s gray and white matter arrangement. Resulting 3D neural structures exhibited genuine connections, with neurites forming links between different cortex layers—much like a living brain. The 3D bioprinted nerve networks displayed spontaneous electrical activity, marking a major stride in neuroscience and bioprinting realms. Muscle/Tendons Study has used 3D bioprinter with four cartridges for deposition of four different components to fabricate an integrated muscle–tendon unit (MTU) construct. Thermoplastic polyurethane (PU) was co-printed with a cell-laden hydrogel-based bi-ink for elasticity and muscle development on one side, while PCL was co-printed with a different cell-laden hydrogel- based bio-ink for stiffness and tendon development on the other. https://mylms.vossie.net/mod/book/tool/print/index.php?id=776277 15/18 18/11/2024, 08:31 14.1. Notes | Eduvos Figure 6: The new approach involves incorporating microparticles loaded with insulin-like growth factor (IGF) into a bio-ink containing myoblast cells and a gelatin-based hydrogel. Source: https://3dprinting.com/news/bioprinted-muscles-can-contract-after-just-10-days-of-growth/. Adipose tissue Previous studies employed decellularized adipose tissue (DAT) matrix encapsulating human adipose tissue-derived mesenchymal stem cells (hASCs) as bio-ink to print flexible dome-shaped structures with engineered porosity within a PCL framework. Implantation experiment showed that the structure did not induce chronic inflammation or cytotoxicity post-implantation, but supported positive tissue infiltration, constructive tissue remodeling, and adipose tissue formation. Figure 7: Illustration of reconstructing the female breast with a personalized three-dimensional bioprinted adipose tissue construct using stem cells derived from autologous tissue. Source: https://www.sciencedirect.com/science/article/pii/S0264127521000204. Blood vessel/vascularization Human umbilical vein smooth muscle cells (HUVSMCs), human skin fibroblasts (HSFs) and porcine aortic smooth muscle cells (PASMCs) have been used to build scaffold-free small-diameter multi- layered tubular vascular grafts. Cells were aggregated into discrete units, then printed layer-by-layer with agarose rods, used as a moulding template. Post-printing fusion of discrete units resulted in single- and double-layered small diameter vascular tubes while agarose was removed. https://mylms.vossie.net/mod/book/tool/print/index.php?id=776277 16/18 18/11/2024, 08:31 14.1. Notes | Eduvos Figure 8: 3D printed blood vessel. Source: https://www.esa.int/ESA_Multimedia/Images/2024/04/3D- bioprinted_blood_vessel. 5.3. Advantages and Disadvantages of 3D Bioprinting Advantages of 3D bioprinting: Allows the mimicking of real structures of desired tissue/organ etc. addressing the organ shortage simultaneously. Possibility to revolutionize future medical treatment capabilities. Possible creation of patient-specific and organ-specific treatments. Effects of drugs can be examined more accurately. Decreased animal testing. Biocompatibility with human cells and tissues. Automating complex processes. Consistency, less human errors. Disadvantages of 3D bioprinting: Pricing, expensive technology, therefore also not available to everyone. Complexity → more room for errors. Maintaining cell environment can be difficult, contamination sensitive. Ethical concerns → “playing God” by creating human organs in labs. Energy consumption https://mylms.vossie.net/mod/book/tool/print/index.php?id=776277 17/18 18/11/2024, 08:31 14.1. Notes | Eduvos https://mylms.vossie.net/mod/book/tool/print/index.php?id=776277 18/18