Alkyl Halides: Reactions and Synthesis PDF
Document Details
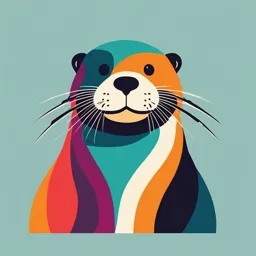
Uploaded by DignifiedSard558
Pondicherry University
Tags
Summary
This document provides a detailed overview of the preparation and reactions of alkyl halides. Topics covered include various methods for synthesizing alkyl halides from alkenes and alcohols, along with their reactions leading to the formation of nitriles, isonitriles, and ethers. The different mechanisms, such as S_N1 and S_N2, are explained with examples. This document is suitable for organic chemistry students and researchers.
Full Transcript
AI Chat 12/02/2024 11:45 AM gives information on question given You 12/03/2024 06:12 PM Alkyl halides:Preparation from alkenes and alcohol AI Chat 12/03/2024 06:13 PM Alkyl halides, also known as haloalkanes, are organic compounds that contain a halogen atom (such as fluorine, chlorine, bromine, or...
AI Chat 12/02/2024 11:45 AM gives information on question given You 12/03/2024 06:12 PM Alkyl halides:Preparation from alkenes and alcohol AI Chat 12/03/2024 06:13 PM Alkyl halides, also known as haloalkanes, are organic compounds that contain a halogen atom (such as fluorine, chlorine, bromine, or iodine) attached to an alkane carbon chain. They can be prepared from alkenes and alcohols through various methods. Below is a detailed explanation of the preparation of alkyl halides from both alkenes and alcohols. ### 1. Preparation of Alkyl Halides from Alkenes: Alkenes can be converted into alkyl halides through several methods, mainly involving electrophilic addition reactions. #### a. **Hydrogen Halide Addition:** Alkenes react with hydrogen halides (HX) to form alkyl halides. This reaction is governed by Markovnikov’s rule, which states that the more substituted carbon of the double bond will receive the halide. **Example:** - Propene + HBr → 2-Bromopropane #### b. **Halogen Addition:** Alkenes can react with dihalogens (X₂), such as Br₂ or Cl₂, to form vicinal dihalides (compounds with two halogen atoms on adjacent carbons). These can sometimes lead to the formation of alkyl halides through further reactions. **Example:** - Propene + Br₂ → 1,2-Dibromopropane (which can be converted to an alkyl halide through further reactions) #### c. **Hydrohalogenation:** In the presence of an alcohol solvent, alkenes can react with hydrogen halides to give alkyl halides. The alcohol acts as a solvent and can stabilize the carbocation intermediate. ### 2. Preparation of Alkyl Halides from Alcohols: Alcohols can be converted into alkyl halides through halogenation reactions. The process generally involves the conversion of the hydroxyl (-OH) group into a halogen atom. #### a. **Conversion to Hydrohalic Acids:** Alcohols react with hydrogen halides (HX) to replace the -OH group with a halogen atom. **Example:** - Ethanol + HCl → Ethyl chloride + H₂O #### b. **Thionyl Chloride (SOCl₂):** Alcohols can be treated with thionyl chloride to produce alkyl chlorides. This method is commonly used for converting alcohols to alkyl chlorides efficiently. **Example:** - Ethanol + SOCl₂ → Ethyl chloride + SO₂ + HCl #### c. **Phosphorus Halides:** Alcohols can also be converted using phosphorus halides (PBr₃, PCl₃, or PI₃), which reacts with alcohols to form alkyl halides. **Example:** - Ethanol + PBr₃ → Ethyl bromide + H₃PO₃ #### d. **Swarts Reaction:** Alcohols can be treated with silver halides and heat to form alkyl halides. This method is especially useful for converting alcohols to more reactive alkyl halides. ### Summary: - **From Alkenes:** Alkyl halides are primarily prepared via electrophilic addition (e.g., hydration with HX or halogenation) and follow Markovnikov's rule. - **From Alcohols:** Conversion typically involves methods such as treatment with hydrogen halides, thionyl chloride, phosphorus halides, or through alternative halogenation methods. These processes not only showcase the versatility of alkenes and alcohols in synthesizing alkyl halides but also highlight the influence of reaction conditions and reagents employed. You 12/03/2024 06:13 PM Alkyl halides : Reactions - hydrolysis, nitrite & nitro formation AI Chat 12/03/2024 06:13 PM Alkyl halides, or haloalkanes, participate in various chemical reactions due to the presence of the halogen atom, which can be replaced or modified under certain conditions. Here, we will discuss three significant reactions of alkyl halides: hydrolysis, formation of nitrites, and formation of nitro compounds. ### 1. Hydrolysis of Alkyl Halides: Hydrolysis of alkyl halides involves the reaction of the alkyl halide with water (or a hydroxide), resulting in the formation of an alcohol. This reaction can occur through two primary mechanisms: nucleophilic substitution (S_N1 and S_N2). #### a. **S_N1 Mechanism:** - This mechanism generally occurs with tertiary alkyl halides where the halide ion leaves first, forming a carbocation intermediate. - Water then acts as a nucleophile, attacking the carbocation to form the alcohol. **Example:** - (CH₃)₃CBr + H₂O → (CH₃)₃COH + HBr #### b. **S_N2 Mechanism:** - This mechanism usually occurs with primary or secondary alkyl halides where the nucleophile attacks the carbon simultaneously as the leaving group (the halide) departs. - Here, a single transition state is formed without any intermediates. **Example:** - CH₃Br + OH⁻ → CH₃OH + Br⁻ ### 2. Formation of Nitrites from Alkyl Halides: Alkyl halides can react with sodium nitrite (NaNO₂) to form nitrites. This reaction typically occurs under alkaline conditions and uses a nucleophilic substitution mechanism. #### - **S_N2 Reaction:** In this reaction, the nitrite ion (NO₂⁻) acts as a nucleophile and attacks the alkyl halide, leading to the displacement of the halide ion. **Example:** - CH₃Br + NaNO₂ → CH₃NO₂ (ethyl nitrite) + NaBr The formed nitrites can be further processed for creating various nitrogen-containing compounds. ### 3. Formation of Nitro Compounds from Alkyl Halides: Alkyl halides can be converted into nitro compounds through a nucleophilic aromatic substitution (for aromatic alkyl halides) or through a more direct reaction with nitrogenous compounds. #### a. **Nitration Reaction:** - Alkyl halides, particularly benzyl halides, can be subjected to nitrating conditions (using a mixture of concentrated nitric and sulfuric acids), leading to the formation of nitro compounds. **Example:** - C₆H₅CH₂Cl + HNO₃ → C₆H₅CH₂NO₂ + HCl #### b. **Bromination and Subsequent Nitration:** - An alkyl halide can first react with bromine to create a brominated compound, which upon treatment with HNO₃ results in the formation of the nitro compound. ### Summary: - **Hydrolysis:** Alkyl halides undergo hydrolysis to yield alcohols through S_N1 or S_N2 mechanisms, depending on the structure of the alkyl halide. - **Formation of Nitrites:** Alkyl halides react with nitrite ions in nucleophilic substitution reactions to form nitrites. - **Formation of Nitro Compounds:** Through the process of nitration, alkyl halides can be transformed into nitro compounds, contributing to the formation of numerous important organic compounds. Understanding these reactions is critical for applications in organic synthesis, pharmaceuticals, and materials science. You 12/03/2024 06:14 PM Alkyl halides : nitrile and isonitrile formation AI Chat 12/03/2024 06:14 PM Alkyl halides can be converted into nitriles and isonitriles through various chemical reactions. Below are detailed mechanisms and examples for the formation of both types of compounds. ### 1. Formation of Nitriles from Alkyl Halides: Nitriles (R-C≡N) are functional groups that contain a cyano group. Alkyl halides can be transformed into nitriles primarily through nucleophilic substitution reactions. One common method involves the reaction of alkyl halides with sodium cyanide (NaCN). #### a. **Nucleophilic Substitution Reaction:** In this reaction, sodium cyanide acts as a nucleophile and displaces the halide ion in an S_N2 reaction. This process is especially effective for primary alkyl halides, as they readily undergo S_N2 mechanisms. **General Reaction:** \[ \text{R-X} + \text{NaCN} \rightarrow \text{R-C}\equiv\text{N} + \text{NaX} \] **Example:** - **Bromoethane → Ethanenitrile** \[ \text{C}_2\text{H}_5\text{Br} + \text{NaCN} \rightarrow \text{C}_2\text{H} _5\text{C}\equiv\text{N} + \text{NaBr} \] (Bromoethane + Sodium cyanide → Ethanenitrile + Sodium bromide) ### 2. Formation of Isonitriles from Alkyl Halides: Isonitriles (R-N≡C) are also called carbamates and have a functional group that contains a terminal isocyanide group. The formation of isonitriles from alkyl halides is less common but can be achieved using specific reagents like silver cyanide (AgCN) or by reaction with certain isocyanide-generating agents. #### a. **Using Silver Cyanide (AgCN):** When alkyl halides react with silver cyanide, they can convert into isonitriles via a similar nucleophilic substitution mechanism. In this case, AgCN is similar to NaCN, but with different mechanistic pathways leading to isonitriles. **General Reaction:** \[ \text{R-X} + \text{AgCN} \rightarrow \text{R-N}\equiv\text{C} + \text{AgX} \] **Example:** - **Bromoethane → Ethyl isocyanide** \[ \text{C}_2\text{H}_5\text{Br} + \text{AgCN} \rightarrow \text{C}_2\text{H} _5\text{N}\equiv\text{C} + \text{AgBr} \] (Bromoethane + Silver cyanide → Ethyl isocyanide + Silver bromide) #### b. **Alternative Methods:** Another approach to form isonitriles includes using a combination of primary amines with chloroform (CHCl₃) or other chloroalkanes under basic conditions. This method entails generating an isocyanide intermediate that reacts with an alkyl halide. ### Summary: - **Nitrile Formation:** - Nitriles are typically synthesized from alkyl halides through nucleophilic substitution with sodium cyanide, leading to the formation of a cyano group attached to the carbon. - **Isonitrile Formation:** - Isonitriles can be prepared from alkyl halides using silver cyanide or other specific reagents, resulting in a nitrogen atom triple-bonded to the carbon. These reactions are valuable in organic synthesis for generating compounds used in pharmaceuticals, agrochemicals, and various industrial applications. Each pathway reflects the versatility and reactivity of alkyl halides in forming new functional groups. You 12/03/2024 06:14 PM Alkyl halides : Williamson’s synthesis AI Chat 12/03/2024 06:14 PM **Williamson's Synthesis** is a well-known method for the preparation of ethers from alkyl halides and is a significant reaction in organic chemistry. It is named after the chemist Alexander Williamson, who described it in the mid-19th century. This reaction primarily involves the nucleophilic substitution of an alkyl halide with an alkoxide ion to generate an ether. ### Overview of Williamson's Synthesis #### General Reaction The Williamson synthesis can be represented by the following general reaction scheme: \[ \text{R-X} + \text{R'-O}^- \rightarrow \text{R-O-R'} + \text{X}^- \] Where: - R-X is the alkyl halide (where X = Br, Cl, or I) - R'-O^- is the alkoxide ion, which is generated from an alcohol (R'-OH) by deprotonation (usually using a base). - R-O-R' is the resultant ether. - X⁻ is the leaving group (halide ion). ### Mechanism of Williamson's Synthesis The Williamson synthesis typically proceeds through an **S_N2 mechanism**, especially when using primary or some secondary alkyl halides. 1. **Formation of Alkoxide Ion:** The alcohol (R'-OH) is treated with a strong base (like sodium hydride (NaH) or potassium tert-butoxide (KOt-Bu)) to generate the alkoxide ion (R'-O⁻). **Example:** \[ \text{R'-OH} + \text{NaH} \rightarrow \text{R'-O}^- \text{Na}^+ + \text{H} _2 \] 2. **Nucleophilic Attack:** The alkoxide ion then acts as a nucleophile and attacks the electrophilic carbon of the alkyl halide in an S_N2 fashion, resulting in the substitution of the halide ion and the formation of the ether. **Example:** \[ \text{R-X} + \text{R'-O}^- \rightarrow \text{R-O-R'} + \text{X}^- \] ### Requirements and Considerations 1. **Structure of Alkyl Halide:** - **Primary Alkyl Halides:** Best for Williamson synthesis due to minimization of steric hindrance. They allow for straightforward S_N2 reactions. - **Secondary Alkyl Halides:** Can be used but may lead to elimination reactions if sterically hindered. - **Tertiary Alkyl Halides:** Generally not suitable for Williamson synthesis since they favor elimination (E2) reactions over substitution. 2. **Base Selection:** A strong base is used to effectively convert the alcohol into the alkoxide. Common bases include: - Sodium hydride (NaH) - Sodium metal (Na) - Potassium tert-butoxide (KOt-Bu) - Other alkali metal alkoxides 3. **Solvent:** The reaction is often conducted in polar aprotic solvents (like dimethyl sulfoxide (DMSO) or acetone) to facilitate the nucleophilic reaction. ### Example of Williamson's Synthesis **Preparation of Ethyl Methyl Ether:** 1. **Reactants:** - Alkyl halide: Bromomethane (CH₃Br) - Alkoxide: Sodium ethoxide (NaOEt) 2. **Reaction:** \[ \text{CH}_3\text{Br} + \text{NaOEt} \rightarrow \text{CH}_3\text{OEt} + \text{NaBr} \] (Where CH₃OEt is ethyl methyl ether) ### Advantages - **Versatility:** Williamson synthesis allows for the creation of a wide range of ethers using different alcohols and alkyl halides. - **Selective:** Enables the formation of asymmetric ethers by choosing appropriate alkyl halide and alcohol. ### Limitations - **Stereochemistry:** The reaction may lead to stereochemical inversion at the carbon center (due to the S_N2 mechanism). - **Competing Reactions:** With more hindered substrates, elimination reactions may compete with nucleophilic substitution. - **Availability of Alkyl Halide:** Synthesis of certain alkyl halides may be limited. ### Conclusion Williamson's synthesis is a powerful and widely used method for ether formation in organic chemistry, allowing for the preparation of a diverse array of ethers through the nucleophilic substitution mechanism. It showcases the interplay between nucleophilicity and electrophilicity while highlighting the importance of reaction conditions and mechanism considerations. You 12/03/2024 06:14 PM Alkyl halides : Elimination vs Substitution AI Chat 12/03/2024 06:15 PM In organic chemistry, alkyl halides can undergo two major types of reactions: **elimination** and **substitution**. Both types of reactions involve breaking bonds and forming new ones, but they differ significantly in their mechanisms, products, and reaction conditions. Below is a detailed comparison of elimination and substitution reactions involving alkyl halides. ### 1. Substitution Reactions Substitution reactions involve the replacement of one atom or group in a molecule with another. In the case of alkyl halides, the halogen atom (X) is typically replaced by a nucleophile. #### Mechanisms of Substitution: There are two main mechanisms for substitution reactions involving alkyl halides: **a. S_N1 Mechanism:** - **Definition:** Unimolecular nucleophilic substitution, where the rate-determining step involves the formation of a carbocation intermediate. - **Characteristics:** - Two-step process: formation of a carbocation followed by nucleophilic attack. - Commonly occurs with tertiary and some secondary alkyl halides due to greater stability of the carbocation. - Rate depends only on the concentration of the alkyl halide. - **Example:** \[ \text{(CH}_3\text{)}_3\text{CBr} \rightarrow \text{(CH}_3\text{)}_3\text{C} ^+ + \text{Br}^- \rightarrow \text{(CH}_3\text{)}_3\text{COH} \] **b. S_N2 Mechanism:** - **Definition:** Bimolecular nucleophilic substitution, where the nucleophile attacks the substrate at the same time as the leaving group departs. - **Characteristics:** - One-step process: simultaneous bond formation and breaking. - Preferred for primary and some secondary alkyl halides due to steric factors. - Rate depends on the concentrations of both the alkyl halide and the nucleophile. - Involves an inversion of configuration if the alkyl halide is chiral. - **Example:** \[ \text{CH}_3\text{Br} + \text{OH}^- \rightarrow \text{CH}_3\text{OH} + \text{Br}^- \] ### Products of Substitution: - The product of a substitution reaction involving an alkyl halide is typically an alcohol, amine, or other functional group, depending on the nucleophile used. --- ### 2. Elimination Reactions Elimination reactions involve the removal of a small molecule (often HX) from the substrate, resulting in the formation of a double bond (alkene) or triple bond (alkyne). #### Mechanisms of Elimination: The two primary mechanisms for elimination reactions are: **a. E1 Mechanism:** - **Definition:** Unimolecular elimination, similar to S_N1, where a carbocation is formed first before the elimination occurs. - **Characteristics:** - Two-step process: formation of carbocation followed by loss of a proton to form a double bond. - Occurs mainly with tertiary and some secondary alkyl halides. - Rate depends only on the concentration of the alkyl halide. - **Example:** \[ \text{(CH}_3\text{)}_3\text{CBr} \rightarrow \text{(CH}_3\text{)}_3\text{C} ^+ \rightarrow \text{(CH}_3\text{)}_2\text{C}=\text{C} + \text{HBr} \] **b. E2 Mechanism:** - **Definition:** Bimolecular elimination, where the base removes a hydrogen atom at the same time as the leaving group departs. - **Characteristics:** - One-step process: simultaneous removal of H and X. - Works best with strong bases and typically requires a dehydrohalogenation condition. - Rate depends on the concentrations of both the alkyl halide and the base. - **Example:** \[ \text{CH}_3\text{CH}_2\text{Br} + \text{OH}^- \rightarrow \text{CH} =\text{CH}_2 + \text{Br}^- + \text{H}_2\text{O} \] ### Products of Elimination: - The products of elimination reactions are alkenes or alkynes, depending on the extent of elimination (double or triple bond formation). --- ### Comparison of Substitution and Elimination | Feature | Substitution (S_N1/S_N2) | Elimination (E1/E2) | |----------------------|--------------------------------------|------------------------------------| | **Reaction Type** | Replacement of a group | Removal of groups (forming dbl/trpl bond) | | **Mechanism** | S_N1 or S_N2 | E1 or E2 | | **Intermediates** | S_N1: Carbocation / S_N2: Transition state | E1: Carbocation / E2: None | | **Factors Affecting Rate** | Concentration of alkyl halide and nucleophile (S_N2) | Concentration of alkyl halide and base (E2) | | **Preferred Substrates** | Primary (S_N2), Secondary, Tertiary (S_N1) | Tertiary (E1), Secondary (E2) | | **Product Type** | Alcohols, Amines, Ethers | Alkenes, Alkynes | | **Stereochemistry** | Inversion of configuration (S_N2), racemization (S_N1) | No preference, but E2 can lead to anti-coplanar elimination | | **Conditions** | Generally milder conditions | Requires strong bases, high temperature for E1/E2 | ### Conclusion Both substitution and elimination are fundamental reactions involving alkyl halides, with distinct mechanisms, products, and conditions. The choice between these reactions largely depends on the structure of the alkyl halide, the nature of the nucleophile or base, and the reaction conditions. Understanding these pathways helps in the design and optimization of synthetic routes in organic chemistry. You 12/03/2024 06:15 PM Aryl halides:Preparation of chloro-, bromo- and iodo-benzenes from pheno AI Chat 12/03/2024 06:15 PM Aryl halides, particularly chloro-, bromo-, and iodo-benzenes, can be derived from phenol through various halogenation reactions. Below is a detailed description of the methods for preparing these aryl halides from phenol. ### 1. Preparation of Chloro-, Bromo-, and Iodo-benzenes from Phenol: #### a. **Preparation of Chloro-benzene:** Chloro-benzene can be prepared from phenol through electrophilic aromatic substitution involving chlorination. This reaction typically uses a chlorinating agent such as phosphorus trichloride (PCl₃) or thionyl chloride (SOCl₂). **Using Phosphorus Trichloride (PCl₃):** **Reaction:** \[ \text{C}_6\text{H}_5\text{OH} + \text{PCl}_3 \rightarrow \text{C}_6\text{H} _5\text{Cl} + \text{HCl} + \text{POCl}_3 \] - **Steps:** 1. Phenol reacts with PCl₃, resulting in the formation of chloro-benzene and byproducts such as HCl and POCl₃. **Using Thionyl Chloride (SOCl₂):** **Reaction:** \[ \text{C}_6\text{H}_5\text{OH} + \text{SOCl}_2 \rightarrow \text{C} _6\text{H}_5\text{Cl} + \text{SO}_2 + \text{HCl} \] - **Steps:** 1. Phenol reacts with thionyl chloride to yield chloro-benzene, with sulfur dioxide and hydrochloric acid as byproducts. #### b. **Preparation of Bromo-benzene:** Bromo-benzene can be prepared by bromination of phenol. The use of bromine in the presence of a solvent (like water or acetic acid) can yield bromo-benzene. **Reaction:** \[ \text{C}_6\text{H}_5\text{OH} + \text{Br}_2 \rightarrow \text{C}_6\text{H} _5\text{Br} + \text{HBr} \] - **Steps:** 1. Phenol reacts with bromine, typically in an aqueous solution or a polar solvent, to produce bromo-benzene and hydrobromic acid. 2. The process involves electrophilic aromatic bromination, where the phenolic oxygen enhances the electron density of the aromatic ring, facilitating substitution. **Example:** - It is common to see bromo-benzene synthesized by simply adding liquid bromine to an ice-cold suspension of phenol. #### c. **Preparation of Iodo-benzene:** Iodo-benzene can be prepared through iodination of phenol. A common method involves using iodine in the presence of a suitable oxidizing agent such as iodine monochloride (ICl), or through direct reaction using aqueous iodine. **Using Iodine Monochloride (ICl):** **Reaction:** \[ \text{C}_6\text{H}_5\text{OH} + \text{ICl} \rightarrow \text{C}_6\text{H} _5\text{I} + \text{HCl} \] - **Steps:** 1. Phenol reacts with iodine monochloride, resulting in the formation of iodo-benzene and hydrochloric acid. **Using Aqueous Iodine:** - When phenol is treated with an aqueous solution of iodine in the presence of a base, this can lead to the formation of iodo-benzene. ### Summary of Reaction Pathways - **From phenol to chloro-benzene:** - Uses PCl₃ or SOCl₂. - **From phenol to bromo-benzene:** - Utilizes bromine (Br₂) under controlled conditions. - **From phenol to iodo-benzene:** - Can involve reaction with ICl or aqueous iodine. ### Conclusion The conversion of phenol to chloro-, bromo-, and iodo-benzenes demonstrates the versatility of phenol in organic synthesis. Each method utilizes electrophilic aromatic substitution, with the substitutive abilities of halogenated reagents taking advantage of the activated aromatic system of phenol. These reactions are fundamental in organic chemistry, contributing to the synthesis of various intermediates and fine chemicals. You 12/03/2024 06:15 PM Aryl halides: Sandmeyer and Gattermann reactions. AI Chat 12/03/2024 06:15 PM **Aryl halides** are aromatic compounds containing a halogen atom (such as fluorine, chlorine, bromine, or iodine) attached to an aromatic ring. They play a significant role in organic synthesis, and two important reactions involving aryl halides are the **Sandmeyer reaction** and the **Gattermann reaction**. These reactions are primarily used to introduce various substituents onto an aromatic ring. ### 1. Sandmeyer Reaction The **Sandmeyer reaction** is a method for transforming aryl amines into aryl halides with the use of copper(I) halides (CuX). It involves the reaction of an aryl diazonium salt with a copper(I) halide. #### Mechanism: 1. **Formation of Aryl Diazonium Salt**: - An aromatic amine (e.g., aniline) is treated with nitrous acid (HNO₂), typically generated in situ, to form a diazonium salt. \[ \text{C}_6\text{H}_5\text{NH}_2 + \text{HNO}_2 \rightarrow \text{C} _6\text{H}_5\text{N}_2^+ \text{X}^- + \text{H}_2\text{O} \] (Where X is generally a chloride ion.) 2. **Reactions with Copper(I) Halide**: - The diazonium salt can then react with copper(I) halides (like copper(I) bromide or copper(I) chloride) to produce the corresponding aryl halide. \[ \text{C}_6\text{H}_5\text{N}_2^+ + \text{CuX} \rightarrow \text{C} _6\text{H}_5\text{X} + \text{N}_2 + \text{Cu}^{2+} \] #### Example: - **Chlorination of Aniline**: - Aniline reacts with nitrous acid to form the diazonium salt, which then reacts with CuCl to yield chlorobenzene. \[ \text{C}_6\text{H}_5\text{NH}_2 \xrightarrow{\text{HNO}_2} \text{C} _6\text{H}_5\text{N}_2^+ \text{Cl}^- \xrightarrow{\text{CuCl}} \text{C} _6\text{H}_5\text{Cl} + \text{N}_2 \] ### 2. Gattermann Reaction The **Gattermann reaction** is used to introduce a formyl group (-CHO) into the aromatic ring via the reaction of an aryl halide with carbon monoxide in the presence of an acid catalyst, usually hydrogen chloride (HCl) or zinc chloride (ZnCl₂). #### Mechanism: 1. **Formation of Aryl Halide**: - In this reaction, the aryl halide (such as chlorobenzene or bromobenzene) reacts with a mixture of carbon monoxide and hydrogen chloride or another Lewis acid catalyst to form an aryl aldehyde. 2. **Catalytic Support**: - The reaction mechanism involves coordination of the Lewis acid to the halide, activation of carbon monoxide, and electrophilic substitution to yield the aryl aldehyde. **General Reaction**: \[ \text{Ar-X} + \text{CO} + \text{HCl} \rightarrow \text{Ar-CHO} + \text{HX} \] #### Example: - **Formylation of Chlorobenzene**: - Chlorobenzene reacts with carbon monoxide and HCl in the presence of zinc chloride to produce benzaldehyde. \[ \text{C}_6\text{H}_5\text{Cl} + \text{CO} + \text{HCl} \xrightarrow{\text{ZnCl}_2} \text{C}_6\text{H}_5\text{CHO} + \text{HCl} \] ### Summary 1. **Sandmeyer Reaction**: - Converts aryl amines into aryl halides using aryl diazonium salts and copper(I) halides. - Example: Aniline to chlorobenzene using CuCl. 2. **Gattermann Reaction**: - Adds a formyl group to the aromatic ring using aryl halides, carbon monoxide, and hydrogen chloride (or Lewis acids). - Example: Chlorobenzene to benzaldehyde using CO and HCl. Both reactions are pivotal in aromatic chemistry and are used widely for producing aryl halides and aryl aldehydes in synthetic organic chemistry. You 12/03/2024 06:15 PM Reactions of aryl halides: Aromatic nucleophilic substitution (replacement by –OH group and effect of nitro substituent AI Chat 12/03/2024 06:16 PM Aryl halides (halogen-substituted aromatic compounds) exhibit unique chemical behavior due to the reactivity of the aromatic ring. One of the significant reactions involving aryl halides is **aromatic nucleophilic substitution**, where a nucleophile replaces the halogen atom on the aromatic ring. This process is often referred to as the **S_NAr (nucleophilic aromatic substitution)** mechanism. Below, we discuss the mechanism of S_NAr, the replacement of a halogen with a hydroxyl (-OH) group, and the effect of nitro substituents on this reaction. ### 1. Aromatic Nucleophilic Substitution (S_NAr) **Mechanism:** The S_NAr mechanism typically involves two key steps: - **Formation of a Meisenheimer Complex:** The nucleophile attacks the aromatic ring at the carbon atom bearing the halogen, resulting in the formation of a negatively charged intermediate (Meisenheimer complex). This complex is stabilized by strong electron-withdrawing groups, usually present on the ring. - **Elimination of the Halide:** The halogen atom leaves the aromatic ring as a halide ion, leading to the formation of the substituted aromatic compound. ### Example: Replacement by Hydroxyl Group (-OH) An example of aromatic nucleophilic substitution where the aryl halide is converted into a phenol (substituted with -OH) involves the following reaction: #### Reaction with Hydroxide Ion (OH⁻): 1. **Starting Material:** Aryl halide (e.g., chlorobenzene). 2. **Nucleophile:** Hydroxide ion (OH⁻). 3. **Reaction Conditions:** The reaction often requires the presence of a strong base (e.g., NaOH) and sometimes elevated temperature. **General Reaction:** \[ \text{Ar-Cl} + \text{OH}^- \rightarrow \text{Ar-OH} + \text{Cl}^- \] **Example:** - **Chlorobenzene to Phenol:** \[ \text{C}_6\text{H}_5\text{Cl} + \text{NaOH} \rightarrow \text{C}_6\text{H} _5\text{OH} + \text{NaCl} \] (Chlorobenzene + Sodium hydroxide → Phenol + Sodium chloride) ### 2. Effect of Nitro Substituent (-NO₂) The presence of electron-withdrawing groups, particularly nitro groups (-NO₂), on the aromatic ring significantly enhances the reactivity of aryl halides towards nucleophilic substitution. This effect is due to the following reasons: - **Increased Reactivity:** The nitro group is a strong electron-withdrawing group through both resonance and inductive effects. Its presence on the aromatic ring stabilizes the negative charge developed in the Meisenheimer complex by delocalizing it into the nitro group, facilitating the attack of the nucleophile. - **Meta vs. Para Substitution:** While the nitro group directs incoming nucleophiles to the *ortho* and *para* positions in electrophilic aromatic substitution reactions, in the context of nucleophilic aromatic substitution, it typically enhances the rates of substitution at the *para* or *ortho* position relative to itself. **Example of Nitro-Substituted Aryl Halides:** Consider the nucleophilic substitution of **4-nitrochlorobenzene**: 1. **Starting Material:** 4-Nitrochlorobenzene. 2. **Nucleophile:** Hydroxide ion (OH⁻). 3. **Reaction:** \[ \text{4-NO}_2\text{C}_6\text{H}_4\text{Cl} + \text{OH}^- \rightarrow \text{4-NO}_2\text{C}_6\text{H}_4\text{OH} + \text{Cl}^- \] Here, the 4-nitrochlorobenzene reacts to form 4-nitrophenol. ### Summary - **Aromatic Nucleophilic Substitution (S_NAr)** involves the replacement of a halogen atom on an aromatic ring with a nucleophile, typically in a two-step mechanism of complex formation followed by halide elimination. - **Replacing with Hydroxyl (-OH):** The nucleophile in this case can be hydroxide ion, leading to the formation of phenols such as phenol from chlorobenzene. - **Effect of Nitro Substituent:** The nitro group acts as a strong electron-withdrawing group, enhancing the rate of nucleophilic substitution and stabilizing the Meisenheimer complex, facilitating the substitution of halides in aromatic compounds. Understanding these mechanisms is crucial for organic synthesis, as it provides insights into how functional groups influence reactivity and can be utilized for diverse applications in synthetic chemistry. You 12/03/2024 06:16 PM Aryl Halides: Benzyne mechanism: K(Na)NH2/NH3 AI Chat 12/03/2024 06:16 PM The **Benzyne mechanism** is a significant concept in organic chemistry that describes a particular pathway of reactivity for aryl halides. This mechanism is especially relevant when discussing nucleophilic aromatic substitution reactions, especially those carried out by strong bases such as sodium amide (NaNH₂) in ammonia (NH₃) solvent. ### Overview of the Benzyne Mechanism Aryl halides (compounds wherein a halogen atom is directly bonded to an aromatic ring) generally do not participate in nucleophilic substitution reactions like alkyl halides due to the stability of the aromatic system. However, under specific conditions, a more reactive intermediate called **benzyne** can be generated, allowing for substitution reactions to occur. #### Benzyne Formation 1. **Deprotonation:** Sodium amide (NaNH₂) is a strong base that can deprotonate an acidic hydrogen atom adjacent to the aryl halide at ortho or para positions, generating a negative charge localized on the aromatic system. 2. **Halide Elimination:** The next step involves the elimination of the halide ion (X⁻) resulting in the formation of the **benzyne intermediate**. This process occurs through a mechanism in which the triple bond forms between the carbon atoms that were initially bonded to the halide and the adjacent carbon with the negative charge. **Reaction Steps:** \[ \begin{align*} \text{Step 1:} & \quad \text{Ar-H} + \text{NaNH}_2 \rightarrow \text{Ar}^- + \text{NH}_3 \\ \text{Step 2:} & \quad \text{Ar}^- \text{ + Ar-X} \rightarrow \text{Benzyne + NaX} \end{align*} \] Example of the benzyne formation: - For chlorobenzene (C₆H₅Cl), the reaction with sodium amide can represent the overall process: \[ \text{C}_6\text{H}_5\text{Cl} + \text{NaNH}_2 \rightarrow \text{C} _6\text{H}_4 \equiv \text{C} + \text{NaCl} + \text{NH}_3 \] ### Reactivity of Benzyne Once formed, the highly reactive benzyne intermediate can react with a variety of nucleophiles. The nucleophile attacks one of the carbons in the triple bond, leading to a nucleophilic aromatic substitution product. - **Nucleophilic Attack:** Nucleophiles such as water (OH⁻), alkoxides (RO⁻), and other nucleophilic agents can react with the benzyne: \[ \text{Benzyne} + \text{Nu}^- \rightarrow \text{Substituted Aryl Compound} \] **Example:** If benzyne reacts with hydroxide ion: \[ \text{C}_6\text{H}_4 \equiv \text{C} + \text{OH}^- \rightarrow \text{C} _6\text{H}_4\text{(OH)} + \text{H}^- \] This results in the formation of a phenol derivative. ### Summary - **Conditions:** The Benzyne mechanism typically requires strong bases (like NaNH₂) and polar aprotic solvents (like NH₃) for effective nucleophilic substitution at aryl halides. - **Reactions:** The mechanism offers a pathway for aryl halides that are normally inert to nucleophilic substitution to react and provide substituted derivatives. - **Nucleophiles Fate:** After the formation of benzyne, the choice of nucleophile dictates the final substitution product, allowing chemists to create diverse functional groups on the aromatic ring. ### Conclusion The Benzyne mechanism represents a fascinating aspect of aryl halide chemistry, particularly showcasing how strong bases can facilitate nucleophilic substitutions in systems typically resistant to such transformations. Understanding this mechanism is crucial for synthetic organic chemistry and offers pathways to various functionalized aromatic derivatives. You 12/03/2024 06:16 PM Reactivity and relative strength of Carbon-Halogen bond in alkyl, allyl, benzyl, vinyl and aryl halides. AI Chat 12/03/2024 06:16 PM The reactivity and relative strength of the carbon-halogen (C-X) bond in various types of halides (alkyl, allyl, benzyl, vinyl, and aryl halides) depend significantly on the structure of the carbon atom to which the halogen is attached and the nature of the halogen itself. Here's a detailed analysis: ### 1. General Overview of Carbon-Halogen Bonds The strength of the carbon-halogen bond varies with the type of halogen (F, Cl, Br, I), as well as the hybridization of the carbon atom: - **C-F bond**: Strongest due to the small size and high electronegativity of fluorine. - **C-Cl bond**: Strong, but weaker than C-F. - **C-Br bond**: Weaker than C-Cl due to larger size and increased orbital overlap. - **C-I bond**: Weakest due to poor overlap, leading to a larger bond length and lower bond energy. ### 2. Reactivity of Carbon-Halogen Bonds The reactivity of the C-X bond can be influenced by several factors including the structure of the compound (primary, secondary, tertiary) and the stability of formed intermediates (e.g., carbocations, radicals). **Comparison of Carbon-Halogen Reactivity:** - **Alkyl Halides (primary, secondary, tertiary):** - **Primary Alkyl Halides (e.g., CH₃CH₂Br)**: Generally undergo S_N2 reactions, where the bond strength is significant but still allows for nucleophilic attack. - **Secondary Alkyl Halides (e.g., (CH₃)₂CHBr)**: Can undergo either S_N2 or S_N1 reactions, making them moderately reactive. - **Tertiary Alkyl Halides (e.g., (CH₃)₃CBr)**: Favor S_N1 mechanisms due to carbocation stability; C-X bond is weaker because of the steric hindrance, leading to easier dissociation. - **Allyl and Benzyl Halides:** - **Allyl Halides (e.g., CH₂=CH-CH₂Br)**: Show significant reactivity due to resonance stabilization of the allylic carbocation. The C-Br bond is relatively strong, but the ability to form a stable carbocation accelerates nucleophilic substitution. - **Benzyl Halides (e.g., C₆H₅-CH₂Br)**: Highly reactive as well due to resonance stabilization of the benzyl carbocation formed during the S_N1 reaction. The C-Br bond is relatively strong but breaks more readily due to the stability of the resulting intermediate. - **Vinyl Halides (e.g., CH₂=CH-Br):** - The C-Br bond in vinyl halides is relatively strong and less reactive toward nucleophilic substitution compared to alkyl halides because they cannot form stable carbocations through S_N1 mechanisms. Vinyl halides primarily undergo reactions via electrophilic addition rather than substitution due to this bond stability. - **Aryl Halides (e.g., C₆H₅-Cl):** - The C-X bond in aryl halides is very strong because the carbon is sp² hybridized. Aryl halides generally exhibit very low reactivity toward nucleophilic substitution under normal conditions, as the C-Cl bond is stable and the aromatic ring participates in resonance that stabilizes the structure. They may undergo substitution reactions like the nucleophilic aromatic substitution but require strong electrophiles or other specific conditions. ### Summary of Reactivity and Bond Strength **Order of Reactivity (from most to least reactive towards nucleophilic substitution):** 1. **Benzyl Halides** (high reactivity due to resonance stabilization) 2. **Allyl Halides** (high reactivity due to resonance stabilization) 3. **Tertiary Alkyl Halides** (favors S_N1 due to stable carbocation) 4. **Secondary Alkyl Halides** (may undergo S_N1 or S_N2) 5. **Primary Alkyl Halides** (generally undergo S_N2) 6. **Vinyl Halides** (strong C-Br bond, mainly undergo addition reactions) 7. **Aryl Halides** (very low reactivity, strong C-X bond) ### Bond Strengths (relative, from strongest to weakest): 1. **Aryl Halides** (C-X bond is strongest due to sp² hybridization) 2. **Vinyl Halides** (C-X bond is strong due to sp² hybridization) 3. **Alkyl Halides** (strength varies; tertiary < secondary < primary) 4. **Allyl Halides** (weak due to resonance stabilization) 5. **Benzyl Halides** (weak due to resonance stabilization) 6. **Halogens Order**: C-F > C-Cl > C-Br > C-I (overall weaker bonds for heavier halogens) This overview highlights the importance of structural context and the nature of the halogen when considering the reactivity and bond strength of carbon-halogen bonds in various types of halides. You 12/03/2024 06:17 PM Alcohols:Preparation of primary, secondary and tertiary alcohols using Grignard reagent, AI Chat 12/03/2024 06:17 PM **Grignard reagents** are organomagnesium compounds (RMgX) that play a significant role in organic synthesis, particularly for preparing alcohols. Grignard reagents react with various carbonyl compounds to yield alcohols upon hydrolysis. The nature of the alcohol formed (primary, secondary, or tertiary) depends on the type of carbonyl compound used in the reaction. ### 1. Preparation of Primary Alcohols Primary alcohols can be synthesized from Grignard reagents through the reaction with formaldehyde (H₂C=O). #### a. **Mechanism:** 1. The Grignard reagent reacts with formaldehyde to form a primary alcohol. 2. The resulting alkoxide intermediate is then hydrolyzed in the presence of water or dilute acid to yield the primary alcohol. #### **General Reaction:** \[ \text{R-MgX} + \text{H}_2\text{C=O} \rightarrow \text{R-CH}_2\text{OH} + \text{MgX(OH)} \] #### **Example:** - **Preparation of Ethanol:** \[ \text{CH}_3\text{MgBr} + \text{H}_2\text{C=O} \rightarrow \text{CH} _3\text{CH}_2\text{OH} + \text{MgBr(OH)} \] In this case, methyl magnesium bromide (CH₃MgBr) reacts with formaldehyde to produce ethanol (CH₃CH₂OH). ### 2. Preparation of Secondary Alcohols Secondary alcohols can be produced by reacting Grignard reagents with other aldehydes (except formaldehyde). #### a. **Mechanism:** 1. The Grignard reagent adds to the carbonyl carbon of an aldehyde, leading to the formation of a secondary alcohol after hydrolysis. 2. The alkoxide intermediate formed in the first step is hydrolyzed to yield the corresponding secondary alcohol. #### **General Reaction:** \[ \text{R-MgX} + \text{R'-CHO} \rightarrow \text{R-R'-C(OH)-X} + \text{MgX(OH)} \] #### **Example:** - **Preparation of Isopropyl Alcohol:** \[ \text{CH}_3\text{MgBr} + \text{CH}_3\text{CHO} \rightarrow \text{CH} _3\text{C(OH)(CH}_3\text{)} + \text{MgBr(OH)} \] In this case, methyl magnesium bromide (CH₃MgBr) reacts with acetaldehyde (CH₃CHO) to form isopropyl alcohol (2-propanol, CH₃CHOHCH₃). ### 3. Preparation of Tertiary Alcohols Tertiary alcohols can be synthesized by reacting Grignard reagents with ketones. #### a. **Mechanism:** 1. The Grignard reagent reacts with a ketone, forming a tertiary alcohol after hydrolysis. 2. As with primary and secondary alcohols, the alkoxide intermediate is converted into the alcohol upon treatment with water or acid. #### **General Reaction:** \[ \text{R-MgX} + \text{R'-(C=O)-R''} \rightarrow \text{R-R'-C(OH)-R''} + \text{MgX(OH)} \] #### **Example:** - **Preparation of Tertiary butyl Alcohol:** \[ \text{C}_4\text{H}_9\text{MgBr} + \text{(CH}_3\text{)}_2\text{CO} \rightarrow \text{(CH}_3\text{)}_3\text{COH} + \text{MgBr(OH)} \] In this example, butyl magnesium bromide reacts with acetone ((CH₃)₂CO) to yield tert-butyl alcohol ((CH₃)₃COH). ### Summary - **Primary Alcohols:** Formed by the reaction of Grignard reagents with formaldehyde. - **Secondary Alcohols:** Prepared by the reaction of Grignard reagents with other aldehydes. - **Tertiary Alcohols:** Synthesized by the reaction of Grignard reagents with ketones. Grignard reagents are versatile tools in organic synthesis, allowing for the efficient preparation of different types of alcohols depending on the carbonyl compound utilized. They highlight the significance of nucleophilic addition reactions in generating functionalized organic compounds. You 12/03/2024 06:17 PM Alcohols: ester hydrolysis AI Chat 12/03/2024 06:17 PM **Ester hydrolysis** is a chemical reaction that involves the cleavage of an ester bond, resulting in the formation of an alcohol and a carboxylic acid. This process can be catalyzed by an acid or a base and is an important reaction in both organic chemistry and biological processes. ### Overview of Ester Hydrolysis Ester hydrolysis is typically represented by the following general equation: \[ \text{RCOOR'} + \text{H}_2\text{O} \rightarrow \text{RCOOH} + \text{R'OH} \] Where: - RCOOR' is the ester (with R representing an alkyl or aryl group and R' representing another alkyl or aryl group). - H₂O is water. - RCOOH is the resulting carboxylic acid. - R'OH is the resulting alcohol. ### Mechanism of Ester Hydrolysis Ester hydrolysis can occur through two mechanisms: **acid-catalyzed hydrolysis** (using an acid) and **base-catalyzed hydrolysis** (also known as saponification). #### 1. Acid-Catalyzed Hydrolysis In acid-catalyzed hydrolysis, the reaction generally proceeds through the following steps: 1. **Protonation of the Ester:** The carbonyl oxygen of the ester is protonated by the acid, increasing the electrophilicity of the carbonyl carbon. \[ \text{RCOOR'} + \text{H}^+ \rightarrow \text{RCOOR'}^+ \] 2. **Nucleophilic Attack:** Water acts as a nucleophile, attacking the electrophilic carbon of the protonated ester, leading to the formation of a tetrahedral intermediate. \[ \text{RCOOR'}^+ + \text{H}_2\text{O} \rightarrow \text{Tetrahedral intermediate} \] 3. **Proton Transfer and Departure of Alcohol:** A proton transfer occurs within the intermediate, followed by the departure of R'OH, regenerating the acid and resulting in the formation of the carboxylic acid. \[ \text{Tetrahedral intermediate} \rightarrow \text{RCOOH} + \text{R'OH} \] #### Summary of Acid-Catalyzed Hydrolysis - **Conditions:** Requires the presence of an acid (typically a strong acid, such as HCl, H₂SO₄). - **Reactants:** Ester and water. - **Products:** Carboxylic acid and alcohol. #### 2. Base-Catalyzed Hydrolysis (Saponification) In base-catalyzed hydrolysis, known as saponification, an ester reacts with a strong base, typically sodium hydroxide (NaOH) or potassium hydroxide (KOH). The general mechanism is as follows: 1. **Nucleophilic Attack:** The hydroxide ion (OH⁻) from the base acts as a nucleophile, attacking the carbonyl carbon of the ester. \[ \text{RCOOR'} + \text{OH}^- \rightarrow \text{Tetrahedral intermediate} \] 2. **Departure of Alcohol:** The tetrahedral intermediate rearranges, resulting in the formation of a carboxylate ion and the release of alcohol (R'OH). \[ \text{Tetrahedral intermediate} \rightarrow \text{RCOO}^- + \text{R'OH} \] 3. **Protonation:** If the reaction is conducted in a neutral or acidic environment afterward, the carboxylate ion can be protonated to produce the carboxylic acid. \[ \text{RCOO}^- + \text{H}_2\text{O} \rightarrow \text{RCOOH} + \text{OH} ^- \] #### Summary of Base-Catalyzed Hydrolysis (Saponification) - **Conditions:** Requires a strong base (e.g., NaOH, KOH). - **Reactants:** Ester and hydroxide ion. - **Products:** Carboxylate salt and alcohol. If treated with acid, the carboxylate can form the carboxylic acid. ### Applications of Ester Hydrolysis 1. **Soap Production:** Saponification is the basis for soap production, where triglycerides (fats/oils) are hydrolyzed to form glycerin and fatty acid salts (soaps). 2. **Biodiesel Production:** Ester hydrolysis is central in transesterification processes to produce biodiesel from triglycerides. 3. **Analytical Chemistry:** Ester hydrolysis can be used in quantitative and qualitative analysis in organic synthesis and testing. ### Conclusion Ester hydrolysis is a fundamental reaction in organic chemistry, providing a route to form carboxylic acids and alcohols from esters in both acidic and basic conditions. Understanding this reaction is crucial for applications in synthetic chemistry, industrial processes, and understanding biological reactions involving esters. You 12/03/2024 06:17 PM Alcohols : reduction of aldehydes AI Chat 12/03/2024 06:17 PM The reduction of aldehydes is a crucial reaction in organic chemistry that transforms aldehydes into alcohols. Aldehydes (R-CHO) are reactive functional groups that can undergo reduction via various reducing agents under different conditions. ### Overview of Aldehyde Reduction In this process, the carbonyl group (C=O) of the aldehyde is converted into a hydroxyl group (-OH), resulting in the formation of a primary alcohol (R-CH₂OH). **General Reaction:** \[ \text{R-CHO} + \text{[H]} \rightarrow \text{R-CH}_2\text{OH} \] ### Common Reducing Agents 1. **Sodium Borohydride (NaBH₄):** - NaBH₄ is a mild reducing agent commonly used to reduce aldehydes to primary alcohols. It acts selectively on aldehydes without affecting other functional groups like ketones or esters. - **Reaction:** \[ \text{R-CHO} + \text{NaBH}_4 \rightarrow \text{R-CH}_2\text{OH} + \text{NaB(OH)}_4 \] 2. **Lithium Aluminum Hydride (LiAlH₄):** - LiAlH₄ is a more potent reducing agent and can reduce not only aldehydes but also ketones, esters, and other functional groups. It reacts violently with water, so it is usually used in anhydrous conditions. - **Reaction:** \[ \text{R-CHO} + \text{LiAlH}_4 \rightarrow \text{R-CH}_2\text{OH} + \text{LiAl(OH)}_4 \] 3. **Catalytic Hydrogenation:** - This method utilizes hydrogen gas (H₂) in the presence of a catalyst, typically palladium (Pd), platinum (Pt), or nickel (Ni). It’s an effective way to reduce aldehydes under high pressure and temperature conditions. - **Reaction:** \[ \text{R-CHO} + \text{H}_2 \xrightarrow{\text{Pd}} \text{R-CH} _2\text{OH} \] 4. **Other Reducing Agents:** - **Formic Acid:** It can act as a reducing agent under certain conditions in the presence of catalysts. - **Hydride Reductants**: Other hydride reagents such as Diisobutylaluminum hydride (DIBAL-H) can also reduce aldehydes to alcohols. ### Mechanism of Aldehyde Reduction The general mechanism for the reduction of an aldehyde involves the following steps: 1. **Nucleophilic Attack:** The reducing agent donates a hydride ion (H⁻) to the carbon atom of the carbonyl group, resulting in the formation of a tetrahedral intermediate. 2. **Protonation:** The intermediate then undergoes protonation, often from a solvent or water, leading to the formation of the alcohol. ### Example of Aldehyde Reduction - **Reduction of Formaldehyde (Methanal) to Methanol:** \[ \text{HCHO} + \text{NaBH}_4 \rightarrow \text{CH}_3\text{OH} \] - **Reduction of Acetaldehyde (Ethanal) to Ethanol:** \[ \text{CH}_3\text{CHO} + \text{LiAlH}_4 \rightarrow \text{CH}_3\text{CH} _2\text{OH} \] ### Summary The reduction of aldehydes to alcohols is a fundamental reaction in organic synthesis, enabling the conversion of carbonyl compounds into alcohols. Various reducing agents, including sodium borohydride, lithium aluminum hydride, and hydrogen in the presence of a catalyst, can effectively facilitate this transformation. Understanding the conditions and mechanisms involved in these reactions is essential for various applications in synthetic organic chemistry, the pharmaceutical industry, and the production of fine chemicals. You 12/03/2024 06:17 PM Alcohols : ketones AI Chat 12/03/2024 06:18 PM **Alcohols** and **ketones** are important classes of organic compounds in organic chemistry. Conversion between these two functional groups can be achieved through various methods. Below, we discuss the properties of alcohols and ketones, and the key processes to convert alcohols into ketones. ### Alcohols #### Definition: Alcohols are organic compounds containing one or more hydroxyl (-OH) groups attached to a carbon atom. The general formula for alcohols is R-OH, where R is an alkyl or aromatic group. #### Classification: 1. **Primary Alcohols (1°)**: The carbon atom bearing the hydroxyl group is attached to one other carbon atom (e.g., ethanol). 2. **Secondary Alcohols (2°)**: The carbon atom with the hydroxyl group is attached to two other carbon atoms (e.g., isopropanol). 3. **Tertiary Alcohols (3°)**: The carbon atom with the hydroxyl group is bound to three other carbon atoms (e.g., tert-butanol). ### Ketones #### Definition: Ketones are organic compounds that contain a carbonyl group (C=O) flanked by two carbon atoms. The general formula is R-CO-R', where R and R' can be either alkyl or aryl groups. #### Characteristics: - Ketones are typically named by replacing the "-e" ending of the corresponding alkane with "-one." - They are commonly found in everyday substances like nail polish remover (acetone). ### Converting Alcohols to Ketones The conversion of alcohols to ketones primarily involves oxidation reactions. The nature of the alcohols (primary, secondary, or tertiary) determines the pathway taken for the conversion. #### 1. **Oxidation with Mild Oxidizing Agents:** - **Secondary Alcohol to Ketone:** Secondary alcohols are typically oxidized to ketones using mild oxidizing agents such as chromium trioxide (CrO₃) or pyridinium chlorochromate (PCC). **Reaction:** \[ \text{R}_2\text{CHOH} \xrightarrow{\text{[O]}} \text{R}_2\text{C=O} + \text{H}_2\text{O} \] **Example:** - Isopropanol (2-propanol) oxidized to acetone (2-propanone). \[ \text{(CH}_3)_2\text{CHOH} \xrightarrow{\text{PCC}} \text{(CH} _3)_2\text{C=O} + \text{H}_2\text{O} \] #### 2. **Oxidation of Primary Alcohols:** - Primary alcohols (1°) are typically oxidized to aldehydes first and can subsequently be oxidized to carboxylic acids: - However, using certain techniques (such as controlled oxidation), primary alcohols can be selectively converted to ketones, though this is less common. **Example:** \[ \text{R-CH}_2\text{OH} \xrightarrow{\text{[O]}} \text{R-CHO} \xrightarrow{\text{[O]}} \text{R-COOH} \] #### 3. **Using Grignard Reagents:** - Secondary alcohols can also be formed from ketones using Grignard reagents. If working backward, ketones can be reduced to secondary alcohols and then oxidized back to ketones, albeit not a direct conversion. **Reaction:** \[ \text{R-CO-R'} + \text{RMgX} \rightarrow \text{R-C(OH)-R'} + \text{MgXOH} \] #### 4. **Dehydration of Alcohols:** Though not directly converting alcohols to ketones, it's worth noting that when certain secondary alcohols undergo dehydration (removal of water), they may form alkenes, which can then be oxidized to ketones. **Example:** - Isopropanol can undergo dehydration to form propene, which can subsequently be oxidized to form acetone. ### Conclusion The conversion of alcohols to ketones primarily relies on oxidation reactions, with secondary alcohols being the most straightforward substrates for this transformation. The choice of oxidizing agent, along with the structure of the alcohol, is crucial in determining the success and selectivity of the reaction. This transformation is significant in organic synthesis, contributing to the production of various ketones used in pharmaceuticals, fragrances, and industrial chemicals. You 12/03/2024 06:18 PM Alcohols : carboxylic acids and esters. AI Chat 12/03/2024 06:18 PM Alcohols are important functional groups in organic chemistry that can be transformed into various derivatives, including carboxylic acids and esters. Below, we will examine the processes by which alcohols can be converted into carboxylic acids and esters, along with relevant mechanisms and examples. ### 1. Conversion of Alcohols to Carboxylic Acids #### a. **Oxidation of Alcohols:** The primary method for converting alcohols into carboxylic acids is through oxidation. There are two types of alcohols that can be oxidized: 1. **Primary Alcohols:** These can be fully oxidized to form carboxylic acids. Common oxidizing agents include potassium dichromate (K₂Cr₂O₇), chromium trioxide (CrO₃), and sodium hypochlorite (NaOCl). **Example:** - Ethanol (C₂H₅OH) can be oxidized to acetic acid (CH₃COOH). **Reaction:** \[ \text{CH}_3\text{CH}_2\text{OH} \xrightarrow[\text{H₂O}]{\text{[O]}} \text{CH}_3\text{COOH} \] 2. **Secondary Alcohols:** Secondary alcohols can be oxidized to ketones, not carboxylic acids. However, if they undergo further oxidation under extreme conditions, they can be converted to carboxylic acids. 3. **Tertiary Alcohols:** These cannot be oxidized to carboxylic acids because there is no hydrogen atom attached to the carbon bearing the -OH group. #### b. **Oxidative Cleavage:** In some cases, alcohols can be cleaved using strong oxidizing agents to ultimately yield carboxylic acids when using compounds like ozone (O₃) followed by oxidative workup. ### 2. Conversion of Alcohols to Esters #### a. **Esterification Reaction (Fischer Esterification):** The most common method to form esters from alcohols is through an esterification reaction. This process typically involves the reaction of an alcohol with a carboxylic acid in the presence of an acid catalyst. **General Reaction:** \[ \text{R-OH} + \text{R'-COOH} \xrightarrow[\text{Acid Catalyst}]{\Delta} \text{R'-COOR} + \text{H}_2\text{O} \] Where: - R-OH is the alcohol. - R'-COOH is the carboxylic acid. - R'-COOR is the ester formed. ##### Example of Fischer Esterification: - When acetic acid reacts with ethanol: \[ \text{CH}_3\text{COOH} + \text{C}_2\text{H}_5\text{OH} \xrightarrow{H^+} \text{CH}_3\text{COOC}_2\text{H}_5 + \text{H}_2\text{O} \] (Ethyl acetate is formed along with water.) #### b. **Transesterification:** This reaction involves the exchange of the alkoxy group of an ester with an alcohol, resulting in the formation of a new ester. **General Reaction:** \[ \text{RCOOR'} + \text{R''OH} \rightarrow \text{RCOOR''} + \text{R'OH} \] ##### Example of Transesterification: - Methyl acetate can be converted to ethyl acetate using ethanol: \[ \text{CH}_3\text{COOCH}_3 + \text{C}_2\text{H}_5\text{OH} \rightarrow \text{CH}_3\text{COOC}_2\text{H}_5 + \text{CH}_3\text{OH} \] #### c. **Acid Chloride Reaction:** Alcohols can also be converted to esters by reacting with acid chlorides (also known as acyl chlorides). The reaction is typically more reactive and does not require an acid catalyst. **Example:** \[ \text{R'C(O)Cl} + \text{R-OH} \rightarrow \text{R'COOR} + \text{HCl} \] Where R'COCl is the acid chloride, and the reaction produces an ester alongside hydrochloric acid. ### Summary - **Alcohols to Carboxylic Acids**: Alcohols can be oxidized to carboxylic acids using oxidizing agents. Primary alcohols can be directly converted, while secondary alcohols require harsher conditions. - **Alcohols to Esters**: Esters can be prepared from alcohols via esterification reactions with carboxylic acids or acid chlorides, as well as through transesterification involving existing esters. These transformations are fundamental in organic synthesis, providing access to a wide range of important compounds used in various applications, including pharmaceuticals, fragrances, and industrial chemicals. You 12/03/2024 06:18 PM Alcohols : Reactions with sodium AI Chat 12/03/2024 06:18 PM Reactions of alcohols with sodium are significant in organic chemistry, especially in the preparation of alkoxides. Sodium can react with alcohols to form sodium alkoxides and hydrogen gas. Below is a detailed exploration of this reaction: ### Reaction of Alcohols with Sodium 1. **General Reaction:** The reaction between sodium (Na) and an alcohol (R-OH) typically results in the formation of a sodium alkoxide (R-O⁻Na⁺) and hydrogen gas (H₂). The reaction can be represented as follows: \[ 2 \text{R-OH} + 2 \text{Na} \rightarrow 2 \text{R-O}^- \text{Na}^+ + \text{H}_2 \uparrow \] Where: - R-OH is the alcohol. - R-O⁻Na⁺ is the corresponding sodium alkoxide. - H₂ is the hydrogen gas released in the reaction. 2. **Mechanism:** - **Deprotonation:** In this reaction, sodium donates an electron to the hydrogen atom of the hydroxyl group (-OH) of the alcohol, resulting in the formation of an alkoxide ion (R-O⁻) and the release of hydrogen gas (H₂). - The alcohol loses a hydrogen atom from its hydroxyl group, leading to the generation of the alkoxide species. 3. **Example Reactions:** To illustrate this reaction, consider the following examples: - **Reaction with Ethanol:** \[ 2 \text{C}_2\text{H}_5\text{OH} + 2 \text{Na} \rightarrow 2 \text{C} _2\text{H}_5\text{O}^- \text{Na}^+ + \text{H}_2 \uparrow \] (Ethanol reacting with sodium produces sodium ethoxide and hydrogen gas.) - **Reaction with Butanol:** \[ 2 \text{C}_4\text{H}_{10}\text{O} + 2 \text{Na} \rightarrow 2 \text{C} _4\text{H}_{9}\text{O}^- \text{Na}^+ + \text{H}_2 \uparrow \] (Butanol reacting with sodium produces sodium butoxide and hydrogen gas.) 4. **Properties of Sodium Alkoxides:** - **Solubility:** Sodium alkoxides are usually soluble in organic solvents and can serve as strong bases and nucleophiles in various synthetic reactions. - **Reactivity:** Sodium alkoxides can participate in reactions such as nucleophilic substitution and Williamson ether synthesis, making them valuable intermediates in organic synthesis. 5. **Applications:** - **Formation of Alkoxides:** This reaction is used to generate alkoxide salts, which can be used in various chemical reactions (e.g., in Williamson synthesis of ethers). - **Strong Base:** Sodium alkoxides are powerful bases that can deprotonate weak acids. - **Reaction with Carbonyl Compounds:** Sodium alkoxides can react with carbonyls in condensation reactions to form alcohols or other functional groups. ### Summary The reaction of alcohols with sodium leads to the formation of sodium alkoxides and hydrogen gas. This reaction is key in organic synthesis, particularly for creating reactive alkoxide intermediates that can further participate in different chemical transformations. Understanding this reaction is critical in various fields such as organic chemistry, pharmaceuticals, and material science. You 12/03/2024 06:18 PM Alcohols : HX (Lucas Test) AI Chat 12/03/2024 06:18 PM The **Lucas Test** is a qualitative analytical method used to distinguish between primary, secondary, and tertiary alcohols based on their reactivity with hydrogen halides (HX) such as hydrochloric acid (HCl) or hydrobromic acid (HBr). This test is particularly useful in organic chemistry for identifying the structure of alcohols. ### Principle of the Lucas Test The Lucas Test relies on the different rates at which primary, secondary, and tertiary alcohols react with hydrogen halides. The test involves the conversion of alcohols into alkyl halides. The rate of reaction is influenced by the stability of the carbocation intermediate formed during the process: 1. **Tertiary alcohols** form stable tertiary carbocations, leading to rapid reaction and immediate formation of the corresponding alkyl halide. 2. **Secondary alcohols** form less stable secondary carbocations. The reaction is slower compared to tertiary alcohols, but still noticeable. 3. **Primary alcohols** tend to form unstable primary carbocations or react by an S_N2 mechanism, resulting in a very slow reaction; thus, they do not produce visible products under the Lucas Test conditions. ### Procedure for the Lucas Test 1. **Preparation of Lucas Reagent:** This reagent is typically prepared by mixing hydrogen chloride (HCl) with an alcohol, often using a zinc chloride catalyst, although simple dry HCl can also be used. 2. **Testing:** - Take a small amount of the alcohol to be tested and place it in a test tube. - Add an equal volume of the Lucas reagent to the alcohol. - Shake the test tube to mix the contents thoroughly. 3. **Observation of Reaction:** - After a few minutes, observe the solution for the formation of a layer of alkyl halide or any turbidity (cloudiness) indicating that an ether or a halide has been formed. - The time taken for the formation of the alkyl halide or the appearance of cloudiness is noted. ### Interpretation of Results - **Tertiary Alcohols:** Immediate turbidity or formation of a separate layer within a matter of seconds (e.g., tert-butanol). - **Secondary Alcohols:** Cloudiness may develop after a few minutes (e.g., isopropanol will show evidence of reaction in a few minutes). - **Primary Alcohols:** No turbidity or reaction observed after several minutes, indicating minimal to no change (e.g., ethanol). ### Example of Lucas Test Application 1. **Tertiary Alcohol:** - **tert-Butanol (C₄H₁₁OH)** - Reaction with HCl will produce tert-butyl chloride and noticeable turbidity occurs immediately. 2. **Secondary Alcohol:** - **Isopropanol (C₃H₈O)** - Reaction with HCl will produce isopropyl chloride, with turbidity appearing after several minutes. 3. **Primary Alcohol:** - **Ethanol (C₂H₅OH)** - Little to no reaction is observed, as ethanol does not react significantly under the conditions of the Lucas Test. ### Limitations of the Lucas Test - **False Negatives:** Some primary alcohols (especially very hindered ones) can give slow reactions that might be interpreted as negative. - **Sensitivity to Conditions:** Reaction conditions such as temperature and concentration may affect the results. - **Not Universal:** The test is more suitable for identifying alcohols with simple structures and may not be conclusive for complex molecules. - **Formation of Mixtures:** In cases where both classes (secondary and tertiary) are present, interpreting the results may become difficult. ### Conclusion The Lucas Test is a practical approach for distinguishing between primary, secondary, and tertiary alcohols based on their reactivity with hydrogen halides. By observing the rate of reaction and formation of alkyl halides, chemists can infer the structure of unknown alcohols. This test remains a valuable tool in organic chemistry education and analysis. You 12/03/2024 06:19 PM Alcohols : esterification AI Chat 12/03/2024 06:19 PM **Esterification** is a chemical reaction that forms esters by combining an alcohol and a carboxylic acid, typically in the presence of an acid catalyst. This reaction is fundamental in organic chemistry and has wide applications in the production of fragrances, flavoring agents, solvents, and plastics. ### Overview of Esterification #### General Reaction The general reaction for esterification can be represented as follows: \[ \text{RCOOH} + \text{R'OH} \leftrightarrow \text{RCOOR'} + \text{H} _2\text{O} \] Where: - RCOOH is the carboxylic acid. - R'OH is the alcohol. - RCOOR' is the ester produced. - H₂O is water, a byproduct of this condensation reaction. ### Mechanism of Esterification The esterification reaction is typically conducted through a **condensation mechanism**, which involves the following steps: 1. **Protonation of the Carboxylic Acid:** - In the presence of an acid catalyst (like sulfuric acid), the carbonyl oxygen of the carboxylic acid is protonated, increasing the electrophilicity of the carbonyl carbon. 2. **Nucleophilic Attack:** - The alcohol (R'OH) then acts as a nucleophile and attacks the electrophilic carbonyl carbon of the carboxylic acid, forming a tetrahedral intermediate. 3. **Collapse of the Intermediate:** - The tetrahedral intermediate rearranges and expels a water molecule, resulting in the formation of the ester and regeneration of the acid catalyst. ### Characteristics of Esterification - **Reversible Reaction:** The reaction is reversible, meaning that esters can hydrolyze back into the alcohol and carboxylic acid under certain conditions (typically in the presence of water and an acid or base). - **Equilibrium:** The position of equilibrium can be shifted towards the formation of more ester by: - Removing water as it forms. - Using excess reactants (either alcohol or carboxylic acid). ### Types of Esterification 1. **Fischer Esterification:** - The classic example of esterification, involving the reaction of a carboxylic acid with an alcohol under acidic conditions. Here’s how it looks: **Example:** \[ \text{Acetic Acid} + \text{Ethanol} \rightarrow \text{Ethyl Acetate} + \text{Water} \] \[ \text{CH}_3\text{COOH} + \text{C}_2\text{H}_5\text{OH} \rightarrow \text{CH}_3\text{COOC}_2\text{H}_5 + \text{H}_2\text{O} \] 2. **Transesterification:** - Involves the reaction of an ester with an alcohol to form a different ester and a new alcohol. This process typically requires a catalyst. **Example:** \[ \text{Ethyl Acetate} + \text{Methanol} \rightarrow \text{Methyl Acetate} + \text{Ethanol} \] 3. **Esterification with Acid Chlorides:** - Reaction between an alcohol and an acid chloride, which is typically more reactive than carboxylic acids, leading to rapid formation of esters without the need for a catalyst. **Example:** \[ \text{Acetyl Chloride} + \text{Ethanol} \rightarrow \text{Ethyl Acetate} + \text{HCl} \] ### Applications of Esterification - **Flavoring and Fragrance:** Esters are often responsible for the fruity odors of many flowers and fruits, making them desirable in the flavoring and perfume industries. - **Synthesis of Polyesters:** The formation of polyesters through the repeated esterification reaction contributes to the production of materials like polyethylene terephthalate (PET), which is used in plastic bottles and textiles. - **Medicinal Compounds:** Esters derived from alcohol and carboxylic acids can also be involved in the synthesis of pharmaceuticals. ### Conclusion Esterification is a fundamental organic reaction that allows for the convenient synthesis of esters through the reaction of alcohols and carboxylic acids. Understanding this process is crucial in both industrial applications and laboratory settings where esters are utilized for their unique properties and as intermediates in the synthesis of various compounds. The reaction's reversibility and its sensitivity to reaction conditions highlight the intricacies of ester formation and their broader significance in organic chemistry. You 12/03/2024 06:19 PM Alcohols : oxidation (with PCC, alk. KMnO4, acidic dichromate, Con. HNO3) AI Chat 12/03/2024 06:19 PM The oxidation of alcohols is a fundamental reaction in organic chemistry that transforms alcohols into corresponding carbonyl compounds (aldehydes or ketones) or carboxylic acids, depending on the structure of the alcohol and the oxidizing agent used. Below, I have described the oxidation reactions of alcohols using specific oxidizing agents: PCC (Pyridinium Chlorochromate), alkaline KMnO₄, acidic dichromate, and concentrated HNO₃. ### 1. Oxidation with PCC (Pyridinium Chlorochromate) **PCC** is a mild oxidant commonly used for the selective oxidation of primary and secondary alcohols. - **Primary Alcohols:** Primary alcohols are oxidized to aldehydes under PCC conditions. Further oxidation to carboxylic acids does not occur because PCC is a mild oxidant. **Example:** \[ \text{RCH}_2\text{OH} + \text{PCC} \rightarrow \text{RCHO} + \text{by-products} \] - **Secondary Alcohols:** Secondary alcohols oxidize to ketones. **Example:** \[ \text{R}_2\text{CHOH} + \text{PCC} \rightarrow \text{R}_2\text{C=O} + \text{by-products} \] - **Tertiary Alcohols:** Tertiary alcohols remain unchanged as they do not oxidize under these conditions. ### 2. Oxidation with Alkaline KMnO₄ (Potassium Permanganate) **Alkaline KMnO₄** is a strong oxidizing agent used for oxidizing primary and secondary alcohols. - **Primary Alcohols:** Primary alcohols can be oxidized all the way to carboxylic acids due to the strong oxidative power of KMnO₄. **Example:** \[ \text{RCH}_2\text{OH} + \text{KMnO}_4 \rightarrow \text{RCOOH} + \text{by-products} \] - **Secondary Alcohols:** Secondary alcohols are oxidized to ketones. **Example:** \[ \text{R}_2\text{CHOH} + \text{KMnO}_4 \rightarrow \text{R}_2\text{C=O} + \text{by-products} \] - **Tertiary Alcohols:** Tertiary alcohols are resistant to oxidation with KMnO₄ under neutral or basic conditions. ### 3. Oxidation with Acidic Dichromate (CrO₃, H₂SO₄) **Acidic dichromate** (often a combination of dichromate ions in the presence of sulfuric acid) is a well-known oxidizing agent that can oxidize alcohols effectively. - **Primary Alcohols:** Primary alcohols are oxidized to carboxylic acids as well. **Example:** \[ \text{RCH}_2\text{OH} + \text{H}_2\text{Cr}_2\text{O}_7 + \text{H} _2\text{SO}_4 \rightarrow \text{RCOOH} + \text{Cr}^{3+} \text{ + H} _2\text{O} \] - **Secondary Alcohols:** Secondary alcohols are transformed into ketones. **Example:** \[ \text{R}_2\text{CHOH} + \text{H}_2\text{Cr}_2\text{O}_7 + \text{H} _2\text{SO}_4 \rightarrow \text{R}_2\text{C=O} + \text{Cr}^{3+} + \text{H} _2\text{O} \] - **Tertiary Alcohols:** Tertiary alcohols are not oxidized under these conditions, similar to the reactions with PCC and KMnO₄. ### 4. Oxidation with Concentrated HNO₃ (Nitric Acid) **Concentrated Nitric acid** is a strong oxidizing agent that can oxidize alcohols under sufficiently harsh conditions. - **Primary Alcohols:** Primary alcohols can be oxidized to carboxylic acids. **Example:** \[ \text{RCH}_2\text{OH} + \text{HNO}_3 \rightarrow \text{RCOOH} + \text{NO}_2 + \text{H}_2\text{O} \] - **Secondary Alcohols:** Secondary alcohols are oxidized to ketones. **Example:** \[ \text{R}_2\text{CHOH} + \text{HNO}_3 \rightarrow \text{R}_2\text{C=O} + \text{NO}_2 + \text{H}_2\text{O} \] - **Tertiary Alcohols:** Tertiary alcohols can sometimes undergo oxidation to give various products, including ketones and carboxylic acids, but they may also undergo side reactions leading to more complex mixtures. ### Summary - **PCC:** Oxidizes primary alcohols to aldehydes and secondary alcohols to ketones; does not oxidize tertiary alcohols. - **Alkaline KMnO₄:** Oxidizes primary alcohols to carboxylic acids and secondary alcohols to ketones; tertiary alcohols resist oxidation. - **Acidic Dichromate:** Oxidizes primary alcohols to carboxylic acids and secondary alcohols to ketones; tertiary alcohols are unreactive. - **Concentrated HNO₃:** Oxidizes primary alcohols to carboxylic acids, secondary alcohols to ketones, with the possibility of oxidation of tertiary alcohols depending on the conditions. These reactions are essential in organic synthesis and help in the functionalization of alcohols to more reactive carbonyl compounds or carboxylic acids. You 12/03/2024 06:19 PM Alcohols : Oxidation of diols - Pinacol-Pinacolone rearrangement. AI Chat 12/03/2024 06:20 PM The oxidation of diols is an important transformation in organic chemistry, particularly due to its utility in the synthesis of various compounds. One significant reaction related to the oxidation of diols is the **Pinacol-Pinacolone rearrangement**. This reaction illustrates how diols can be rearranged and transformed into more complex structures through oxidation. Below is a detailed explanation of this process. ### Pinacol-Pinacolone Rearrangement #### Overview The Pinacol-Pinacolone rearrangement involves the conversion of a specific class of diols, known as pinacols, into ketones called pinacolones through acid-catalyzed rearrangement. The reaction is particularly notable for its mechanistic steps and the structural changes that occur during the process. #### Structure of Pinacol and Pinacolone - **Pinacol:** 2,3-Dimethyl-2,3-butanediol (a diol).  - **Pinacolone:** 3-Pinacolone, which is a ketone derived from pinacol.  ### Mechanism of Pinacol-Pinacolone Rearrangement 1. **Protonation of the Hydroxyl Groups:** - In the presence of an acid (such as sulfuric acid or hydrochloric acid), the hydroxyl (–OH) groups of pinacol become protonated, converting them into better leaving groups (water). 2. **Formation of a Carbocation:** - The protonated diol undergoes loss of water, leading to the formation of a carbocation. Depending on the molecule's structure, this carbocation can be tertiary or secondary. 3. **Rearrangement:** - The carbocation undergoes rearrangement through a 1,2-shift. The adjacent alkyl group (in this case, from the neighboring carbon) moves to stabilize the carbocation, leading to a more stable tertiary carbocation. 4. **Deprotonation:** - Finally, a proton is lost from the carbocation, yielding the ketone (pinacolone). ### Overall Reaction The overall transformation can be summarized as follows: \[ \text{Pinacol} \stackrel{\text{Acid}}{\longrightarrow} \text{Pinacolone} \] ### Example For instance, when starting with pinacol (2,3-dimethyl-2,3-butanediol), upon treatment with a strong acid, the pinacol undergoes the following sequence: 1. **Protonation:** - The –OH groups become protonated. 2. **Loss of Water:** - Formation of a carbocation. 3. **Rearrangement:** - The adjacent methyl group shifts to stabilize the positive charge. 4. **Formation of Pinacolone:** - Deprotonation leads to the production of 3-pinacolone. ### Significance of the Reaction - **Synthetic Utility:** The Pinacol-Pinacolone rearrangement is an important transformation in organic synthesis, allowing chemists to convert relatively simple diols into more complex structures. - **Rearrangement Mechanisms:** It serves as an excellent example for studying carbocation chemistry, rearrangement, and the effects of structure on stability and reaction pathways. - **Historical Importance:** The reaction was one of the early demonstrations of the power of rearrangement reactions in organic chemistry, illustrating broader principles that apply to many other rearrangements. ### Conclusion The Pinacol-Pinacolone rearrangement is a classic organic reaction that emphasizes the principles of carbocation stability and rearrangement. Through the oxidation of diols like pinacol, it showcases the transformation of simple starting materials into valuable ketones, reinforcing the concept's relevance in organic synthesis and mechanistic studies. You 12/03/2024 06:20 PM Phenols:Preparation bycumene hydroperoxide method, from diazonium salts AI Chat 12/03/2024 06:20 PM Phenols are a class of organic compounds that contain a hydroxyl (-OH) group attached to an aromatic hydrocarbon. There are several methods for preparing phenols, two of which include the **cumene hydroperoxide method** and the **preparation from diazonium salts**. Below is a detailed explanation of both methods. ### 1. Preparation of Phenols via Cumene Hydroperoxide Method The **cumene hydroperoxide method**, also known as the **Hock process**, is a significant industrial process for synthesizing phenol. This method involves the oxidation of cumene (isopropylbenzene) to form phenol and acetone as byproducts. #### **Steps in the Cumene Hydroperoxide Method:** 1. **Formation of Cumene Hydroperoxide:** - Cumene is treated with oxygen (usually from air) under controlled conditions (heat and pressure) to form cumene hydroperoxide. - The reaction can be represented as: \[ \text{C}_6\text{H}_5\text{C(CH}_3)_2 + \text{O}_2 \rightarrow \text{C} _6\text{H}_5\text{C(CH}_3)_2\text{O-OH} \] (Cumene + Oxygen → Cumene hydroperoxide) 2. **Cleavage of Cumene Hydroperoxide:** - The cumene hydroperoxide is then subjected to acid-catalyzed cleavage (usually with an acid like sulfuric acid or phosphoric acid) to give phenol and acetone. - The reaction can be described as follows: \[ \text{C}_6\text{H}_5\text{C(CH}_3)_2\text{O-OH} \xrightarrow{\text{H} ^+} \text{C}_6\text{H}_5\text{OH} + \text{(CH}_3)_2\text{CO} \] (Cumene hydroperoxide → Phenol + Acetone) #### **Summary:** The overall process can be simplified as follows: - **Starting Material:** Cumene (isopropylbenzene) - **Reagents:** Oxygen, acid catalyst - **Products:** Phenol and acetone This method is one of the most commonly used industrial processes for producing phenol. --- ### 2. Preparation of Phenols from Diazonium Salts **Diazonium salts** can be used to prepare phenols through a process called hydrolysis. The general strategy involves converting an aromatic amine to its diazonium salt, which can then be hydrolyzed to yield the corresponding phenol. #### **Steps in the Preparation from Diazonium Salts:** 1. **Formation of Diazonium Salt:** - An aromatic amine (such as aniline) is first reacted with nitrous acid (usually generated in situ from sodium nitrite and a mineral acid like hydrochloric acid) to form the corresponding diazonium salt. - Example reaction: \[ \text{C}_6\text{H}_5\text{NH}_2 + \text{NaNO}_2 + \text{HCl} \rightarrow \text{C}_6\text{H}_5\text{N}_2^+\text{Cl}^- + \text{H}_2\text{O} \] (Aniline + Sodium nitrite + Hydrochloric acid → Benzene diazonium chloride) 2. **Hydrolysis of Diazonium Salt:** - The diazonium salt is then hydrolyzed in warm water or dilute acid to yield the corresponding phenol. - The reaction can be represented as: \[ \text{C}_6\text{H}_5\text{N}_2^+\text{Cl}^- + \text{H}_2\text{O} \rightarrow \text{C}_6\text{H}_5\text{OH} + \text{N}_2 + \text{HCl} \] (Benzene diazonium chloride + Water → Phenol + Nitrogen gas + Hydrochloric acid) #### **Summary:** - **Starting Material:** Aromatic amine (like aniline) - **Reagents:** Sodium nitrite, Hydrochloric acid, Water - **Products:** Phenol, Nitrogen gas, and Hydrochloric acid This method is particularly useful for preparing phenols with specific substitutions on the aromatic ring. --- ### Conclusion Both the **cumene hydroperoxide method** and the **preparation from diazonium salts** are effective methods for synthesizing phenols. 1. The **cumene hydroperoxide method** is widely used in industry for producing phenol and acetone from cumene. 2. The **diazonium salt method** represents a beautifully orchestrated transformation from an amine to a phenol, illustrating the versatility of diazonium chemistry. These methods highlight the reactivity of alkyl and aryl halides in forming phenolic compounds, which play crucial roles in various chemical industries, including pharmaceuticals, fragrances, and plastics. You 12/03/2024 06:20 PM Phenols : Reactions -Electophilic substitution AI Chat 12/03/2024 06:20 PM Phenols are aromatic compounds that contain a hydroxyl group (-OH) directly bonded to a benzene ring. Due to the electron-donating effect of the hydroxyl group through resonance, phenols are more reactive in electrophilic substitution reactions compared to benzene. This increased reactivity enables substitution reactions to occur more readily, particularly with electrophiles. ### Mechanism of Electrophilic Substitution Reactions of Phenols 1. **Activation of the Benzene Ring:** - The lone pair of electrons on the oxygen atom of the hydroxyl group can participate in resonance with the π system of the benzene ring, enhancing the electron density on the ortho and para positions of the phenol. - This resonance stabilization makes the phenol much more susceptible to electrophilic attack compared to simple benzene. 2. **Formation of the Sigma Complex:** - When the electrophile approaches, it attacks the ortho or para position of the aromatic ring, leading to the formation of a sigma complex (also known as an arenium ion or Wheland intermediate). 3. **Deprotonation:** - Subsequently, a proton is lost from the sigma complex, restoring the aromaticity of the ring, and resulting in the substituted aromatic compound. ### Common Electrophilic Substitution Reactions of Phenols #### 1. **Nitration:** Phenols can undergo electrophilic nitration when treated with a mixture of concentrated nitric acid and sulfuric acid. The electrophile in this reaction is the nitronium ion (\(NO_2^+\)). **Reaction Example:** \[ \text{C}_6\text{H}_5\text{OH} + \text{HNO}_3 \ \xrightarrow{\text{H} _2\text{SO}_4} \text{C}_6\text{H}_4(\text{NO}_2)\text{OH} + \text{H} _2\text{O} \] - The major products are 2-nitrophenol and 4-nitrophenol, due to the activation of the ortho and para positions. #### 2. **Sulfonation:** Phenols can also react with sulfur trioxide (\(SO_3\)) or concentrated sulfuric acid to yield sulfonic acids. **Reaction Example:** \[ \text{C}_6\text{H}_5\text{OH} + \text{SO}_3 \rightarrow \text{C}_6\text{H} _4(\text{SO}_3\text{H})\text{OH} \] - This results in the formation of 4-benzenesulfonic acid (p-hydroxybenzenesulfonic acid), with favorable substitution occurring at the para position. #### 3. **Halogenation:** Phenols react with halogens (e.g., bromine, chlorine) to form halogenated phenols. Bromine reacts with phenol even in the absence of a separate catalyst (like FeBr₃). **Reaction Example:** \[ \text{C}_6\text{H}_5\text{OH} + \text{Br}_2 \rightarrow \text{C}_6\text{H} _4(\text{Br})\text{OH} + \text{HBr} \] - The predominant product is 2-bromophenol and 4-bromophenol. #### 4. **Friedel-Crafts Reaction:** Phenols can also participate in Friedel-Crafts alkylation or acylation reactions, although they typically require catalysts (like \(AlCl_3\)) since they may deactivate the aromatic ring. **Alkylation Example:** \[ \text{C}_6\text{H}_5\text{OH} + \text{R-X} \xrightarrow{AlCl_3} \text{C} _6\text{H}_5\text{R} + \text{HX} \] **Acylation Example:** \[ \text{C}_6\text{H}_5\text{OH} + \text{RCOX} \xrightarrow{AlCl_3} \text{C} _6\text{H}_5\text{RCO} + \text{HX} \] ### Summary - **Phenols are much more reactive than benzene in electrophilic substitution reactions due to the electron-donating effect of the hydroxyl group.** - **Common electrophilic substitution reactions include nitration, sulfonation, halogenation, and Friedel-Crafts reactions.** - **The hydroxyl group's resonance effect results in prominent reactions occurring predominantly at the ortho and para positions of the aromatic ring.** Understanding these reactions is crucial for organic synthesis and the production of a wide range of organic compounds. You 12/03/2024 06:20 PM Phenols : Reactions -Electophilic substitution - nitration AI Chat 12/03/2024 06:21 PM Phenols are aromatic compounds that contain a hydroxyl (-OH) group directly attached to a benzene ring. They are characterized by their reactivity, especially in electrophilic substitution reactions. One of the notable reactions of phenols is nitration, which involves the introduction of nitro groups (-NO₂) into the aromatic system. ### Nitration of Phenols #### Overview The nitration of phenols involves the electrophilic substitution of a nitro group on the benzene ring of phenol. This reaction is typically carried out using a mixture of concentrated nitric acid (HNO₃) and concentrated sulfuric acid (H₂SO₄), which generates the active nitrating species, the nitronium ion (NO₂⁺). #### Reaction Mechanism 1. **Generation of the Electrophile:** The first step in the nitration process is the formation of the nitronium ion (NO₂⁺) from the reaction of nitric acid and sulfuric acid. This occurs as follows: \[ \text{HNO}_3 + \text{H}_2\text{SO}_4 \rightarrow \text{NO}_2^+ + \text{HSO}_4^- + \text{H}_2\text{O} \] 2. **Electrophilic Attack:** The nitronium ion generated acts as a strong electrophile. Phenol, which is activated due to the presence of the hydroxyl (–OH) group (which is an electron-donating group), undergoes electrophilic substitution with the nitronium ion. The reaction pathway can be depicted as: \[ \text{C}_6\text{H}_5\text{OH} + \text{NO}_2^+ \rightarrow \text{C} _6\text{H}_4\text{(NO}_2)\text{OH} + \text{H}^+ \] In the case of phenol, the hydroxyl group enhances the electron density of the aromatic ring, making it more reactive toward electrophilic attack, primarily at the ortho (2-position) and para (4-position) positions relative to the –OH group. 3. **Formation of the Nitro-Substituted Phenol:** The final product can be either 2-nitrophenol or 4-nitrophenol (or a mixture of both, depending on the reaction conditions): - **2-Nitrophenol:** \[ \text{C}_6\text{H}_4\text{(NO}_2)\text{OH} \] (with the nitro group at the ortho position) - **4-Nitrophenol:** \[ \text{C}_6\text{H}_4\text{(NO}_2)\text{OH} \] (with the nitro group at the para position) #### Major Products - **Ortho- and Para-Substituted Nitrophenols:** - Due to the electron-donating nature of the -OH group, the ortho and para positions are favored during nitration. - The ortho product (2-nitrophenol) and para product (4-nitrophenol) are typically the major products; however, due to sterics, the para product is often more favored. ### Summary of Nitration: - **Reagents:** Concentrated nitric acid and concentrated sulfuric acid. - **Electrophile:** Nitronium ion (NO₂⁺). - **Mechanism:** Electrophilic substitution. - **Main Products:** 2-nitrophenol and 4-nitrophenol. ### Considerations 1. **Reactivity:** The presence of the -OH group increases the electron density of the ring, making it more susceptible to electrophilic attack, compared to benzene itself, which does not have such activating groups. 2. **Influence of the Hydroxyl Group:** The -OH group directs the incoming nitro group primarily to the ortho and para positions due to resonance effects. 3. **Reaction Conditions:** The ratio of ortho to para products can be influenced by the reaction temperature, concentration of reagents, and time of reaction. Higher temperatures can sometimes favor ortho substitution due to more effective collisions but may lead to more side products. ### Conclusion The nitration of phenols is a classic electrophilic substitution reaction that highlights the influence of substituent effects in aromatic chemistry. Understanding the mechanisms and factors influencing product distribution is critical in synthetic organic chemistry, especially when designing pathways for complex organic compounds. You 12/03/2024 06:21 PM Phenols : Reactions -Electophilic substitution - nitration AI Chat 12/03/2024 06:21 PM Phenols are aromatic compounds that possess a hydroxyl (-OH) group attached to a benzene ring. They exhibit unique reactivity compared to other aromatic compounds due to the electron-donating nature of the hydroxyl group, which directs electrophilic substitution reactions to the ortho and para positions of the aromatic ring. One of the key electrophilic substitution reactions of phenols is nitration. ### Nitration of Phenols Nitration is the process in which a nitro group (-NO₂) is introduced into an aromatic compound. For phenols, the nitration reaction takes place via electrophilic aromatic substitution. #### Mechanism of Nitration The nitration of phenol typically proceeds through the following steps: 1. **Formation of the Electrophile:** - The nitronium ion (NO₂⁺) is generated from a mixture of concentrated nitric acid (HNO₃) and concentrated sulfuric acid (H₂SO₄). The sulfuric acid acts as a catalyst and helps to activate the nitric acid. - The overall reaction to generate the nitronium ion can be represented as: \[ \text{H}_2\text{SO}_4 + \text{HNO}_3 \rightarrow \text{NO}_2^+ + \text{HSO}_4^- + \text{H}_2\text{O} \] 2. **Electrophilic Attack:** - The nitronium ion acts as the electrophile and attacks the electron-rich aromatic ring of phenol. Due to the presence of the hydroxyl group, the electron density of the ring is increased, favoring substitutions at the ortho and para positions. 3. **Formation of Sigma Complex:** - This step results in the formation of a sigma complex (also called an arenium ion), where the aromaticity of the benzene ring is temporarily lost. - **Ortho Position:** The nitro group can substitute either at the ortho or para position relative to the hydroxyl group. - A representation of this can be shown as follows (let’s say para substitution): \[ \text{C}_6\text{H}_5\text{OH} + \text{NO}_2^+ \rightarrow \text{C} _6\text{H}_4(\text{NO}_2)\text{OH}^+ \text{ (sigma complex)} \] 4. **Deprotonation:** - The sigma complex then loses a proton (H⁺), restoring the aromaticity of the ring and leading to the formation of the nitrophenol product. - The final product can be either **ortho-nitrophenol** or **para-nitrophenol**, depending on where the nitro group is introduced: \[ \text{C}_6\text{H}_4(\text{NO}_2)\text{OH} + \text{H}_2\text{O} \rightarrow \text{O}-\text{C}_6\text{H}_4\text{NO}_2 + \text{H}^+ \] ### Products of Nitration The nitration of phenol predominantly yields two isomers: - **Ortho-nitrophenol (2-nitrophenol):** The nitro group is in the ortho position relative to the hydroxyl group. - **Para-nitrophenol (4-nitrophenol):** The nitro group is in the para position relative to the hydroxyl group. While both ortho and para products are formed, the para product is generally favored due to it being less sterically hindered compared to the ortho product. ### Summary of the Reaction: The overall reaction for the nitration of phenol can be summarized as: \[ \text{C}_6\text{H}_5\text{OH} + \text{HNO}_3 \xrightarrow{\text{H} _2\text{SO}_4} \text{o- or p-} \text{C}_6\text{H}_4\text{NO}_2 + \text{H} _2\text{O} \] ### Reaction Conditions - **Reagents:** Concentrated nitric acid and concentrated sulfuric acid. - **Temperature:** Ambient temperature or slightly elevated; excess heat may favor nitration at ortho positions more. - **Time:** The reaction is monitored to ensure it doesn't proceed to undesired reactions or excessive nitration. ### Key Points to Consider - The electron-donating hydroxyl group makes phenols highly reactive towards electrophiles, leading to efficient nitration. - Safety precautions should be taken due to the corrosive nature of the reagents and the toxic nature of nitro compounds. - The products can be purified by methods such as recrystallization or column chromatography for further analysis or use. This ability to functionalize the benzene ring at specific locations is a powerful tool in organic synthesis, allowing for the production of various nitro-substituted phenols used in dyes, pharmaceuticals, and other chemical intermediates. You 12/03/2024 06:21 PM Phenols : Reier-Tiemann reaction AI Chat 12/03/2024 06:21 PM The **Reimer-Tiemann reaction** is a chemical reaction that involves the ortho-formylation of phenols to produce ortho-hydroxyaldehydes. It is an important reaction for introducing a formyl group (-CHO) into the ortho position of aromatic compounds and is widely used in organic synthesis. ### Overview of the Reimer-Tiemann Reaction #### General Reaction The Reimer-Tiemann reaction can be summarized by the following general equation: \[ \text{Phenol} + \text{CHCl}_3 + \text{Base} \rightarrow \text{ortho-Hydroxyaldehyde} + \text{By-products} \] ### Mechanism of the Reimer-Tiemann Reaction The mechanism of the Reimer-Tiemann reaction involves several key steps: 1. **Formation of the Dichloroformate Ion:** - In the presence of a base (commonly sodium hydroxide, NaOH), chloroform (CHCl₃) deprotonates to generate the dichloroformate ion. The base converts the phenol into its alkoxide form, which is more reactive as a nucleophile. \[ \text{CHCl}_3 + \text{Base} \rightarrow \text{Cl}_2\text{C}(\text{Base}) + \text{HCl} \] 2. **Nucleophilic Attack:** - The alkoxide ion (formed from phenol) attacks the electrophilic carbon of the dichloroformate ion, resulting in the formation of a tetrahedral intermediate. \[ \text{Ar-O}^- + \text{Cl}_2\text{C} \rightarrow \text{Tetrahedral Intermediate} \] 3. **Rearrangement:** - The intermediate undergoes rearrangement, leading to the migration of a chloride ion and formation of an ortho hydroxyaldehyde after elimination of HCl. 4. **Formation of Ortho-Hydroxyaldehyde:** - The rearrangement yields the ortho-formed aldehyde: ortho-hydroxybenzaldehyde. \[ \text{Tetrahedral Intermediate} \rightarrow \text{Ortho-Hydroxyaldehyde} \] ### Example of the Reimer-Tiemann Reaction An example of the Reimer-Tiemann reaction is the ortho-formylation of phenol to produce ortho-hydroxybenzaldehyde: 1. **Starting Materials:** - Phenol (C₆H₅OH) - Chloroform (CHCl₃) - Base (usually NaOH) 2. **Reaction:** \[ \text{C}_6\text{H}_5\text{OH} + \text{CHCl}_3 + \text{NaOH} \rightarrow \text{o-Hydroxybenzaldehyde} + \text{By-products} \] 3. **Product:** - The main product is **ortho-hydroxybenzaldehyde** (also known as salicylaldehyde). ### Key Points - **Selectivity:** The Reimer-Tiemann reaction predominantly leads to ortho-substitution rather than para-substitution due to steric factors and the stabilization of the intermediate. - **Conditions:** A base is essential for the reaction, and it typically occurs in alkaline conditions. - **Limitations:** - The reaction is somewhat limited to certain phenolic compounds. - Strong bases can sometimes lead to additional side reactions or multiple substitutions. - The reaction does not produce a high yield of products when using sterically hindered or highly substituted phenols. ### Conclusion The Reimer-Tiemann reaction is a valuable synthetic method for producing ortho-hydroxyaldehydes from phenols. Understanding its mechanism and conditions allows chemists to use this reaction effectively in organic synthesis, particularly in the preparation of compounds with significant biological and synthetic relevance. You 12/03/2024 06:21 PM Phenols : Gattermann-Koch reaction AI Chat 12/03/2024 06:22 PM The **Gattermann-Koch reaction** is a valuable chemical reaction that involves the formylation of phenols (and other aromatic compounds) using carbon monoxide and hydrogen chloride in the presence of a Lewis acid catalyst (often aluminum chloride, AlCl₃). This reaction allows for the introduction of an aldehyde functional group (–CHO) directly onto the aromatic ring, typically yielding ortho- and para-formylated phenolic compounds. ### Overview of the Gattermann-Koch Reaction #### Reaction Mechanism 1. **Formation of the Electrophile:** The reaction begins with the generation of the electrophile, which is the formyl cation (or an equivalent formyl species). This is typically produced from carbon monoxide and hydrogen chloride in the presence of a Lewis acid catalyst. For example: \[ \text{CO} + \text{HCl} \xrightarrow[\text{AlCl}_3]{\text{Lewis Acid}} \text{H-C(=O)}^+ + \text{Cl}^- \] 2. **Electrophilic Aromatic Substitution:** The formyl cation acts as an electrophile and undergoes electrophilic aromatic substitution with the phenol. The hydroxyl group of the phenol enhances the nucleophilicity of the aromatic ring, facilitating the attack on the formyl cation. The reaction can yield both ortho and para products due to the resonance stabilization provided by the hydroxyl group. **Example Reaction:** \[ \text{C}_6\text{H}_5\text{OH} + \text{H-C(=O)}^+ \rightarrow \text{ortho} \text{ or } \text{para}-\text{C}_6\text{H}_4\text{CHO} + \text{H}_2\text{O} \] ### Conditions Required 1. **Lewis Acid Catalyst:** Aluminum chloride (AlCl₃) is commonly used to facilitate the reaction by generating the reactive formyl species from CO and HCl. 2. **Reagents:** The primary reagents needed for the Gattermann-Koch reaction are carbon monoxide (CO), hydrogen chloride (HCl), and phenol. 3. **Temperature and Pressure:** The reaction is typically run under controlled conditions, often at elevated pressure due to the use of carbon monoxide. ### Products The main products of the Gattermann-Koch reaction are ortho- and para-formyl phenols, depending on the regioselectivity of the electrophilic substitution: - **Ortho-formyl phenol (2-formylphenol)** - **Para-formyl phenol (4-formylphenol)** ### Limitations 1. **Regioselectivity:** While the reaction can yield both ortho and para products, the relative amounts depend on the nature of the phenolic compound and the conditions used. 2. **Side Reactions:** The presence of strong Lewis acids can lead to polymerization or other side reactions, especially with sensitive substrates. 3. **Safety Concerns:** Handling carbon monoxide requires safety precautions due to its toxicity. ### Applications The Gattermann-Koch reaction is useful in organic synthesis for: - Preparing aldehyde derivatives of phenolic compounds, which are important intermediates in the manufacture of pharmaceuticals, agrochemicals, and flavors. - Introducing functional groups that can further be transformed into other valuable chemical building blocks. ### Summary The Gattermann-Koch reaction is an effective method for the formylation of phenols, using carbon monoxide and hydrogen chloride in the presence of a Lewis acid. It exemplifies the principles of electrophilic aromatic substitution and demonstrates the reactivity of aromatic compounds in organic synthesis. Understanding this reaction is crucial for chemists involved in the synthesis of nitrogen-containing aromatic compounds and other derivatives. You 12/03/2024 06:22 PM Phenols : Houben- Hoesch condensation AI Chat 12/03/2024 06:22 PM **Houben-Hoesch Condensation** is a significant reaction in organic chemistry used for the synthesis of phenolic compounds, particularly aryl ethers, by reacting phenols with carbonyl compounds such as aldehydes or ketones in the presence of Lewis acids as catalysts. This reaction results in the formation of aryl-alcohol derivatives and contributes to the preparation of alkoxyphenols. ### Overview of the Houben-Hoes