Protein Modifications and Folding PDF
Document Details
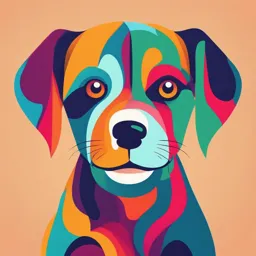
Uploaded by .keeks.
Marian University
Tags
Summary
This document discusses protein modifications and folding, including post-translational modifications, and their roles in protein regulation and function. It explains how modifications like phosphorylation influence protein activity and highlights the importance of folding in protein function.
Full Transcript
Okay, good morning, good afternoon, good evening or good night. This is probably one of our shortest lectures, it's a really kind of mini lecture and it deals all in proteins, protein modifications and then gets into our abnormal proteins and protein aggregation. So we have a few brief objectives fo...
Okay, good morning, good afternoon, good evening or good night. This is probably one of our shortest lectures, it's a really kind of mini lecture and it deals all in proteins, protein modifications and then gets into our abnormal proteins and protein aggregation. So we have a few brief objectives for this, the idea here being that we understand what can be done to a protein and how does that result in regulation and such and it really brings us full circle in all of our signaling and processing and it just finishes up molecular genetics really nicely in a functional way. So remember that we can regulate at the level of transcription, translation and now we're at the level of the protein itself, translation as well as the one we just did. So these are all post-translational modifications and protein folding. Any change that we make to a protein, any additions from subtractions, degradation can be classified as a post-translational modification. As long as we are working on a previously strong sequences of amino acids that were translated from a product, that's a post-translational modification. The most common form is usually addition of functional groups and so this is typically a readily reversible process like phosphorylation where we add the phosphate group. When it comes to protein function, we've really sort of spent time talking about domains or loss of key areas, but this really delves into the idea of also folding, how the loss of those domains can actually affect the tertiary or three-dimensional structure of the protein and thus affect the overall function. Remember that folding modifications, things that we add or remove are often critical to signaling and function and so it's not just that we need to be able to add the modification but also sometimes remove the modification. So our first one of course is the one that we have talked about previously which is phosphorylation and this is of course always a balance between a kinase and a phosphatase. The kinase is going to add the phosphate, the phosphatase is going to remove the phosphate. The thing about this is that phosphorylation can turn on a molecule or it can turn it off and so it really depends on which one we're talking about to determine what effect that phosphate is going to have and so part of the reason why we have this lecture now is to really give us a strong foundation and bring things full circle regarding biochemical processes and pathways. So just remember that when it comes to phosphorylation, we use that using our serine, threonine kinases to propagate a signal, to expand a signal and of course we are going to be using this in a regulatory capacity. When it comes to other post translational modifications, we also have the addition of other functional groups. Hydroxylation is actually going to be the addition of hydroxyl groups, sulfation is going to add sulfur groups and this is important because once we have additional sulfur groups we can actually create things like disulfide bonds which can actually play a really critical role in maintaining a tertiary structure or a folding structure. We can also add hydrophobic molecules and sugars to these. Acetylation adds acetyl groups, we can do predilation which actually adds lipid groups but we can also add lipid groups in a different conformation, specifically undergoing acyl lipidation. Ultimately, this is a lot of biochem making its way into here so we understand that when we add these things to these molecules we are changing their behavior. So moving forward, just a quick reminder, we have methylation, glycosylation, ubiquitylation and then of course we can chop things up and get rid of it. All of these modifications require their own enzymes to carry out that modification and ensure a different behavior potential or activity to the protein that is being modified. Why does this matter? Because it all changes the entire system. It gives us the ability to regulate how a change is taking place, when a change is taking place and this is really that regulation at the end of the line with the protein sitting in front of us. Remember that in order for a protein to function it must take on the right shape and have all the right functional groups it's supposed to have. Modification gives us some of those functional groups but it also helps ensure folding. We have to undergo a balance of synthesis, folding, transport and destruction for the whole thing to work properly. Folding is not always spontaneous. Some folding can be. Some folding can involve just enough close proximity biochemically and you can get the molecule to fold. Other folding is not. Other folding requires what are called molecular chaperones and those molecular chaperones actually ensure that folding can take place. When it comes to how domains and regions interact with each other, you have to have those binding elements and that ability to interact in order for folding to take place. When folding is spontaneous, it is a relatively consistent process. Protein folding is going to involve a lot of hydrophobic interactions, non covalent interactions, side chains, hydrogen bonding. All of these things are going to contribute to creating the tertiary structure. When it is spontaneous, which it frequently is, it is going to happen in a very consistent way each time unless you change the structure. If you change the underlying amino acids, you can change the folding potential. Without folding, you often don't have final function of the molecule. When folding is not spontaneous, it tends to correlate with these more complex or large proteins where we have to undergo intermediate stages. In terms of why we have this intermediate, there is some sort of kinetic interference, some sort of barrier to being able to take on that final conformation. The complexity of it, some interaction, some space constraints, something is preventing the final conformation from taking place. Because there is this kinetic interference, a partially folded or misfolded protein can actually have aberrant non-native interactions. It can become abnormal. It can treat the environment in which it is in in a way that is detrimental to that environment. We have the molecular chaperones to try to keep this from happening. Many of our chaperones are actually ATP independent, but we actually often do require energy input to take on these special conformations. We have to kind of overcome the barrier, overcome the interference. When we have a dysfunction in a chaperone, not only do we have the chaperone itself having a problem, but whatever it was supposed to help fold can be prevented from having that final function. An example of this is actually ALS. If we look at our picture to the right, what we see is that we have our translated material and we have a couple of modifications and associated proteins and we actually enter into a molecular chaperone to get to the proper place. Some of this could happen, and this is this line here, some of this could happen spontaneously and we'll be fine. Some of it could involve extra intermediates, but ultimately getting to the proper final product is what we're looking for. If we fail in those steps, we can get what we call aggregates. When we fail to achieve proper folding, the confirmation that we're in may actually promote gathering together with friends that look like us. This misfolded material can gather in such large collections that it can actually reach the level of aggregate. Protein aggregation is the bringing together of aberrantly folded molecules in a way that causes a collective mass. When this happens, it can result in clogging up processes, it can prevent transport, and when it becomes a situation where misfolded material further leads to misfolding of other material, we're at the stage of Creon. Accumulation is expected with aging and pathology. Aggregation can lead to altered growth rates, virulence in our prokaryotes, and understanding aggregation really does matter in terms of things like neurodegenerative diseases where we are impacting cellular function because of the presence of aggregated protein. If we look at this aggregation summary in bacteria, I want us to understand that we are just having misfoldings that can generate other misfoldings. This is not a know every detail of this figure. This one I want us to understand that we're using a chaperone to actually generate the folding, but some folding can occur spontaneously or it can occur in the absence of the chaperone. We can get some associated molecules to get there. This one I just want you to see that we have folding intermediates and then we get to our native protein, but that we can have misfolded proteins which could lead to their degradation or it could lead to aggregation, and so this is just showing you the different things that can happen when you have aggregation, and so you can have overexpression, you can have other inappropriate interactions, and then you can get fibrils or you can get inclusive bodies. These are problems that can lead to toxic buildup of material, further propagation of the material, and in the case of prions, what you have taken is a protein that is misfolded and it essentially becomes an infectious particle because it induces other misfolding. It becomes resistant to any sort of modification or degradation, and it leads to what are called transmissible spongiform encephalopathies. Although prions do in fact represent their own misfolded proteins, some misfolded proteins can take on that prion-like behavior I was describing. What it essentially is, is that if I am normal, I can keep altering other normal and I can create an aggregate. A fibril is an aggregate, and if I break up that aggregate, I can make additional aggregates. This has been linked to ALS, especially in regards to superoxide dismutase, which is a protein we'll talk about in Biochem, but it has also been shown in Alzheimer's. So essentially, the idea here is that we've got misfolding, but that presence of misfolding causes other misfolding. That's the infectious-like behavior. And so in terms of spongiform encephalopathies, there are actually three forms of this that have been studied, sporadic, genetic, and acquired. The majority of the cases are sporadic with a mean survival of less than six months, and those include the Kreuzfeld-Jakob disease, which is relatively well known. So sorry, I keep coughing. In terms of the genetic, this is often an familial condition, and it's going to be something that is progressive over life, as opposed to something that has that rapid six-month progression. And then of course, acquired is less than one percent, or about one percent. And these are protein. And so here's an example of some of those spongiform degenerations. So looking at this as a bigger picture, remember that none of these things are truly happening in a vacuum, and so oftentimes, especially in terms of our modifications, we can do a combination of modifications to make the most complex array of proteins that we have. So we can have a starting molecule, excuse me so much, my throat is, I'm so sorry. Anyway, we can have an initial amino acid string, and then we can renolate it, we could then methylate it, and have multiple steps to where we get to the final product. And this stepwise processing is actually a really important aspect of biochemical pathways, where we're taking one piece of material and modifying it so it can do the job that we need it to do. And if we modify it differently, it has slightly different impact, and it's a really important combination. And so if we look at this, we can actually take these multiple steps and actually relate it to activity. And so in the case of something like RELA, depending on what modification we have on it, we can either induce activity or suppress it. In terms of transcription. And so it's really an interesting reality of methylation and phosphorylation being able to talk to each other, methylation and methylation being able to talk to each other, predilation and then glycosylation. each other, predilation and then glycosylation. All of these modifications are essential to the overall function. A string of amino acids is often just the beginning. And so that is really the goal from this whole lecture. And so I've got these questions, which again, we will start off the bat on Monday with just a nice review questions and lead into our next topic. I hope you've enjoyed this asynchronous session, and I truly hope you enjoy the lovely research day that is happening on Friday. Thank you so much for your time, and I can't wait to see you in the class again. Have a great day.