Week 4- Protein Function.pdf
Document Details
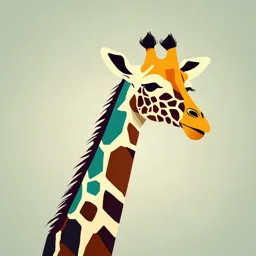
Uploaded by AffirmativeJasper2611
Fenerbahçe Üniversitesi
Tags
Full Transcript
PROTEIN FUNCTION Assist. Prof. Dr. Derya DİLEK KANÇAĞI Room Number: 511 E-mail: [email protected] Office Hour: Wednesday 13.00-15.00 Proteins Function by Interacting Dynamically with Other Molecules Proteins function by interacting dynamically with — binding to — other molecules • Two typ...
PROTEIN FUNCTION Assist. Prof. Dr. Derya DİLEK KANÇAĞI Room Number: 511 E-mail: [email protected] Office Hour: Wednesday 13.00-15.00 Proteins Function by Interacting Dynamically with Other Molecules Proteins function by interacting dynamically with — binding to — other molecules • Two types of interactions: • Protein acting as a reaction catalyst, or enzyme, alters the chemical configuration or composition of a bound molecule • Neither the chemical configuration nor the composition of the bound molecule is changed Central Principles in Protein Function Reversible interactions Principle 1 Principle 2 • The functions of many proteins involve the reversible binding of other molecules. • A ligand binds a protein at a binding site that is complementary to the ligand in size, shape, charge, and hydrophobic or hydrophilic character. • A molecule bound reversibly by a protein is called a ligand. A ligand may be any kind of molecule, including another protein. The transient nature of protein-ligand interactions is critical to life, allowing an organism to respond rapidly and reversibly to changing environmental and metabolic circumstances. • The interaction is specific: the protein can discriminate among the thousands of different molecules in its environment and selectively bind only one or a few types. A given protein may have separate binding sites for several different ligands. These specific molecular interactions are crucial in maintaining the high degree of order in a living system. Central Principles in Protein Function Reversible interactions Principle 3 Principle 4 • Proteins are flexible. • The binding of a protein and a ligand is often coupled to a conformational change in the protein that makes the binding site more complementary to the ligand, permitting tighter binding. • Changes in conformation may be subtle, reflecting molecular vibrations and small movements of amino acid residues throughout the protein. Changes in conformation may also be more dramatic, with major segments of the protein structure moving as much as several nanometers. Specific conformational changes are frequently essential to a protein’s function. • The structural adaptation that occurs between protein and ligand is called induced fit. Central Principles in Protein Function Reversible interactions Principle 5 Principle 6 • In a multisubunit protein, a conformational change in one subunit often affects the conformation of other subunits. • Interactions between ligands and proteins may be regulated. Reversible Binding of a Protein to a Ligand: Oxygen-Binding Proteins Myoglobin and hemoglobin may be the most-studied and best understood proteins. Oxygen Can Bind to a Heme Prosthetic Group Oxygen • Poorly soluble in aqueous solutions • Diffusion through tissues is ineffective over large distances • Transition metals have strong tendency to bind (iron, copper) The evolution of larger, multicellular animals depended on the evolution of proteins that could transport and store oxygen. However, none of the amino acid side chains in proteins are suited for the reversible binding of oxygen Heme Prosthetic Group • Heme = protein-bound prosthetic group • Present in myoglobin and hemoglobin • Consists of a complex organic ring structure, protoporphyrin, with a bound Fe2+ atom Free iron promotes the formation of highly reactive oxygen species such as hydroxyl radicals that can damage DNA and other macromolecules. Iron used in cells is therefore bound in forms that sequester it and/or make it less reactive. Multicellular organisms exploit the properties of metals, most commonly iron, for oxygen transport. The structure of heme and the globins to which it is bound represents an evolutionary adaptation to prevent Fe2+ oxidation. Coordination Bonds of Iron • Six coordination bonds: • Four to nitrogen atoms in the flat porphyrin ring • Two perpendicular to the porphyrin • Two perpendicular coordination bonds: • One is occupied by a side-chain nitrogen • of a highly conserved proximal his residue One is the binding site for molecular oxygen (O2) • Fe2+ binds O2 reversibly • Fe3+ does not bind O2 Some small molecules, such as carbon monoxide (CO) and nitric oxide (NO), coordinate to heme iron with greater affinity than does O2. When a molecule of CO is bound to heme, O2 is excluded, which is why CO is highly toxic to aerobic organisms The coordinated nitrogen atoms (which have an electron-donating character) help prevent conversion of the heme iron to the ferric (Fe3+) state. In heme containing proteins, this reaction is further prevented by sequestering each heme deep within the protein structure. Thus, access to the two open coordination bonds is restricted. Globins Are a Family of Oxygen-Binding Proteins • Globins = widespread protein family • Four types in humans and other mammals: • Highly conserved tertiary structure: eight • Myoglobin = monomeric, facilitates • α-helical segments connected by bends (globin fold) Most function in O2 transport or storage • • • O2 diffusion in muscle tissue Hemoglobin = tetrameric, responsible for O2 transport in the bloodstream Neuroglobin = monomeric, expressed largely in neurons to protect the brain from low O2 or restricted blood supply Cytoglobin = monomeric, regulates levels of nitric oxide, a localized signal for muscle relaxation Myoglobin Has a Single Binding Site for Oxygen • Myoglobin: • 153 residues + one molecule of heme • About 78% of the amino acid residues in • the protein are found in the eight α helices typical of the globin fold, named A through H. Bends named after the α-helical segments they connect Protein-Ligand Interactions Can Be Described Quantitively • A simple equilibrium expression describes the reversible binding of a protein (P) to a ligand (L): • Association constant (ka) = provides a measure of the affinity of the ligand L for the protein • Higher Ka = higher affinity • Equivalent to the ratio of the rates of the forward (association) and the reverse (dissociation) reactions that form the PL complex When the concentration of the ligand is much greater than the concentration of ligand-binding sites, the binding of the ligand by the protein does not appreciably change the concentration of free (unbound) ligand — that is, [L] remains constant. Binding Equilibrium Substituting Ka[L][P] for [PL]: • [L] at which ½ of the available ligand-binding sites are occupied (Y = 0.5) corresponds to 1/Ka x = y/(y + z) describes a hyperbola Representative Kd Values • Dissociation constant (kd) = reciprocal of ka • Equilibrium constant for the release of ligand • Lower Kd = higher affinity • When [L] = Kd, ½ of the ligandbinding sites are occupied Protein Structure Affects How Ligands Bind • Carbon monoxide (CO) binds free heme more than 20,000 times better than does O2 , but it binds only about 40 times better than O2 when the heme is bound in myoglobin. • Differences in the orbital structures of CO and O2 affect binding geometries • The change in relative affinity of CO and O2 for heme when the heme is bound to a globin is mediated by the globin structure. (a) Oxygen binds to heme with the O2 axis at an angle, a binding conformation readily accommodated by myoglobin. (b) Carbon monoxide binds to free heme with the CO axis perpendicular to the plane of the porphyrin ring. Myoglobin’s Distal His Increases Heme’s Affinity for O2 • Hydrogen bond between the imidazole side chain of his E7 and bound O2 electrostatically stabilizes the Fe-O2 polar complex • 20,000-fold stronger binding affinity of free heme for CO compared with O2 declines to ~40-fold This selective enhancement of O2 affinity in globins is physiologically important: it helps prevent poisoning by the CO that is generated in small amounts from metabolism and that is sometimes generated in larger amounts by our industrialage environment. The heme molecule is deeply buried in the folded polypeptide, with limited direct paths for oxygen to move from the surrounding solution to the ligandbinding site. Rapid molecular flexing of the amino acid side chains produces transient cavities in the protein structure, and O2 makes its way in and out by moving through these cavities. They are formed from precursor stem cells called hemocytoblasts. In the maturation process, the stem cell produces daughter cells that form large amounts of hemoglobin and then lose their organelles — nucleus, mitochondria, and endoplasmic reticulum. Erythrocytes are thus incomplete, vestigial cells, unable to reproduce and, in humans, destined to survive for only about 120 days. Hemoglobin Transports Oxygen in Blood • Erythrocytes (red blood cells) transport O2 • Formed from hemocytoblasts (precursor stem cells) • Main function is to carry hemoglobin, which is dissolved in the cytosol at a very high concentration (~34% by weight). • Arterial blood = ~96% saturated with O2 Thus, each 100 mL of blood passing through a tissue releases about onethird of the oxygen it carries • Peripheral blood = ~64% saturated with O2 • Hemoglobin Subunits Are Structurally Similar to Myoglobin • Tetrameric protein with 4 heme groups • Adult hemoglobin has two globin types: two α chains (141 residues each) and two β chains (146 residues each) Interactions among the subunits in Myoglobulin STORAGE Hemoglobin TRANSPORT hemoglobin cause conformational changes that alter the affinity of the protein for oxygen. The Quaternary Structure of Hemoglobin • Strong interactions between unlike subunits • Hydrophobic effect • Hydrogen bonds • Ion pairs (salt bridges) • Α1β1 (and α2β2) interface involves >30 residues • Α1β2 (and α2β1) interface involves 19 residues The hydrophobic effect plays the major role in stabilizing these interfaces, but there are also many hydrogen bonds and a few ion pairs (or salt bridges), • Hemoglobin Undergoes a Structural Change on Binding Oxygen • Two conformations of hemoglobin: • R state = O2 has a higher affinity for hemoglobin • T state = more stable when O2 is absent, predominant conformation of deoxyhemoglobin* *Deoxyhemoglobin: The form of hemoglobin without oxygen, the predominant protein in red blood cells. X-ray analysis has revealed two major conformations of hemoglobin: the R state and the T state. Although oxygen binds to hemoglobin in either state, it has a significantly higher affinity for hemoglobin in the R state. Ion Pairs Stabilize the T State T state is stabilized by a greater number of ion pairs, many of which lie at the α1β2 (and α2β1) interface Conformational Change in Hemoglobin • O2 binding to hemoglobin in the T state triggers a conformational change to the R state • αβ subunit pairs slide past each other and rotate • The pocket between the β subunits narrow • Some ion pairs that stabilize the T state break and some new ones form Changes in Conformation Near Heme • The shift in the position of helix F when heme binds O2 is thought to be one of the adjustments that triggers the T R transition. Hemoglobin Binds Oxygen Cooperatively • Hemoglobin has a hybrid sigmoid binding curve for oxygen. • Hemoglobin must bind oxygen efficiently in the lungs, where the is about 13.3 kPa, and it must release oxygen in the tissues, where the pO2 is about 4 kPa. The first molecule of O2 that interacts with deoxyhemoglobin binds weakly, because it binds to a subunit in the T state. Its binding, however, leads to conformational changes that are communicated to adjacent subunits, making it easier for additional O2 molecules of to bind. In effect, the T › R transition occurs more readily in the second subunit once O2 is bound to the first subunit. The last (fourth) O2 molecule binds to a heme in a subunit that is alreadyin the R state, and hence it binds with much higher affinity than the first molecule. Allosteric Proteins • Allosteric protein (e.g., Hemoglobin) = binding of a ligand to one site affects the binding properties of another site on the same protein • Modulators = ligands that bind to an allosteric protein to induce a conformational change • Homotropic = normal ligand and modulator are identical • Heterotropic = modulator is a molecule other than the normal ligand O2 can be considered as both a ligand and an activating homotropic modulator. There is only one binding site for O2 on each subunit, so the allosteric effects giving rise to cooperativity are mediated by conformational changes transmitted from one subunit to another by subunit-subunit interactions. Hemoglobin Also Transports H+ and CO2 • Hemoglobin carries two end products of cellular respiration: H+ and CO2 to the lungs and kidneys for excretion. • Carbonic anhydrase (abundant in RBCs) catalyzes the hydration of CO2 produced by oxidation of organic fuels in mitochondria to bicarbonate: • Hemoglobin transports about 40% of the total H+ and 15% to 20% of the formed CO2 in the tissues to the lungs and kidneys. • The binding of oxygen by hemoglobin is profoundly influenced by pH and CO2 concentration, so the interconversion of CO2 and bicarbonate is of great importance to the regulation of oxygen binding and release in the blood. The Bohr Effect • The structural effects of H+ and CO2 binding to hemoglobin favor the T state • The binding of H+ and CO2 is inversely related to the binding of O2 • Bohr effect = describes the effect of pH and [CO2] on the binding and release of O2 by hemoglobin • The Effect of pH on O2 Binding to Hemoglobin • When [O2] is high, hemoglobin binds O2 and releases H+ (lungs) • When [O2] is low, hemoglobin releases O2 and binds H+ (peripheral tissues) Both H+ and O2 are bound by hemoglobin, but with inverse affinity HHb+ denotes a protonated form of hemoglobin Hemoglobin Binds CO2 • CO2 binding to hemoglobin is inversely related to binding of O2 • Contributes to the Bohr effect by producing H+ Oxygen Binding to Hemoglobin Is Regulated by 2,3Bisphosphoglycerate • 2,3-bisphosphoglycerate (BPG): • Example of heterotropic allosteric modulation • Binds to a site distant from O2-binding site • Greatly reduces the affinity of hemoglobin for oxygen (inverse relationship) • BPG binds to the cavity between the β subunits in the T state • Cavity is lined with positively charged residues interacting with negatively charged groups of BPG • BPG stabilizes the T state • Unlike O2, only one molecule of BPG is bound to each hemoglobin tetramer. • BPG increases at high altitudes • Hypoxia = lowered oxygenation of peripheral tissues • Causes BPG increases In the absence of BPG, hemoglobin is primarily present in the R state, where it binds O2 efficiently in the lungs but fails to release it in the tissues. Fetal Hemoglobin • Fetus synthesizes α2γ2 hemoglobin • Lower affinity for BPG than normal adult hemoglobin • Higher affinity for O2 than normal adult hemoglobin Sickle Cell Anemia Is a Molecular Disease of Hemoglobin • Sickle cell anemia: • Homozygous condition • Single amino acid substitution (glu6 to val6) β chains produces a hydrophobic patch • Deoxygenated hemoglobin becomes insoluble and forms polymers that aggregate • Normal hemoglobin remains soluble upon deoxygenation Complementary Interactions between Proteins and Ligands: The Immune System and Immunoglobulins Immune Responses • Immune response = coordinated set of interactions among many classes of proteins, molecules, and cell types • Distinguishes molecular “self” from “nonself” and destroys “nonself” • Eliminates viruses, bacteria, and other pathogens and molecules Almost all organisms have an immune system of some type that allows them to respond to challenges presented by environmental pathogens. The Immune Response Includes a Specialized Array of Cells and Proteins • Leukocytes = white blood cells, including macrophages and lymphocytes , all of which develop from undifferentiated stem cells in the bone marrow. • The immune response consists of two complementary systems: • Humoral immune system = directed at bacterial infections and extracellular viruses • Cellular immune system = destroys infected host cells, parasites, and foreign tissues The Humoral Immune Response • Antibodies = immunoglobulins (Ig) = bind bacteria, viruses, or large molecules identified as foreign and target them for destruction • Produced by B lymphocytes or B cells The Cellular Immune Response • T lymphocytes = cytotoxic T cells (TC cells) • Recognition of infected cells or parasites involves T-cell receptors on the surface of TC cells • Helper T cells (TH cells) = produce soluble signaling proteins called cytokines (interleukins) • Interact with macrophages • The TH cells participate only indirectly in the destruction of infected cells and pathogen • Stimulate the selective proliferation of TC and B cells that can bind to a particular antigen (clonal selection) • Memory cells = permit a rapid response to pathogens previously encountered Vaccines • A vaccine to protect against a particular viral infection • Often consists of weakened or killed virus or isolated proteins from a viral or bacterial protein coat • “Teaches” the immune system what the viral particles look like, stimulating the production of memory cells Humans are capable of producing more than 108 different antibodies with distinct binding specificities. Antigens and Haptens • Antigen = molecule or pathogen capable of eliciting an immune response • Can be a virus, a bacterial cell wall, or an individual protein or other macromolecule • Antibodies or T-cell receptors bind to an antigenic determinant or epitope within the antigen • Haptens = small molecules that can elicit an immune response when covalently attached to large proteins • The antibodies produced in response to protein-linked haptens will then bind to the same small molecules in their free form. Antibodies Have Two Identical Antigen-Binding Sites • Immunoglobulin G (IgG) = major class of antibodies • One of the most abundant blood serum proteins • 4 polypeptide chains: 2 heavy chains and 2 light chains • Cleavage with protease papain releases the basal fragment Fc and two Fab branches (each with a single antigen-binding site) • Constant domains contain the immunoglobulin fold structural motif, a well-conserved structural motif in the all-β class of proteins The Structure of Immunoglobulin G The Variable Domain of Immunoglobulin G • Heavy and light chains each have a variable domain • Variable domains associate to create the antigen-binding site • Allows formation of an antigen-antibody complex Classes and Structure of Immunoglobulins • 5 classes in vertebrates: • characterized by heavy chain: • α for IgA • δ for IgD • ε for IgE • γ for IgG • μ for IgM IgM pentamer • IgD and IgE = similar in structure to IgG • IgM = monomeric, membrane-bound form or in a secreted form that is a cross-linked pentamer of this basic structure • IgA = monomer, dimer, or trimer Phagocytosis of Antibody-Bound Viruses by Macrophages • When Fc receptors bind an antibody pathogen complex, macrophages engulf the complex Antibodies Bind Tightly and Specifically to Antigen • Induced fit = conformational changes in the antibody and/or antigen allow the complementary groups to interact fully • Kd values as low as 10–10 M • The Antibody-Antigen Interaction Is the Basis for a Variety of Important Analytical Procedures • Two types of antibody preparations are used: • Polyclonal antibodies • Produced by many different B lymphocytes responding to one antigen • Produced by injecting a protein into an animal • Contain a mixture of antibodies that recognize different parts of the protein • Monoclonal antibodies • Synthesized by a population of identical B cells (a clone) • Homogeneous, all recognizing the same epitope. Western Blots • Immunoblot = western blot assay = uses antibodies to detect a protein nd provides an approximation of its molecular weight Protein Interactions Modulated by Chemical Energy: Actin, Myosin, and Molecular Motors The Major Proteins of Muscle Are Myosin and Actin: Arranged in filaments that undergo transient interactions and slide past each other to bring about contraction Myosin • Myosin (mr 520,000) • 2 heavy chains and 4 light chains • Forms a fibrous, left-handed coiled coil domain (tail) and a large globular domain (head) The contractile force of muscle Thick Filaments • Thick filaments = rodlike structures of aggregated myosin Actin • Actin: • Monomeric G-actin (mr 42,000) associates to form a long polymer called F-actin • Abundant in almost all eukaryotic cells. The contractile force of muscle Together, actin and myosin make up more than 80% of the protein mass of muscle Thin Filaments • Thin filaments = F-actin along with the proteins troponin and tropomyosin • Assemble as successive monomeric actin molecules add to one end • Each monomer binds and hydrolyzes ATP Components of Muscle • Each actin monomer in the thin filament binds to one myosin head group Additional Proteins Organize the Thin and Thick Filaments into Ordered Structures • Muscle fiber = large, single, elongated, multinuclear cell • Each muscle fiber contains ~1,000 myofibrils, each consisting of thick and thin filaments complexed to other proteins and surrounded by A system of flat membranous vesicles called the sarcoplasmic reticulum The Structure of a Sarcomere • Sarcomere = entire contractile unit • A band = stretches the length of the thick filament • I band = contains only thin filaments • Z disk = attachment site for thin filaments • M line = bisects the A band The arrangement of interleaved bundles allows the thick and thin filaments to slide past each other (by a mechanism discussed below), causing a progressive shortening of each sarcomere Myosin Thick Filaments Slide along Actin Thin Filaments • The interaction between actin and myosin, like that between all proteins and ligands, involves weak bonds. • Myosin binds actin tightly when ATP is not bound to myosin • Series of conformational changes due to binding, hydrolysis, and release of ATP and ADP causes muscle contraction Tropomyosin Regulation • Tropomyosin = binds to the thin filament and blocks the myosin-binding sites • Troponin = binds Ca2+ released from the sarcoplasmic reticulum, causes a conformational change, and exposes myosin-binding sites • Subunit C binds Ca2+ • Subunit I prevents binding of the myosin head to actin • Subunit T links the troponin complex to tropomyosin Muscle contraction is stimulated by the release of Ca2+ from the sarcoplasmic reticulum. The Ca2+ binds to the protein troponin, leading to a conformational change in a troponintropomyosin complex that triggers the cycle of actin-myosin interactions