Practical Lab 4 - Energy and Living Cells: Photosynthesis and Respiration - Fall 2022 PDF
Document Details
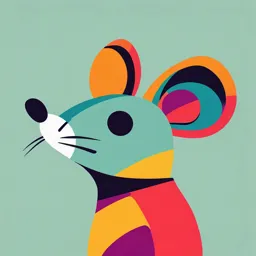
Uploaded by LaudablePanther7166
2022
Tags
Summary
This lab manual outlines practical exercises on photosynthesis and respiration, including methods to measure reaction rates. It covers concepts relevant to biological processes.
Full Transcript
PRACTICAL LAB 4 ENERGY AND LIVING CELLS: PHOTOSYNTHESIS AND RESPIRATION OBJECTIVES: 1. To illustrate the relationship between photosynthesis and cellular respiration. 2. To illustrate two different methods of measuring reaction rates. 3. To investigate the conditions necessary for photos...
PRACTICAL LAB 4 ENERGY AND LIVING CELLS: PHOTOSYNTHESIS AND RESPIRATION OBJECTIVES: 1. To illustrate the relationship between photosynthesis and cellular respiration. 2. To illustrate two different methods of measuring reaction rates. 3. To investigate the conditions necessary for photosynthesis to occur in a preparation of isolated chloroplasts using a colorimetric (or spectrophotometric) method. 4. To measure the rates of respiration in germinating seeds and small animals using a manometric method. ADDITIONAL REFERENCE MATERIAL FOR THIS LAB: Chapter 8 of your textbook, entitled Photosynthesis, is a useful reference to help you with the content in this lab. INTRODUCTION At some point in this course, you’ve looked at the structure of cells in plants and animals. You’ve seen that cellular structure is highly organized. What you have to remember is that in order for the cell to maintain this structure, it must expend considerable energy to repair its membranes, transport molecules, make proteins, and so on. In other words, cell structure is preserved by active energy-requiring processes. In the absence of these processes, a cell would simply become a random collection of disordered molecules and would not be distinguishable from its surroundings. In simple terms, a cell (or organism), in order to maintain its structure, or to divide and grow, needs an energy source and mechanisms to utilize this energy and to parcel it out as it is needed to make the necessary cellular materials. This sounds simple enough and, in fact, the concept is simple: there is a "flow" of energy from the sun which is captured and transformed in some fashion into the variety of structures seen in bacteria, fungi, plants and animals. The actual reactions involved are, predictably, complex. But remember the basic concept the high energy state of the sun is utilized by cells, which produce the low energy wastes of carbon dioxide and water which are, in turn, reactivated into a higher energy state by the sun. And around it goes. Somewhere in between, important intermediates are synthesized that give identifiable cell structure. 1 AUTOTROPHS, HETEROTROPHS AND GLUCOSE In terms of their abilities to utilize energy, all cells can be divided into two groups. Those cells which can utilize the energy of the sun directly (by absorbing light) to produce useful cellular compounds, are called autotrophs (literally "self-feeding"). A cell is a heterotroph (literally "feeding on others") if it relies on the ingestion of foodstuffs which have already been produced by the autotrophs. To use a familiar example, a strawberry bush has autotrophic cells, which can absorb sunlight and the energy is used to produce fruit, the strawberries. We heterotrophs eat the strawberries and can use energy contained in them to fuel our own requirements. What is in strawberries that enables heterotrophs to survive? Mainly glucose, a sugar molecule that contains carbon, hydrogen and oxygen. It seems that light can somehow cause the autotrophic cells of the strawberry bush to put carbon, hydrogen and oxygen into an energetically useful form that we heterotrophs can use! The example of the strawberry bush can best be illustrated diagrammatically (Figure 1), showing the relationship between the two major processes that are at work in the biosphere; namely, photosynthesis, or the light-driven synthesis of glucose and oxygen; and cellular respiration, or the consumption of the glucose and oxygen thus formed. Figure 1: Energy flow in living things. Certain cells in green plants or algae can use light energy to synthesize higher energy organic compounds (like glucose) from CO2 and H2O. All organisms break down organic compounds during cellular respiration to produce useful intermediates. Respiration uses glucose and oxygen and produces CO2 and water, which are, in turn, the essential substrates in photosynthesis. 2 The scheme shown in Figure 1 can also be represented by an equation, which illustrates the basic bioenergetics of autotrophs and heterotrophs: EQUATION 1: (photosynthesis) requires energy from the sun 6CO2 + 6H2O C6H12O6 + 6O2 liberates energy as ATP (respiration) As written, the reaction from left to right represents the overall process of photosynthesis and requires energy. Another way of saying this is that photosynthesis is an endergonic process. This is balanced by the exergonic, or energy-yielding reaction of cellular respiration, from right to left. The overall process is not perfect, however. In either direction, considerable energy is dissipated in unusable forms, usually as heat. For this reason, a constant input of light is necessary to keep the whole biosphere going. The light drives the system UP the energy gradient, to the right, so that it can spontaneously run DOWN the energy gradient, to the left. The amounts of energy involved are enormous -- approximately 686,000 calories are required to synthesize a "mole" of glucose (one mole of glucose is approximately 180 grams), and precisely this same amount of energy is released if the glucose is completely consumed during cellular respiration. CHLOROPLASTS AND MITOCHONDRIA Photosynthesis and respiration occur within the confines of two prominent membrane- bound organelles: photosynthesis occurs in chloroplasts, while respiration occurs in mitochondria. There are illustrations in your text of the main features of these organelles. A brief description of the two organelles follows, and you should understand the location and function of the underlined structures. a) Chloroplast Structure: All green plants contain chloroplasts. The chloroplast is bound on the outside by a double membrane (Figure 2). Within the semi-fluid interior or stroma of the chloroplast is another extensive system of membranes. These membranes form disc-like structures, the thylakoids, which enclose internal spaces; think of the thylakoids as a stack of flattened balloons. A stack of these thylakoids is called a granum and is the major site of the pigment molecules and electron carriers of photosystems I and II; i.e. the light reactions of photosynthesis occur here. The Calvin Cycle takes place within the stroma. Each of the grana is connected by extensions of some of the thylakoid membranes, the lamellae, which randomly extend throughout the stroma. An occasional starch granule is present in some chloroplasts, representing some of the stored products of photosynthesis. 3 Figure 2: Diagram of a chloroplast. b) Mitochondrial Structure: All eukaryotic cells (both plant and animal) contain mitochondria. The mitochondrion is surrounded, like the chloroplast, by a double membrane. The outer membrane is unfolded while the inner membrane is extensively folded inward to form cristae. There is a semi-fluid matrix between the cristae (Figure 3). The intermediates produced by glycolysis (in the cytosol) are found in the matrix while the electron transport chain and ATP synthesizing enzymes are located on the cristae. Figure 3: Diagram of mitochondrion. 4 MEASURING REACTION RATES As you can tell from the preceding descriptions of photosynthesis and cellular respiration, these two vital energy-yielding processes are complex and involve many steps and intermediates. Critical to our understanding of processes such as these is the ability to measure the rate of a reaction, both in an overall reaction as well as in the component reactions (if appropriate). Measuring reaction rates as reliably and simply as possible is a cornerstone of much of biochemistry and cell biology. In general, to measure a reaction rate, the amounts of one of the reactants or products is measured and used as an index of the rate of the process. This principle applies to the determination of reaction rates of either overall net reactions or intermediate, partial reactions. The principles of measuring reaction rates are very simple; select a reactant or product or a specific intermediate and monitor its level or concentration within a reaction vessel. The details of how one might do this monitoring however, can sometimes become complex, depending on the specific reaction being studied as well as the accuracy required of the rate determination. In addition, when reaction rates are being measured it is important to recognize all of the variables that can influence reaction rates and take the necessary steps to ensure that they remain constant or are controlled in some way. Let us consider specifically the process of photosynthesis and some of the different ways of determining its reaction rate. Looking at the overall reaction first, it seems that it should be possible to monitor the concentration of either of the reactants (i.e. H2O or CO2) or either of the products (i.e. O2 or glucose) to determine the reaction rate. In practice, however, because all biological reactions occur within an aqueous environment, it is not possible to follow what is happening to the concentration of water. Any of the other three compounds, however, can be measured to determine the reaction rate of photosynthesis, and protocols for each have been worked out. For example, the concentration of O2 may be followed using an oxygen electrode, which measures the concentration of this gas specifically within a closed reaction chamber. Or, the amount of oxygen within the system may be followed using a technique which measures changes in the total gas volume within a closed system. This is a manometric technique. Manometric techniques are used extensively in science in general, not just in biology, to measure the rates of very diverse reactions. Another way to measure reaction rate is the colorimetric technique. In this procedure, the appearance or disappearance of a colourized material is monitored as a measure of a reaction rate. Sometimes, one of the actual reactants or products may be colourized and a colorimetric technique can be directly applied. In other situations, the interaction of an intermediate or final product with a colourized marker may be used to indirectly measure the concentration of the intermediate or product and hence measure the rate of the reaction. There are a large number of dye molecules which display different colour states depending on prevailing conditions, (such as pH, redox potential, concentration of ligands) and there are numerous procedures for measuring reaction rates that utilize these compounds. 5 In this lab, you’ll use two different techniques (manometric and colorimetric) to examine the reaction rates of cellular respiration and photosynthesis. The manometric technique (that measures a change in gas volume in a closed system) will be used to determine the rate of cellular respiration of either a small animal or of germinating plant seeds (i.e., a non- photosynthetic stage in the plant life cycle). The principle behind the manometric technique is that some type of gas is consumed by the reaction in question and the volume of that gas is measured and converted to a number of molecules of gas consumed. The colorimetric technique will be used to examine the rate of photosynthesis in isolated plant chloroplasts. The colorimetric method uses a “chemical indicator” that interacts with a substance produced in the reaction. This chemical indicator changes colour as more of the substance is produced. The substance being produced by the reaction is not visible but its effect on the chemical indicator is visible. A spectrophotometer will be used in this technique. As you perform the two different procedures, think about: 1) The applicability of each technique to the measurement of reaction rates; and 2) The advantages and limitations of each technique. EXERCISE I: Examination of respiration rates in different organisms using the manometric technique Since we’re studying cellular respiration rates in this experiment, it’s worth noting the following simplified equation for cellular respiration: 6O2 + C6H12O6 6CO2 + 6H2O + ENERGY (ATP) The Scholander respirometer (Figure 4, next page) is the manometer that will be used in this experiment. Note that the respirometer is made up of a pair of glass vials, which act as reaction chambers, plus a machined plastic block. The organisms are placed in one vial (C1) with a small vial of concentrated KOH (potassium hydroxide) [or NaOH (sodium hydroxide)]. The CO2 produced by the organisms during cellular respiration is absorbed by the KOH and a net reduction in gas volume results. The Scholander must be set up in a specific way if it’s to work properly and allow you to collect data. If you look at the one pictured in Figure 4, you’ll see a blue-ish fluid in the middle of the square block. When the system is open to the atmosphere (as it is in Figure 4), the level of the fluid should be equal on both sides of the “V”, because the pressure on both sides of the tube is equal. If you use the tape to seal the ends of the tube with organisms inside the vial, they’ll be consuming oxygen and producing CO2. If you put a chemical (KOH) in the vial with the organisms that absorbs CO2, you can measure the amount of oxygen being used. The blue fluid will move towards the vial containing the organisms because of a pressure difference caused by the consumption of oxygen. You can use the syringe to apply pressure to the fluid to make it equal again. The syringe allows you to measure how much air you had to add back into the system to equalize the fluid. 6 In this experiment, you’ll determine the respiration rate of two different organisms. You’ll work as a group on one of these organisms and you’ll obtain the data for the second organism from another group. (a) (b) Figure 4: Scholander Respirometer. (a) Diagram of the apparatus with parts labelled. (b) Picture of the actual apparatus with chopped mushrooms in the C-1 vial. The red arrows are indicating that the level of the fluid in the V-shaped tube is equal on both sides. Equipment: The various components of the Scholander apparatus include the following: i) A small [1 mL] plastic syringe mounted on the plastic machined block. ii) The machined plastic block mounted on a retort stand. NOTE: there should be blue fluid filling about half of the "V" shaped tube within the block. iii) Two large glass vials. iv) One small vial with filter paper, soaked in concentrated KOH folded inside. v) 2 pieces of Scotch tape. BE SURE TO WATCH THE VIDEO “USING THE SCHOLANDER RESPIROMETER” THAT’S POSTED ON NEXUS 7 Procedures: 1. Before reading any further, have a look at Figure 4 and think about how you would set up the Scholander respirometer to measure respiration in a test organism. Describe, using words and diagrams, how you plan to set up your Scholander respirometer to measure respiration. Assume that you want to measure the respiration rate for about 30 minutes. Be as detailed as is necessary. Your instructor or demonstrator will "approve" your method before you can actually proceed with the experiment. Some useful tips for figuring out your procedure include the following: a) In order for the system to work, it has to be CLOSED to the atmosphere, but to get it ready to work, it has to be OPEN. b) KOH will ABSORB CO2 gas. c) Gas changes will cause the blue fluid in the manometer to be displaced, but you have to QUANTIFY IT in some way. 2. Once your procedure has been approved by the Instructor or demonstrator, as a group of two, choose an organism and measure the rate of respiration for 30 minutes. Record your group’s data in Table 1 on the next page. Your data should go under the “Organism 1” heading. Please be sure to identify what the organism is in the space provided in Table 1. 3. Repeat your experiment at least once. Open the system to the air and obtain a new KOH filter between trials. 4. Do the required calculations. 5. Obtain the data for another organism from another group and record them in Table 1. This data should be recorded under the “Organism 2” heading. Please be sure to identify what the organism is in the space provided in Table 1. 6. Convert the values for oxygen consumption to glucose consumption, as outlined in Equation 3 at the end of this exercise. Data for all the trials should be recorded in Table 1 on the following page. Make sure you understand how to do these calculations, for exam purposes. 8 Data Analysis: Table 1: Data collected using the Scholander respirometer. Organism 1 (______________) Organism 2 (_____________) Time Trial 1 Trial 2 Trial 1 Trial 2 Syringe Reading at 0 min Syringe Reading at 30 min (after adjustment) Volume of Gas Change in 30 min (mL) Weight of Plant or Animal Tissue (g) Table 2 can now be used to calculate the respiration rates of Organism 1 and Organism 2 (from Table 1). To do these calculations, you need to know the relationship between the volume of a gas and the molar quantity of the gas. A simplified form of this relationship is expressed in Equation 2. EQUATION 2: 1.0 mL of gas = 4.0 x 10-5 moles of gas Table 2: Calculation of respiration rates using the data from Table 1. Respiration Rate of Organism 1 Respiration Rate of Organism 2 (__________________________) (_________________________) Trial 1 Trial 2 Trial 1 Trial 2 Total Oxygen This value Volume Change comes directly from Observed in 30 Table 1 minutes (mL/0.5 hr) Oxygen Volume Change in 1 Hour (mL/hr) Oxygen Volume Change Expressed in moles O2/hr (use Equation 2 above) Oxygen Volume Change per gram of plant or animal (moles O2/hr/g) 9 Respiration (or photosynthetic) rates can be expressed in terms of moles of glucose consumed or produced. Since 1 mole of glucose is consumed (or produced) for every 6 moles of O2 consumed (or produced) -- refer to EQUATION 1, the rates in terms of glucose consumption or production are 1/6 as large as the rates in terms of O2 consumption or production. EQUATION 3: Using the data from Table 2, express the respiration rates in moles of glucose. Organism 1 Respiration rate of Organism 1: _________ (moles O2/hr/g) ÷ 6 = _________ moles glucose/hr/g (Name of Organism 1: ______________________ ) Organism 2 Respiration rate of Organism 2: _________ (moles O2/hr/g) ÷ 6 = _________ moles glucose/hr/g (Name of Organism 2: ______________________ ) EXERCISE II: Examination of photosynthesis in isolated chloroplasts using a colorimertic (or spectrophotometric) technique As seen in the diagram below, photosynthesis can be divided into two basic sets of reactions. The Light Reactions (light capturing reactions) and the carbon fixation processes of the Light Independent Reactions (Calvin Cycle). In the light reactions, light is absorbed by chlorophyll molecules and electrons are energized by those photons. Those high energy electrons are then passed on to the carbon fixation processes of the Calvin Cycle. Some of the sunlight energy captured in the light reactions is used to form ATP by a chemiosmosis process, and some of that ATP energy is also used to power the carbon fixation in the Calvin Cycle. Figure 5: The reactions of photosynthesis. 10 Because chloroplasts are large and relatively sturdy organelles, photosynthesis can conveniently be measured in preparations of isolated chloroplasts. Typically, plant cells are gently broken and the chloroplasts are collected into a cold, buffered solution. Such a preparation has been prepared for you to use in this experiment. In this experiment, the rate of photosynthesis will be studied by monitoring the production of the high-energy product of the light reaction, NADPH. We’ll isolate some intact chloroplasts from plant leaves (spinach) and use them to produce NADPH. The blue dye DPIP (2,6-dichlorophenol-indophenol) will be added to the suspension of chloroplasts and will intercept electrons in the electron transport chain of the chloroplast thylakoid membranes. Thus, these electrons rather than being passed to the Calvin Cycle will be passed to DPIP. The oxidized form of DPIP is dark blue, but as DPIP picks up electrons and becomes reduced, it becomes clearer and clearer. In other words, the DPIP blue dye becomes colourless upon accepting electrons from the chain. Thus, decolorization of the dye means that photosynthesis is taking place and that NADPH is being produced. The rate of the light reaction, then, is followed by monitoring the decolorization of the dye using a spectrophotometer. Procedures: 1. Four experimental cuvettes are required for this experiment. The contents of each cuvette are outlined in Table 3 below. It’s important to note the following things about each cuvette: Cuvette 1 is a “blank” and therefore has no indicator dye present. You’ll use this cuvette to calibrate the spectrophotometer. Cuvette 2 (dark control) and Cuvette 3 (unboiled) have viable chloroplasts in them. Cuvette 2 is simply protected from the light (i.e. covered with tin foil). Cuvette 4 (boiled) contains a preparation of chloroplasts that has been boiled (100oC) for 10 minutes, which denatures all proteins present. Table 3: Contents of Cuvettes for Photosynthetic Rate Determination. Cuvette 1 Cuvette 2 Cuvette 3 Cuvette 4 Cuvette contents (Blank) (Dark Control) (Unboiled) (Boiled) Phosphate Buffer 1.0 mL 1.0 mL 1.0 mL 1.0 mL Distilled Water 4.0 mL 3.0 mL 3.0 mL 3.0 mL DPIP 0.0 mL 1.0 mL 1.0 mL 1.0 mL Unboiled Chloroplasts 3 drops 3 drops 3 drops --- Boiled Chloroplasts --- --- --- 3 drops 2. Turn on the spectrophotometer to warm up the instrument. Set the wavelength to 605 nm. 3. Number the cuvettes near the top rim using a grease pencil. Clean the outside of each cuvette using Kimwipes and then avoid handling the cuvettes except by holding them near the top rim to prevent interference from smudges and fingerprints. Cover cuvette 2 with aluminium foil and prepare a cap of the foil for this cuvette as well. This is the dark control for the experiment. 11 4. Using Table 3 as a guide, add the appropriate volumes of phosphate buffer, distilled water and DPIP to each of the four cuvettes. Obtain small samples of boiled and unboiled chloroplasts from the Instructor. Be sure to label these clearly. Keep both samples on ice to prevent deterioration of the chloroplasts. Note that the chloroplast suspension is not added to the cuvettes at this point. It’s important to only add the chloroplasts at the time the first spectrophotometer reading will be made. Figure 6 shows what the tubes should look like at this point in the procedures. Tube 2 without foil Tube 2 with foil Figure 6: Tubes 1-4 after the addition of phosphate buffer, distilled water, and DPIP. Note: the chloroplast suspension has not been added yet. 5. Once the spectrophotometer is warmed up, add 3 drops of the unboiled chloroplast suspension to cuvette 1. Cover the top of the cuvette with Parafilm (a stretchy, waxy material that can be used to seal cuvettes) and invert the whole tube several times to mix the contents. Use cuvette 1 as the spectrophotometric blank to calibrate the spectrophotometer using the specific directions provided with the machine. Retain this cuvette to check the calibration of the machine at the end of the experiment. 6. Place the test-tube rack 12 to 15 cm in front of the lamp seen in Figure 7. Use both the incandescent bulb and the fluorescent bulb and place the large bottle of water, which will act as a heat sink, between the lights and the cuvettes (as seen in Figure 7). Figure 7: Photograph of the experimental setup for this exercise. 12 7. Once the spectrophotometer is calibrated with cuvette 1, add 3 drops of the unboiled chloroplast suspension cuvette 2. Immediately cover and mix cuvette 2. Then remove the foil sleeve and place the cuvette in the spectrophotometer to measure the % transmittance. Record this value as the time “0” reading in Table 4. Rewrap cuvette 2 in foil and place it in the test-tube rack in front of the light. Record additional % transmittance readings at 2, 4, 6, 8 and 10 minutes. The cuvette should be mixed by inversion each time prior to taking the reading. 8. Once cuvette 2 is started, add 3 drops of the unboiled chloroplast suspension to cuvette 3. Cover it with Parafilm and mix it, and then immediately place it in the spectrophotometer and take the time “0” % transmittance reading. Record this value in Table 4. Place cuvette 3 in the test-tube rack and take additional readings at 2, 4, 6, 8 and 10 minutes. The cuvette should be mixed prior to taking each reading. 9. Repeat the procedure with cuvette 4, except add 3 drops of BOILED chloroplast suspension. Obtain and record % transmittance readings (in Table 4) in the same manner as for the other cuvettes. Table 4: % Transmittance readings from the photosynthesis experiment. Sample 0 min 2 min 4 min 6 min 8 min 10 min Cuvette 2 (Dark) Cuvette 3 (Unboiled) Cuvette 4 (Boiled) Analysis of the results: 1. A great way to display information gathered in an experiment is by plotting the data on a graph. By doing this, it presents the results in an easy to read format. For the above experiment, plot the % transmittance data for the three experimental tubes (tubes 2, 3 & 4) in the space provided on the next page. Time (the independent variable) should be plotted on the x-axis and % transmittance (the dependent variable) should go on the y- axis. You should properly label each axis, include a legend, and give your graph a figure number and appropriate title. Remember that graph titles go below the graph. BE SURE TO WATCH THE TIME-LAPSE VIDEO OF THIS EXPERIMENT THAT’S POSTED ON NEXUS 13 2. Use the graph to briefly explain the different rates of decolorization of DPIP observed in the three experimental tubes. 14 EXERCISE III: Fluorescence in a chloroplast suspension As mentioned, chloroplasts are responsible for the process of photosynthesis. Photosynthesis can be defined as the process by which plants, algae, and photosynthetic bacteria (known as cyanobacteria or blue-green algae) use light energy to drive the synthesis of organic compounds. The photosynthetic process involves the removal of CO2 from the atmosphere, which is used to synthesize carbohydrates, and results in the release of O2. The energy to drive the chemical reactions of photosynthesis comes from the sunlight absorbed by the chlorophyll molecules of chloroplasts. Hence, the first step in photosynthesis is the absorption of visible light from the sun by chlorophyll molecules. Without light, photosynthesis cannot take place. Note: Leaves appear green because the chlorophyll molecules of chloroplasts reflect (or transmit) the green light of the light spectrum. On the other hand, they absorb the violet- blue and red-orange light of the spectrum. When a molecule of chlorophyll absorbs a photon of light, an electron moves to the higher orbital where it has more potential energy. When this happens, chlorophyll is said to be in the excited state. However, the electron can’t stay in the higher orbital for a long time and immediately falls back down to the ground state. As the electron falls down, its excess energy is given off as heat and photons of light (fluorescence). See Figure 8 for a diagrammatic explanation of this process. If a solution of isolated chlorophyll is illuminated, it will fluoresce in the red-orange part of the spectrum. Figure 8: Diagram showing the process of electron excitation by a photon of light and its subsequent fall to a lower energy state. 15 Procedures: 1. Observe the demonstration of two chloroplast suspensions in the lab. They were prepared ahead of time and one is in acetone while the other one is in water (with no addition of acetone). To prepare these chloroplast suspensions, spinach leaves were used. 2. Place the two suspensions under the lamp provided and observe the reddish glare emitted only by the acetone suspension. The red glare is known as fluorescence. BE SURE TO WATCH THE VIDEO OF THIS SETUP THAT’S POSTED ON NEXUS Questions to consider 1. Would you expect to see any fluorescence if the acetone solution of chlorophyll was illuminated with green light? 2. Why did you observe fluorescence in the tube with acetone only? EXERCISE IV: Observation of cellular organelles involved in photosynthesis and cellular respiration a) Mitochondria in liver cells (Slide #87). The liver in higher animals is the major organ for the chemical breakdown of nutrients and the subsequent release of energy. Examine the prepared slide of liver (#87). The cells are polygonal in shape, each containing a dark blue nucleus and grey cytoplasm (Figure 9). Within the cytoplasm, there are a large number of mitochondria. These organelles appear as very small blue-black dots under the light microscope. Also notice the brown stained glycogen granules scattered throughout the slide. Excess carbohydrates are stored in the form of glycogen in the liver. The mitochondrion contains a number of enzymes involved in the energy-yielding process of cellular respiration. a) b) Figure 9: Mitochondria in liver cells. a) Very high magnification image of liver cells b) Liver cells at 400x magnification 16 b) Chloroplasts in parenchyma cells of plants. i) Prepare a wet mount by placing a plant leaf on a clean glass slide, adding a drop of water, and then placing a coverslip over it (see Figure 8 in Practical Lab #1). Observe the typical shape of plant cells and note the chloroplasts, which can be seen as small green granules inside the cells. Figure 10 (below) is an example of what you should see in your microscope. It could be useful for you to sketch one of these plant cells (with chloroplasts inside). ii) Observe the demonstration station in the lab showing cytoplasmic streaming, a special process that takes place in plant cells. This process is the circular flow of cytoplasm and chloroplasts within cells. Cytoplasmic streaming speeds the distribution of materials within plant cells. BE SURE TO WATCH THE VIDEO OF THIS PROCESS THAT’S POSTED ON NEXUS Figure 10: High magnification image of plant cells showing chloroplasts. 17