Week 3 - Bio PDF
Document Details
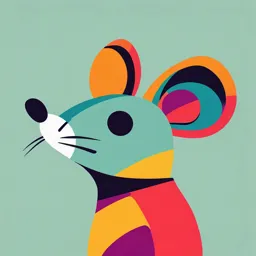
Uploaded by ConscientiousPascal
Tags
Summary
This document describes the action potential diagram phases A, B, C, and D, including the resting membrane potential, depolarization, peak of action potential, repolarization, undershoot, hyperpolarization, and the sodium-potassium pump (and action potential initiation concepts).
Full Transcript
Week 3 -- bio Action Potential Diagram \- \*\*Phases of the Action Potential\*\*: The diagram showed different phases (A, B, C, D) of the action potential. The instructor asked students to describe what happens at each phase, starting with phase A. \#\#\# Phase A: Resting Membrane Potential \...
Week 3 -- bio Action Potential Diagram \- \*\*Phases of the Action Potential\*\*: The diagram showed different phases (A, B, C, D) of the action potential. The instructor asked students to describe what happens at each phase, starting with phase A. \#\#\# Phase A: Resting Membrane Potential \- \*\*Resting Membrane Potential\*\*: - At phase A, the axon is at rest with a membrane potential of about -70 millivolts. - \*\*Key Players\*\*: The sodium-potassium pump is crucial in maintaining this resting state by actively transporting sodium ions (Na⁺) out of the cell and potassium ions (K⁺) into the cell, creating a concentration gradient. - \*\*Ion Distribution\*\*: There is a higher concentration of sodium ions outside the axon and a higher concentration of potassium ions inside the axon. - \*\*Voltage-Gated Channels\*\*: At this stage, the voltage-gated sodium and potassium channels are closed, as the axon has not yet received enough excitation to trigger an action potential. \#\#\# Phase B: Depolarization \- \*\*Voltage-Gated Sodium Channels\*\*: At point B, the voltage-gated sodium channels open when the membrane potential reaches about -55 millivolts. This allows sodium ions (Na⁺) to rush into the axon, making the inside more positive, a process known as \*\*depolarization\*\*. \- \*\*Trigger for Depolarization\*\*: The depolarization at the Axon Hillock (where the action potential starts) reduces the voltage to -55 millivolts, triggering this process. \- \*\*Potassium Channels\*\*: The voltage-gated potassium channels are also activated at this point but are slower to open, so the initial rise in positive charge inside the axon is primarily due to sodium influx. \#\#\# Phase C: Peak of Action Potential \- \*\*Sodium Channels Inactivation\*\*: By the time the action potential reaches its peak (around +40 millivolts), the sodium channels close and become inactivated. \- \*\*Potassium Channels Opening\*\*: The potassium channels, now fully open, allow potassium ions (K⁺) to exit the axon, which starts to repolarize the membrane, bringing the voltage back down toward negative values. \#\#\# Phase D: Repolarization and Undershoot \- \*\*Repolarization\*\*: As potassium exits the axon, the inside of the membrane becomes less positive, eventually returning to a negative value, a process known as \*\*repolarization\*\*. \- \*\*Undershoot (Hyperpolarization)\*\*: After repolarization, the membrane potential briefly becomes more negative than the resting potential, a phase called \*\*undershoot\*\* or \*\*hyperpolarization\*\*. This happens because the potassium channels are slow to close, allowing more potassium to leave the axon than necessary, resulting in a more negative membrane potential. \- \*\*Return to Resting State\*\*: Eventually, the potassium channels close, and the sodium-potassium pump restores the membrane potential to its resting state of -70 millivolts. \#\#\# Undershoot (Hyperpolarization) and Sodium-Potassium Pump \- \*\*Undershoot\*\*: The undershoot (or hyperpolarization) occurs after the action potential, where the membrane potential becomes more negative than the resting potential. This happens because the voltage-gated potassium channels are slow to close, allowing more potassium to leave the axon than necessary. \- \*\*Sodium-Potassium Pump\*\*: The sodium-potassium pump helps re-establish the resting membrane potential after the undershoot. It\'s always active, using ATP as its energy source to maintain the correct ion gradients by pumping sodium out and potassium in. While it contributes to restoring the resting potential, the immediate cause of the undershoot is the delayed closing of the potassium channels. \#\#\# Action Potential Initiation \- \*\*Summation of Inputs\*\*: The action potential begins when the combined inputs from other neurons (or sensory receptors) cause the membrane potential at the Axon Hillock to reach the critical threshold of about -55 millivolts. This process involves the summation of excitatory postsynaptic potentials (EPSPs) and inhibitory postsynaptic potentials (IPSPs). - \*\*EPSP\*\*: An excitatory input makes the membrane potential more positive (depolarization), increasing the likelihood of reaching the threshold. - \*\*IPSP\*\*: An inhibitory input makes the membrane potential more negative (hyperpolarization), decreasing the likelihood of reaching the threshold. \- \*\*Spatial and Temporal Summation\*\*: - \*\*Temporal Summation\*\*: If multiple excitatory inputs occur in rapid succession, they can add together to reach the threshold. - \*\*Spatial Summation\*\*: Inputs from different locations on the dendrites can combine to reach the threshold. \- \*\*Triggering Action Potential\*\*: If the combined input at the Axon Hillock reaches the threshold, an action potential is triggered, leading to the rapid depolarization and subsequent repolarization of the neuron. \#\#\# Voltage-Gated Channels and Action Potential Propagation \- \*\*Mechanism of Voltage-Gated Channels\*\*: The voltage-gated sodium and potassium channels are proteins that physically open and close in response to changes in membrane potential. - \*\*Sodium Channels\*\*: These channels open rapidly in response to depolarization, allowing sodium to rush into the axon. They then inactivate quickly, preventing further sodium entry until the membrane repolarizes. - \*\*Potassium Channels\*\*: These open more slowly, allowing potassium to exit the axon, which repolarizes the membrane and eventually leads to hyperpolarization. \#\#\# Nonlinear Behavior of Neurons \- \*\*Linear Input, Nonlinear Output\*\*: While neurons receive linear inputs (voltage changes), the output response is nonlinear. For example, small increases in depolarization don\'t always lead to proportional changes in response. Only when the critical threshold is reached does a significant response (action potential) occur. This nonlinear behavior is similar to how ANNs process information. \- \*\*Artificial Neural Networks (ANNs)\*\*: ANNs are inspired by the brain but are not exact replicas. They use similar principles of linear input followed by nonlinear activation to process complex tasks like image classification. The example given was recognizing the digit \"8\" despite variations in handwriting. \- \*\*Efficiency in Networks\*\*: The efficiency and power of both biological and artificial neural networks lie in their ability to sum inputs and produce a nonlinear output, enabling complex tasks and learning processes. \#\#\# Communication in Local Neurons \- \*\*Local Neurons and Graded Potentials\*\*: In response to a question about neurons without an axon, the lecturer explains that these neurons communicate via graded potentials. Since these neurons are often small and in close contact with others, the signals don't need to travel long distances, and the communication is typically localized across the soma. \#\#\# \*\*Neuron Classification & Structure\*\* \- \*\*Neurons\*\*: Basic units of the nervous system, classified by the number of appendages (neurites) they have. - \*\*Neurites\*\*: Includes dendrites and axons. - \*\*Unipolar Neurons\*\*: Have one appendage (usually found in invertebrates). - \*\*Bipolar Neurons\*\*: Have two appendages (one dendrite, one axon). - \*\*Multipolar Neurons\*\*: Have multiple appendages (common in vertebrates, especially in the brain and spinal cord). \- \*\*Axon\*\*: Typically, there is one axon per neuron in vertebrates; it transmits electrical signals over long distances. \- \*\*Dendrites\*\*: Receive signals and transmit them to the neuron's cell body (soma). \#\#\# \*\*Action Potentials\*\* \- \*\*Action Potential\*\*: A rapid rise and fall in voltage across the neuronal membrane, used to transmit signals along the axon. - \*\*Propagation\*\*: Action potentials regenerate at various points along the axon, not just one initial burst. - \*\*Myelination\*\*: Myelin sheaths insulate the axon, allowing for faster transmission and less frequent action potentials. \#\#\# \*\*Synaptic Transmission\*\* \- \*\*Chemical Synapses\*\*: The most common type of synapse where neurotransmitters are released to communicate between neurons. - \*\*Neurotransmitters\*\*: Chemicals that transmit signals across a synapse from one neuron to another. - \*\*Key Points\*\*: - Chemical transmission is slower than electrical, but the synaptic gap is very small, so the delay is minimal. - Sherrington's work on reflexes suggested that synapses are involved in slowing down the transmission, indicating chemical communication. \- \*\*Electrical Synapses\*\*: Less common, use gap junctions to allow direct electrical communication between neurons. - \*\*Gap Junctions\*\*: Allow ions and small molecules to pass directly between neurons, enabling faster communication. - \*\*Applications\*\*: Found in time-critical situations, like synchronized breathing or quick flight responses in animals. \#\#\# \*\*Reflexes & Central Pattern Generators\*\* \- \*\*Reflexes\*\*: Simple, automatic responses to stimuli that involve the spinal cord, not the brain. - \*\*Sherrington\'s Experiments\*\*: Demonstrated that complex movements could be generated by the spinal cord alone, without input from the brain. - \*\*Example\*\*: Dogs with severed spinal cords could still walk on a treadmill, indicating local control of movement by the spinal cord. \#\#\# \*\*Chemical vs. Electrical Communication\*\* \- \*\*Sherrington's Conclusion\*\*: Initially believed only electrical signals were fast enough for reflexes, but later evidence showed chemical communication is involved. \- \*\*Loewi's Experiment\*\*: Showed that chemical substances (neurotransmitters) could induce electrical responses in other neurons, confirming the role of chemicals in synaptic transmission. \#\#\# Study Notes: Synaptic Transmission \#\#\#\# Overview of Synaptic Transmission \- \*\*Synapse:\*\* A junction between two neurons where communication occurs. Neurons are not physically connected but are separated by a tiny gap called the synaptic cleft (\~40 nanometers wide). \- \*\*Presynaptic Neuron:\*\* The neuron that sends the signal. \- \*\*Postsynaptic Neuron:\*\* The neuron that receives the signal. \#\#\#\# Process of Synaptic Transmission 1\. \*\*Action Potential:\*\* - An electrical signal (action potential) travels down the axon of the presynaptic neuron. - Upon reaching the axon terminal, the action potential triggers the opening of voltage-gated calcium channels. 2\. \*\*Calcium Influx:\*\* - Calcium ions (Ca²⁺) enter the presynaptic neuron due to the electrical signal. - Calcium plays a key role in the release of neurotransmitters. 3\. \*\*Vesicle Fusion:\*\* - Vesicles containing neurotransmitters are present in the presynaptic neuron. - Calcium ions cause these vesicles to move toward and fuse with the presynaptic membrane. 4\. \*\*Neurotransmitter Release (Exocytosis):\*\* - The fusion of vesicles with the membrane releases neurotransmitters into the synaptic cleft. - This release process is known as exocytosis. 5\. \*\*Binding to Receptors:\*\* - Neurotransmitters diffuse across the synaptic cleft and bind to specific receptors on the postsynaptic neuron. - The binding of neurotransmitters can either depolarize (excite) or hyperpolarize (inhibit) the postsynaptic neuron. 6\. \*\*Clearing the Synapse:\*\* - \*\*Diffusion:\*\* Some neurotransmitters simply drift away. - \*\*Reuptake:\*\* Neurotransmitters are taken back into the presynaptic neuron to be recycled. - \*\*Enzymatic Breakdown:\*\* Enzymes in the synapse can break down neurotransmitters. \#\#\#\# Types of Receptors 1\. \*\*Ionotropic Receptors:\*\* - Directly linked to ion channels. - Neurotransmitter binding causes the ion channels to open or close. - Fast and localized response. - Example: Acetylcholine opens sodium channels, leading to depolarization. 2\. \*\*Metabotropic Receptors:\*\* - Not directly linked to ion channels. - Neurotransmitter binding activates a G-protein, which then initiates a cascade of intracellular events. - Slower but longer-lasting effects compared to ionotropic receptors. - \*\*G-Protein Coupled Receptors:\*\* - When a neurotransmitter binds, it activates the G-protein. - G-protein exchanges GDP for GTP, detaches, and affects various cellular processes. \#\#\#\# Effects of Drugs on Synaptic Transmission \- \*\*Cocaine:\*\* - Blocks the reuptake of dopamine, causing prolonged presence of dopamine in the synaptic cleft. - Leads to amplified effects of dopamine but results in withdrawal symptoms due to depleted dopamine levels after drug effects wear off. \- \*\*Ritalin:\*\* - Similar to cocaine but has a milder effect on dopamine reuptake, leading to less severe withdrawal symptoms. \- \*\*Nicotine:\*\* - Binds to receptors on the postsynaptic neuron, affecting synaptic transmission. Key Terms \- \*\*Synaptic Cleft:\*\* The gap between the presynaptic and postsynaptic neurons. \- \*\*Vesicle:\*\* A small sac that stores neurotransmitters. \- \*\*Exocytosis:\*\* The process of neurotransmitter release. \- \*\*Reuptake:\*\* The process of neurotransmitter reabsorption by the presynaptic neuron. \- \*\*Ionotropic Receptor:\*\* A receptor that directly controls an ion channel. \- \*\*Metabotropic Receptor:\*\* A receptor that activates a G-protein, leading to a series of intracellular reactions. \- \*\*G-Protein:\*\* A protein activated by a metabotropic receptor that initiates various cellular responses. \- \*\*GDP/GTP:\*\* Guanosine diphosphate/triphosphate; energy sources used in G-protein activation. \#\#\# Lecture Notes: Neurotransmission and Receptor Types \#\#\#\# 1. \*\*Overview of Neurotransmission\*\* - \*\*Intra-neuron Communication:\*\* Information is transmitted electrically within a neuron. - \*\*Inter-neuron Communication:\*\* Information is transmitted chemically between neurons via neurotransmitters. \#\#\#\# 2. \*\*Neurotransmitters and Synaptic Transmission\*\* - \*\*Release Process:\*\* - Neurotransmitters are released from the presynaptic neuron into the synaptic cleft. - They diffuse across the cleft and bind to receptors on the postsynaptic neuron. - \*\*Receptor Types:\*\* - \*\*Ionotropic Receptors:\*\* - Directly open ion channels upon neurotransmitter binding. - Example: Acetylcholine binding allows sodium (Na+) to enter the neuron, leading to depolarization. - \*\*Metabotropic Receptors:\*\* - Indirectly influence the neuron via G proteins and secondary messengers. - Can activate or inhibit various intracellular processes depending on the specific G protein involved. \#\#\#\# 3. \*\*G Proteins and Their Effects\*\* - \*\*Versatile Effects:\*\* - G proteins can communicate information to the cell\'s nucleus, influence gene transcription, or affect intracellular calcium levels. - \*\*Excitatory vs. Inhibitory G Proteins:\*\* - Excitatory G proteins can increase cellular metabolism or trigger other activating processes. - Inhibitory G proteins can deactivate previously activated proteins or prevent depolarization. \#\#\#\# 4. \*\*Complexity of Neurotransmission\*\* - \*\*Multiple Neurotransmitters:\*\* - Different neurotransmitters (e.g., Neurotransmitter A, B, C) can be released simultaneously and have distinct effects. - \*\*Interaction of Receptors:\*\* - One neurotransmitter might activate an ionotropic receptor, while another activates or inhibits a metabotropic receptor. - The overall effect depends on the balance between these processes. \#\#\#\# 5. \*\*Pre-synaptic Receptors (Auto-receptors)\*\* - \*\*Feedback Mechanism:\*\* - Auto-receptors on the presynaptic neuron detect neurotransmitter levels and can stop further release if necessary. - \*\*Example:\*\* - Marijuana can activate auto-receptors, reducing neurotransmitter release, leading to decreased neural activation and reduced anxiety. \#\#\#\# 6. \*\*Drugs and Receptor Interaction\*\* - \*\*Agonists:\*\* - Drugs that mimic neurotransmitters and activate receptors. - Example: Agonist drugs that bind to receptors and initiate neurotransmitter-like effects. - \*\*Antagonists:\*\* - Drugs that block receptors and prevent neurotransmitter effects. - Example: Antagonist drugs that fill receptors, inhibiting neurotransmitter binding and effects. \#\#\#\# 7. \*\*Key Takeaways for Revision\*\* - \*\*Primary Concepts:\*\* - Chemical transmission between neurons is mediated by neurotransmitters. - Receptors can be either ionotropic or metabotropic, with different downstream effects. - \*\*Revision Suggestions:\*\* - Draw out the entire process of neurotransmission, including the key players and how they interact. - Consider the effects of disabling specific parts of the process to understand their roles. - \*\*Additional Resources:\*\* - Videos and resources for further understanding of neurotransmitter-receptor interactions and the effects of drugs.